INTRODUCTION
The Pacific oyster, Crassostrea gigas, is a commercially valuable species with significant production volumes worldwide. In the mid-1990s, Pacific oyster aquaculture substantially improved, leading to increased production (McAfee & Connell, 2021). Most oyster aquaculture countries, such as China, Japan, and Korea, have developed spawning sites to produce better quality of seed. This means that the development of genetically improved broodstock can provide cost-effective seed for aquaculture and enhance profitability (Chen et al., 2022). In this process, to prevent the loss of genetic diversity in spawning sites, methods such as mass use of broodstock, balancing the sex ratio, artificial seedling, and introducing wild individuals from distant locations were applied to increase effective seed production (In et al., 2016; Xu et al., 2019; Chen et al., 2022). Nevertheless, characteristic of spawning site was not clearly apparent because growth is highly influenced by genetics, food, and environmental conditions (Baghurst & Mitchell, 2002; Dridi et al., 2007; Çelik et al., 2015).
In previous studies, the Insulin-like growth factors (IGFs) system has been identified as a major factor in the neuroendocrine regulation of various physiological effects, such as growth and gonadal maturation in the Pacific oyster (Choi et al., 2018; Moon & Choi, 2019; Park & Choi, 2019; Park & Choi, 2021a,b). Moreover, the concentration of neuroendocrine proteins, including IGF, affects body growth in marine organisms (Andrews et al., 2011). The IGF system in the Pacific oyster has three elements: the molluscan insulin-related peptide (MIP), known as the ligand; the IGF binding protein complex acid labile subunit (IGFBP-ALS), which aids in ligand migration and prolongs its half-life; and the receptor of the ligand, called the C. gigas insulin receptor-related receptor (CIR). However, the specific functions and mechanisms of these genes in growth and maturation have not been clearly identified (Hamano et al., 2005; Gricourt et al., 2006). Moreover, the regulation of IGF system is highly affected by nutrients and, thus, often expression is dependent on the availability of food in sites where animals are being grown (Estívariz & Ziegler, 1997; Diederich, 2006). In Korea, numerous studies have shown clear differences in the formation and biomass of phytoplankton communities in adjacent regions, regardless of the sea site, and similar patterns are observed overseas (Kang et al., 2006; Kim et al., 2020; Lin et al., 2020). In particular, Korea is known for the different tastes and sizes of oysters due to variations in farming methods on the west and south coasts. Additionally, habitat characteristics such as location and environmental factors affect the body composition, taste components, and size of oysters (Kitaoka et al., 2016; Haisheng et al., 2019).
Oyster body composition, including fatty acids, amino acids, and glycogen, shows clear differences depending on the season, habitat, and harvest time (Hwang et al., 2016; Cho et al., 2023). Particularly, body composition and condition index (CI) influence taste, while different species within the Crassostrea genus exhibit variations in taste (Murata et al., 2020; Liu et al., 2021). Although no specific food source can be identified due to oysters’ nonselective feeding behavior, these factors are likely influenced by water temperature and available food sources (Flores-Vergara et al., 2004; Tran et al., 2022).
Therefore, in this study, we aimed to confirm the differences in somatic growth, growth-related gene expression of IGF system members, and difference in taste of Pacific oysters collected at three different specific sites within two specific-growing sites. The spawning and growing sites selected in this study have been used for seedling and cultivating natural spat since 1969, when mass production through the aquaculture method was established (Lovatelli, 1988).
MATERIALS AND METHODS
In July 2022, Pacific oyster spat, which are larvae that permanently attach to a surface on shell strings, were collected from Busan Gadeok-do (BS; 35°04’32.7”N, 128°50’07.0”E), Tongyeong Suwol (TY; 34°52’58.3”N, 128°18’10.7”E), and Namhae Galhwa (NH; 34°54’24.8”N, 127°51’12.3”E) located at the southern coast of Korea. They were hardened at their respective collection sites; the BS and NH groups were hardened at their respective collection sites, while the TY group was hardened in Geoje until June 2023. After the hardening period, the oysters were transferred to separate growing sites in Tongyeong Yeongun site (TS; 34°47’28.2”N, 128°25’46.3”E) and Geoje Eogu site (GS; 34°49’07.1”N, 128°30’21.4”E) located at the southern coast of Korea, where they were grown until December 2023 (Fig. 1).
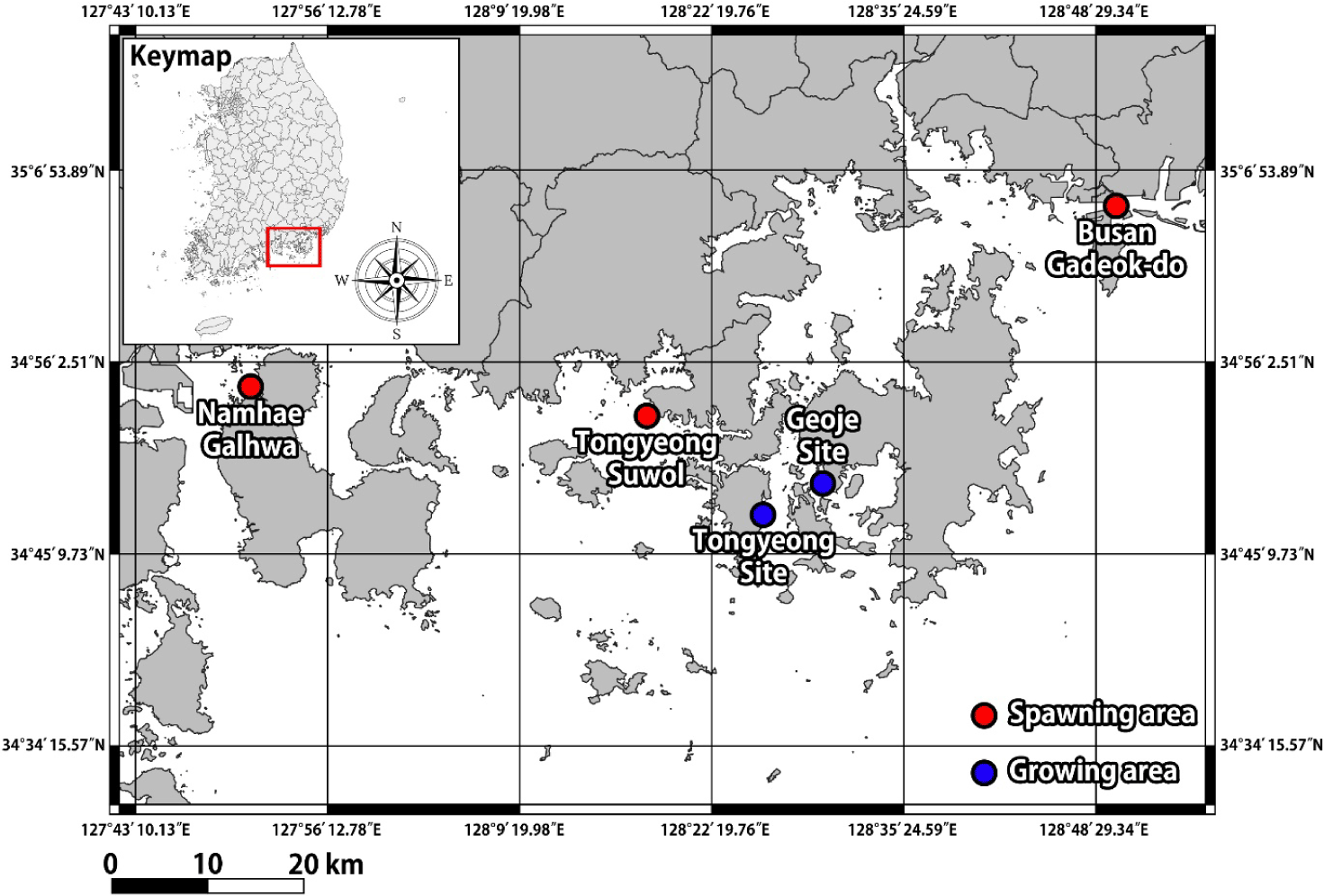
Thirty (30) Pacific oyster samples were collected monthly in each of the growing site. Body parameters were measured using vernier calipers for shell length (SL), shell height (SH), and shell width (SW). Total weight (TW) and soft tissue weight (STW) were measured using an electronic balance to calculate the CI and tissue weight rate (TWR). The calculation followed the formulas of Choi & Chang (2003) and Park & Choi (2021a). The adductor muscle was dissected and immediately frozen in liquid nitrogen, then stored at –80°C until analysis.
To analyze the CIR, IGFBP_ALS, and MIP mRNA expression levels in the adductor muscle of Pacific oysters, the adductor muscles (n=3 per month) were cut into 0.5 cm2 pieces, placed in a 1.5 mL microtube and subjected to total RNA extraction using RNAiso PLUS (600 μL; Takara, Shiga, Japan). Then, samples were homogenized in chloroform and precipitated using isopropanol. The isolated RNA pellets were washed with ethanol. Quantitative and qualitative analysis of the total RNA was conducted using a UV/VIS Nano spectrophotometer (MicroDigital, Seongnam, Korea) after dissolution in DNase/RNase-free water. The total RNA was synthesized into complementary DNA (cDNA) using the PrimeScript™ 1st strand cDNA Synthesis Kit (Takara) following the manufacturer’s instructions. The synthesized cDNA was stored at –20°C until use in RT-PCR. PCR products were obtained using 10 ng/μL of cDNA and EmeraldAMP GT PCR Master Mix (Takara) following the manufacturer’s instructions. The primers used in the analysis were designed based on the nucleotide sequences suggested by Moon & Choi (2019) (Table 1). Gene expression for each target gene (CIR, IGFBP_ALS, and MIP) and the housekeeping gene (Elongation factor I alpha, EF1α) was analyzed using an Azure Biosystems C300 analyzer (Azure Biosystems, Dublin, CA, USA), and the relative quantities were determined with GeneTools software (Syngene, Cambridge, UK).
To analyze the taste, three oysters, one from each spawning per growing site in December 2023, were randomly selected and grouped according to their growing sites. The soft tissue was weighed, and 1 mL of purified water was added per 1 g of tissue. It was then homogenized in an ice-cold bath until completely crushed. The homogenized tissues were subsequently centrifuged to collect the supernatant. After that, a sample solution was made by adding 1 mL of the supernatant to 99 mL of purified water in a standard 100 mL-capacity beaker. Consequently, the electronic tongue (e-tongue, ASTREE II, Alpha MOS, Toulouse, France) was subjected to pre-analysis calibration, conditioning, and diagnostics to reach a constant potential for each of the seven potentiometric sensors (AHS, PKS, CTS, NMS, CPS, ANS, and SCS) and the Ag/AgCl reference electrodes before proper analysis. AHS, CTS, NMS, ANS, and SCS correspond to sourness, saltiness, umami, sweetness, and bitterness, respectively, while the remaining sensors detect other complex tastes. Sample solutions were analyzed according to a sequence designed to measure three times. The resulting data were subjected to multivariate analysis to assess variability among different growing sites. Sensor scores were linearly transformed into a two-dimensional principal component analysis (PCA) for easier observation and interpretation of the data. The taste profiles were further represented on a radar chart with scales from 0 to 12, indicating increasing relative intensity.
The IBM SPSS software version 22 (IBM, Chicago, NY, USA) was utilized for statistical analysis. The statistical significance of somatic growth and growth-related gene expression in each growing sites for each month was determined at the 95% confidence level using one-way analysis of variance (ANOVA), following the verification of data normality (Shapiro-Wilk test) and homogeneity of variance (Levene’s test). Results are reported as mean±SEM.
RESULTS
The TW and STW showed monthly growth, wherein TS showed significantly higher growth than the GS (p<0.05). In particular, among the spawning sites, the NH group showed relatively lower growth than the other groups (Fig. 2A and B). In terms of CI and TWR, the values of the TS were significantly higher than those of the GS in most months (p<0.05) (Fig. 2C and D).
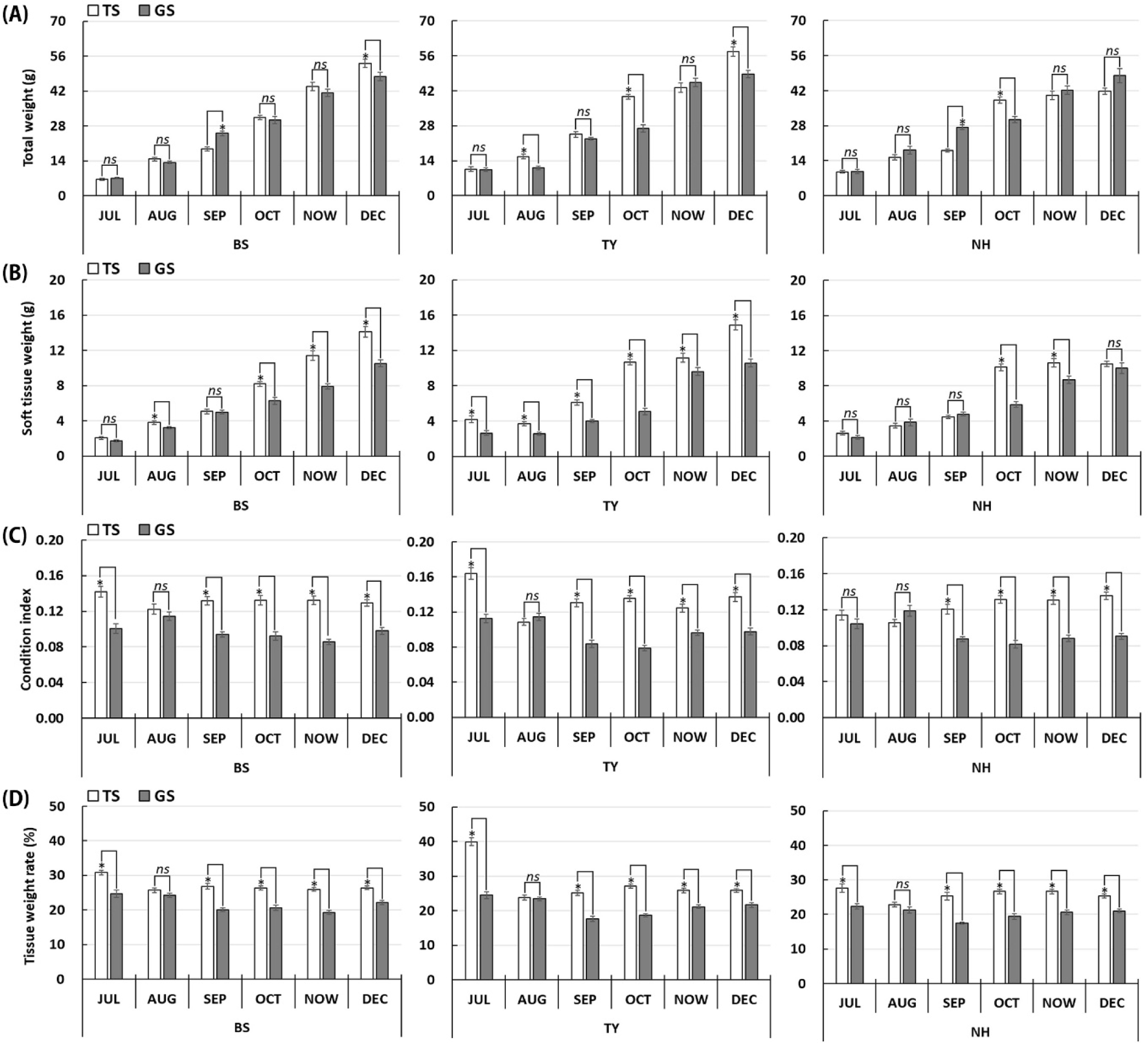
The mRNA expression of MIP and IGFBP_ALS was highest in July and August, with no significant difference between the growing sites (p>0.05). Following this peak, a similar decreasing trend was observed across all sites, with no significant differences between the spawning sites (Fig. 3A and B). Unlike MIP and IGFBP_ALS, the expression of CIR, IGFs receptor, did not exhibit any distinctive monthly trend (Fig. 3C). However, overall IGF mRNA expression was higher in the TS compared to the GS.
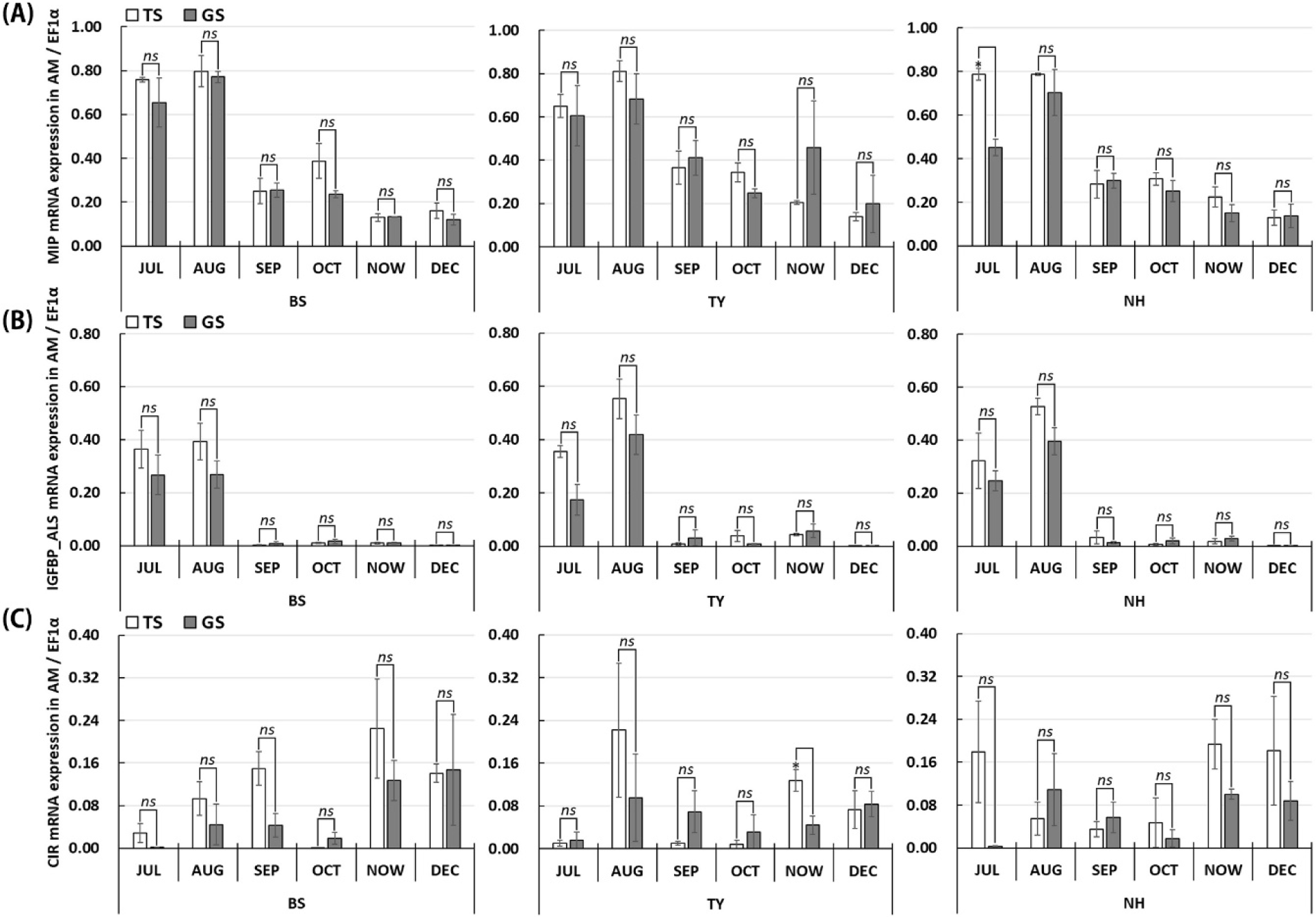
The taste sensory profile of Pacific oysters grown in different sites is shown as a result of multivariate PCA and a taste screening matrix. In the 2-dimensional PCA, PC1 accounts for 68.116% and PC2 for 20.974%, totaling 89.09% variability. The high percentage of variance scores indicates an increased representation of sensor scores within the total set of scores generated during the analysis. The PCA plot revealed overlapping sensor scores, resulting in a negative discrimination index value (–0.09). This negative discrimination index suggests that the overall taste characteristics are similar. However, given the very low discrimination index figures, they likely indicate subtle differences in taste (Fig. 4A). In terms of the taste screening matrix, oyster that GS had a one-score higher umami intensity, and the intensified the saltiness, sweetness, bitterness and sourness flavor of oyster (Fig. 4B).
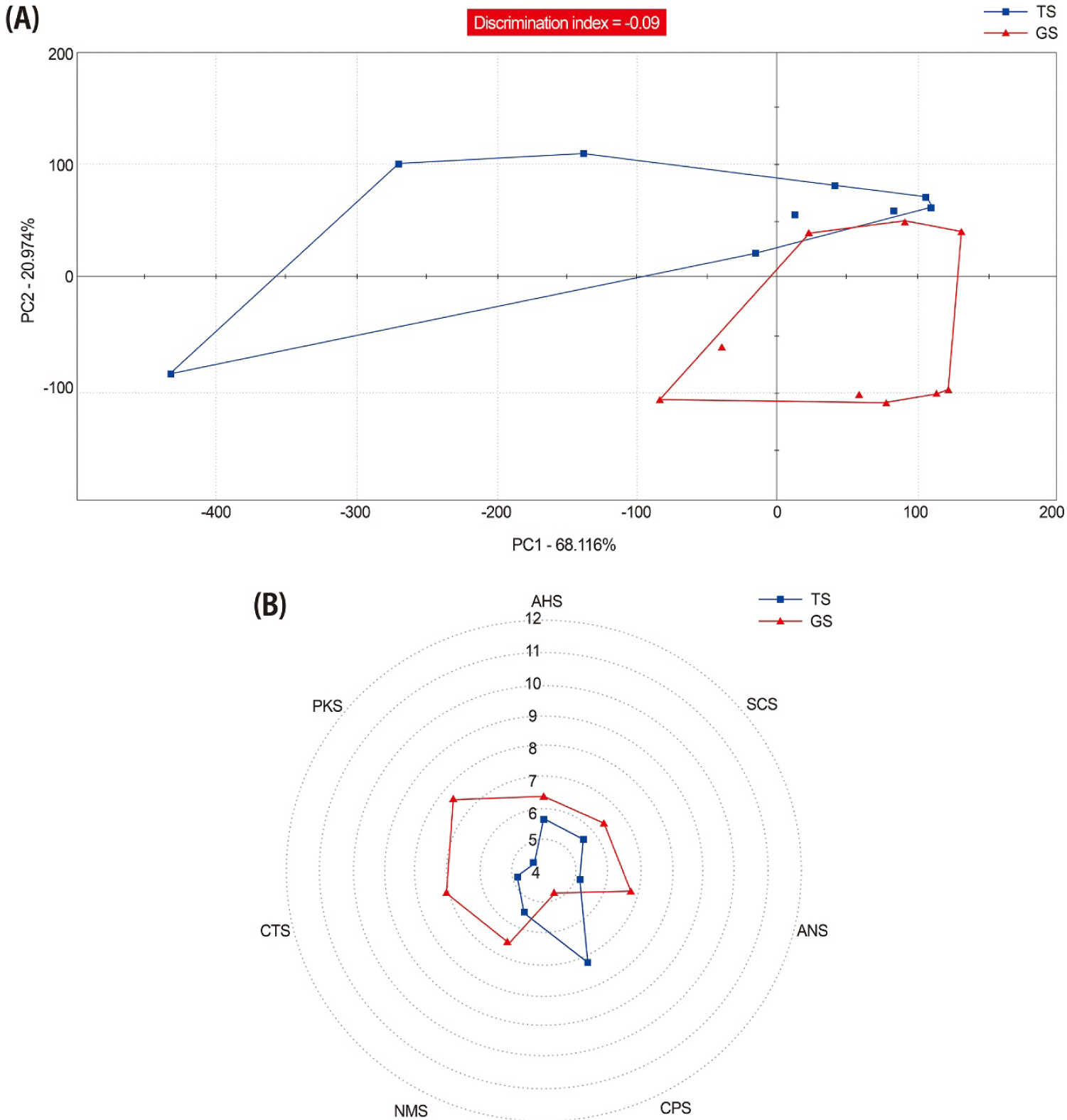
DISCUSSION
Natural seedling is primarily used to obtain spat for oysters in Korea’s aquaculture industry, while artificial seedling is also partially employed to produce oysters of higher quality such as single oyster. Consequently, oyster spawning grounds have been established to ensure continuous and stable oyster farming. Nevertheless, the relationship between location where the oyster grows and its influence on underlying physiological process of growth and taste profile were not fully elucidated.
In the current study, oysters reared in TS generally showed higher growth rates than those that were grown in GS and, thus, oyster growth exhibited regional or location-dependent characteristics. Growth of oysters are known to be influenced by environmental factors such as temperature, phytoplankton abundance including chlorophyll concentration and particulate organic matter (Toro et al., 1999). In the monitoring of the fishery environment near the TS (34°47’19.0”N, 128°25’39.0”E) and GS (34°49’21.0”N, 128°29’29.0”E) it was showed that no major difference was obtained on temperature, salinity, pH and dissolved oxygen level between TS and GS during the sampling period (data not shown). However, chlorophyll concentration in the surface layer near the TS (4.78±0.26 µg/L) was 1.34 µg/L higher than that in near the GS (3.43±1.69 µg/L) (Ministry of Oceans and Fisheries, 2024). With regards to that, the concentration of dietary sources had effect on differences in oyster growth, and a high diversity and biomass of phytoplankton significantly increased the growth of farmed oysters in diatom-dominated sea areas (Toro et al., 1999; Rico-Villa et al., 2009). The concentration of chlorophyll on the southern coast of Korea is about twice as high as on the east and west coasts, and diatoms, which are a food source for oysters, tend to dominate (Oh & Suh, 2006; Jang et al., 2013). However, since the concentration of chlorophyll varies greatly depending on the season and coastal sites that are heavily affected by land runoff, even if they are located adjacent to each other (Kim et al., 2023). Therefore, continuous research is needed to determine whether the above chlorophyll concentration results are suitable for oyster growth.
In the results of mRNA expression in the adductor muscle of Pacific oysters, the expression of MIP and IGFBP_ALS decreased after peaking in July and August. This finding aligns with previous studies, which showed that MIP and IGFBP_ALS exhibited seasonal expression changes, with both showing similar expression patterns and a decreasing trend starting in September (Moon & Choi, 2019; Park & Choi, 2022). Moreover, relatively higher expression of MIP and IGFBP_ALS in TS-grown compared to GS-grown oysters imply that nutritional status had a significant effect on the expression of the IGF members, thereby promoting faster somatic growth. In contrast, CIR mRNA did not display growth expression patterns in this study. This suggests that the IGF system through the CIR do not significantly affect somatic growth of oysters, instead, showed regulating effect on gonadal maturation, as shown by previous studies (Gricourt et al., 2006; Moon & Choi, 2019).
IGF-1 and IGF-2 stimulate the growth and development of vertebrates. Liver-derived IGF-1 mediates the growth-promoting effects of GH from the hypothalamus after hatching, whereas IGF-2 stimulates embryogenic growth and is not regulated by GH (Pierce et al., 2011). However, the clear function of IGFs in shellfish has not yet been identified, and the factors involved are also unclear. The expression of the two IGFs was not related to each other, and their expression trends were also unclear (data not shown). Nevertheless, IGFs protein expression in TS, which showed faster growth, was relatively high, consistent with growth outcomes and IGFs mRNA expression. In previous studies, larger individuals had higher expression of IGF-1 protein and its pathways. These results were similar for mRNA expression, showing similar trends in both previous studies and this study (Choi et al., 2018; Kim & Choi, 2019).
The E-tongue imitate how the human tongue tastes the five essential taste senses and is currently used to evaluate the taste characteristic of food products. E-tongue analysis lead up to for a rapid, impartial, and accurate assessment of the taste attributes of food (Tan & Xu, 2020; Marx et al., 2021). Oysters from the GS, which grew more slowly than those from the TS, showed a higher umami taste rating in the taste analysis results. Additionally, oysters nutritional content such as protein, fat, ash, glycogen, and taurine vary considerably depending on the oyster's growing area (Lin et al., 2019). The artificial supply of certain microalgae (Chlorella vulgaris and Pavlova viridis) led to changes in the fatty acid composition of Pacific oyster and an increase in umami values (Wang et al., 2022). Based on sensory evaluation, Pacific oysters with higher glycogen content have a more sweet or rich taste (Murata et al., 2020). This simply cannot specify that glycogen has a sweet and rich taste, or that glutamic and aspartic acids have a umami taste. Since flavors such as flavor-enhancing nucleotides, betaine, volatile compounds, and free amino acids affect various attributes, continuous research is needed on the total amounts of related ionic components and their combinations, in addition to e-tongue analysis (Fuke & Konosu, 1991).
IGF system is known as growth regulators wherein high expression indicates fast growth and low expression indicates otherwise. Further, its regulation is highly governed by exogenous factors including diet composition, temperature, among others, which could vary by location where the oysters are being reared. Oysters grown in two separate locations-Konagai and Hiroshima in the western coast of Japan-showed variable growth wherein higher body weight and soft weight were obtained in the latter (Kitaoka, et al., 2016). The researchers further analyzed the taste components, and some sweet taste-associated free amino acid composition (serine, alanine) were significantly higher in Konagai compared to Hiroshima. Similarly, Konagai-grown oysters have higher levels of adenosine monophosphate (AMP), an important flavor compound found in marine animals, and show higher umami values in taste sensory tests. This earlier work showed that smaller individuals (relatively lower IGF expression) could have better taste profile than large (relatively higher IGF expression) oysters. The result of the current study supports with above-mentioned study wherein GS-grown oysters, albeit had relatively low expression of IGF system and subsequent growth compared to TS-grown oysters, exhibited better taste sensory profile. However, when the large individual, the glycogen level is high, and it is different from previous studies where the AMP level was shown regardless of the size of the individuals (Hong et al., 2002; Murata et al., 2020). Although the flavor components of oysters have been identified in various research, additional research is needed because the content of taste components in oysters varies significantly depending on several factors, such as the individual oyster, the specific site where they live, and the timing of their collection.
In conclusion, this study confirmed that the endocrine activity of IGFs and growth rates were higher in the TS, indicating a significant difference in growth based on the Pacific oysters’ growing site. Meanwhile, taste sensory evaluation showed that oysters from the GS had a greater variety of tastes, confirming that taste differences are also influenced by the growing site. The findings of the present study imply that different environmental condition in growing site can be a good indicator of production success and consumer taste preference, which can be applied as strategic plan by oyster farmers in their culture operation. Nevertheless, additional location-specific environmental data, such as the phytoplankton profile (including chlorophyll concentration) and recent changes in seasonal water quality parameters, continually need to be explored in regional investigations. These information can serve as key criteria for effective decision-making on what growing sites can sufficiently support growth and produce palatable oysters. As the taste of oysters can vary depending on the environmental conditions of the growing site, which are likely to change indefinitely, determining which region is superior without further investigation seems difficult to address.