INTRODUCTION
The Pacific oyster, Crasssostrea gigas (Magallana gigas), is one of the most important aquaculture species worldwide in terms of aquaculture production and price (Yue et al., 2022). Oysters are affected by various factors such as water temperature, salinity, and feeding conditions (Dridi et al., 2007). Currently, oyster aquaculture production is stagnant due to changes in the marine environment, such as rising water temperatures and decreasing prey (Park et al., 2018). As a result of this influence, changes in physiological characteristics such as oyster growth and condition index (CI) are occurring. The CI is a term for evaluating the edible parts [soft tissue weight (STW)] of shellfish and is known to be an important factor in aquaculture production and marketability (Shim et al., 2021). The CI of C. gigas has been studied in various regions, and the pattern varies by region (Linehan et al., 1999; Chávez-Villalba et al., 2007; Li et al., 2009). Nevertheless, research results on long-term changes in CI and characteristics by sea area are lacking (Shim et al., 2021). CI is closely related to gonadal development in shellfish (Arellano-Martínez & Ceballos-Vázquez, 2001). The stages of gonadal development of oysters can be divided into early development, late development, maturity, spawning, and degenerative stages based on histological characteristics such as the shape and size of gametes (Min et al., 2004). Histological methods classify the reproductive cycle of oysters according to the characteristics observed at various stages of gonadal development. Since the seasonal reproductive cycle of shellfish shows different characteristics influenced by regional distribution, it is important to understand the unique spawning season for each population (Kim & Lee, 2022). Presently due to the rise in coastal water temperature due to climate change, the reproductive cycle changes, and the spawning period gradually changes (Kim et al., 2009). In the face of ever-changing environmental conditions, knowledge of the reproductive cycle and growth parameters is important for the proper management of aquaculture (Jaramillo et al., 2022).
In general, oysters are known to consume stored energy from spring to summer along with gonadal development and store energy in autumn and winter. Many studies have been conducted on seasonal biochemical composition changes in oysters, but studies according to the reproductive cycle throughout the year are insufficient (Mann, 1979; Shin et al., 2002; Ngo et al., 2006; Liu et al., 2010; Pogoda et al., 2013).
Therefore, in this study, histological analysis was performed to confirm the year-round growth change and gonadal development process of oysters cultivated in Gadeok-do, Gyeongsangnam-do, and the year-round biochemical composition change of the soft body part was investigated.
MATERIALS AND METHODS
For this experiment, oysters being cultivated in Gadeok-do, Gyeongsangnam-do (35°04′19.0″N 128°50′28.6″E) were used, and 40 oysters were randomly collected every month from March 2021 to February 2022. The water temperature, salinity, and pH of the nursery were measured at monthly collections (Fig. 1).
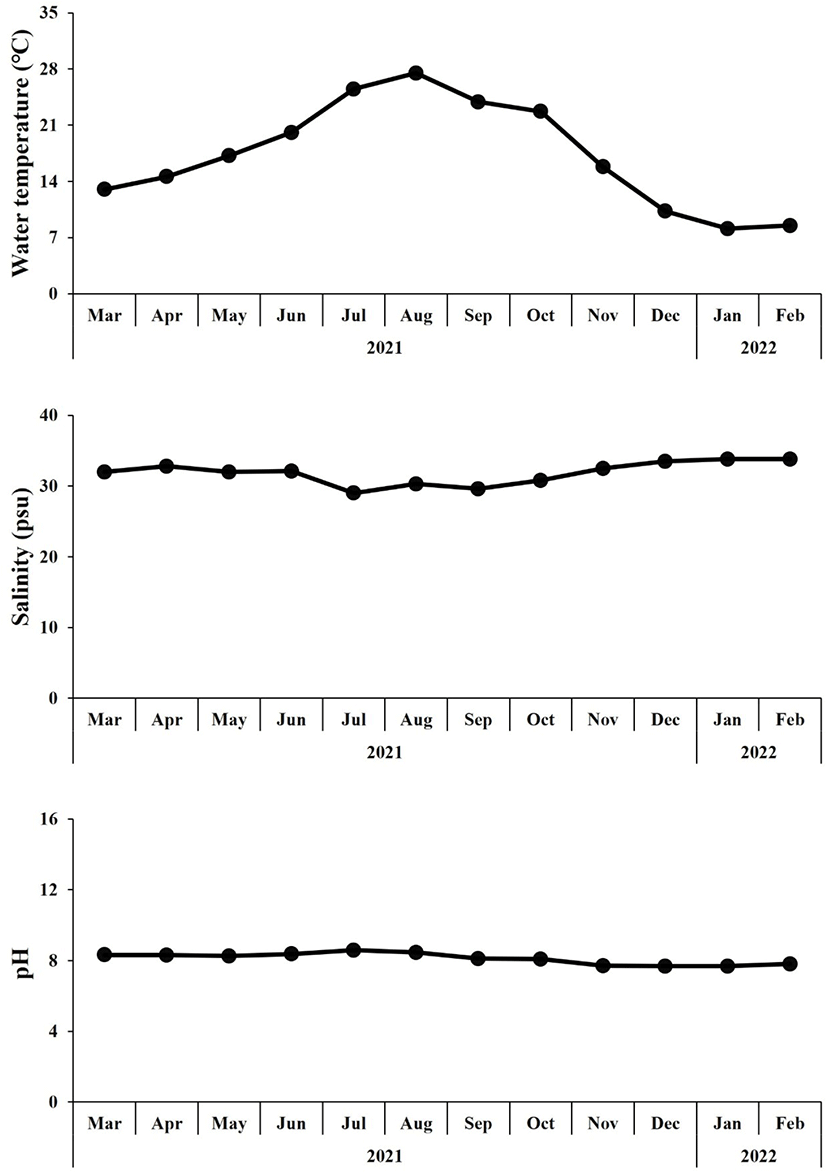
For 40 oysters every month, the shell length (SL), shell height (SH), and shell width (SW) were measured using vernier calipers, and the total weight (TW), STW was measured using an electronic balance (Vibra AJ-4200E, ViBRACANADA, Montreal, QC, Canada). The tissue weight rate (TWR) and CI were calculated using the formula of Choi et al. (2003), and the formula is as follows.
To investigate the reproductive cycle according to the developmental stage of the gonads of oysters, the soft body parts of 20 oysters were fixed in 4% paraformaldehyde every month. After that, it was stored in 70% ethanol. After cutting the gonadal tissue samples and a paraffin block was made and cut into 5 μm thickness using a microtome (Tusen, Fuzhou, China) and attached to the slide. After that, after proceeding with hematoxylin & eosin staining, it was sealed using canada balsam. Tissue slides were observed under a microscope (Olympus BX41, Olympus, Japan), and micrographs were taken using a Mosaic 2.1 analysis system (Tucsen Photonics, Fujian, China).
Proximate composition analysis was conducted according to the AOAC (2005) method using the entire soft body of oysters. Moisture was analyzed using the atmospheric pressure heating and drying method. Ash was analyzed using the direct ashing furnace method. Crude protein was determined by the Kjeldahl nitrogen quantification method, and crude fat was determined by Folch et al. (1957), the analysis was conducted.
Fatty acid methylation was analyzed by gas chromatography (Thermo Finnigan Trace GC; Austin, TX, USA) equipped with a teries silica capillary column. Detection was performed in FID mode, and quadrex (30M, bonded carbowax 0.25 mm I.D×0.25 μm film, cat. No.: 007-CW-30-0.25F, injector; Cole-Parmer, Vernon Hills, IL, USA) was used for the column. The derived fatty acid content was expressed as a relative percentage of the area of each peak.
For amino acid analysis, 0.5 g of the sample was quantified, put into a test tube, 15 mL of 6N-HCl was added, sealed under reduced pressure, and then acid hydrolyzed in a dry oven at 115°C for more than 24 hours. Filter the decomposition solution with a glass filter and concentrate the obtained filtrate under reduced pressure at 55°C to completely evaporate hydrochloric acid and water. After Then, the concentrated sample was purified in a 25 mL volumetric flask with sodium citrate buffer (pH 2.20) and filtered with a 0.45 μm membrane filter was analyzed using an amino acid automatic analyzer (Biochrom 30, Biochrom, Cambridge, UK) under the following conditions. A cation separation column (oxidized feedstuff column, 4.6 mm×200 mm) was used, and 0.2 M sodium citrate buffer (pH 3.20, 4.25), 1.2 M sodium citrate buffer (pH 6.45), and 0.4 M sodium hydroxide solution were used as the mobile phase. The flow rate of the mobile phase was 0.42 mL/min, the flow rate of the ninhydrin solution was 0.33 mL/min, the column temperature was adjusted to 48°C–95°C, and the reaction temperature was adjusted to 135°C.
All results of this experiment were expressed as mean±SD. SPSS Statistics version 27 (IBM SPSS, Armonk, NY, USA) was used for the significant difference test, and after a one-way ANOVA test was performed, a significant difference between experimental groups was confirmed at the 95% confidence level through Duncan’s post hoc test.
RESUTLS
For the oysters used in the experiment, the SL, SH, SW, TW, and STW of the oysters collected from March 2021 to February 2022 in Gadeok-do, Gyeongsangnam-do were measured (Figs. 2 and 3). Fig. 4 shows the change in the CI and tissue weight rate of oysters calculated using this. The condition was the lowest at 0.047±0.015 in November 2021 and highest at 0.105±0.032 in December 2021. The tissue weight rate was the lowest at 9.02±2.43% in November 2021, and the highest at 22.68±4.36% in May 2021.
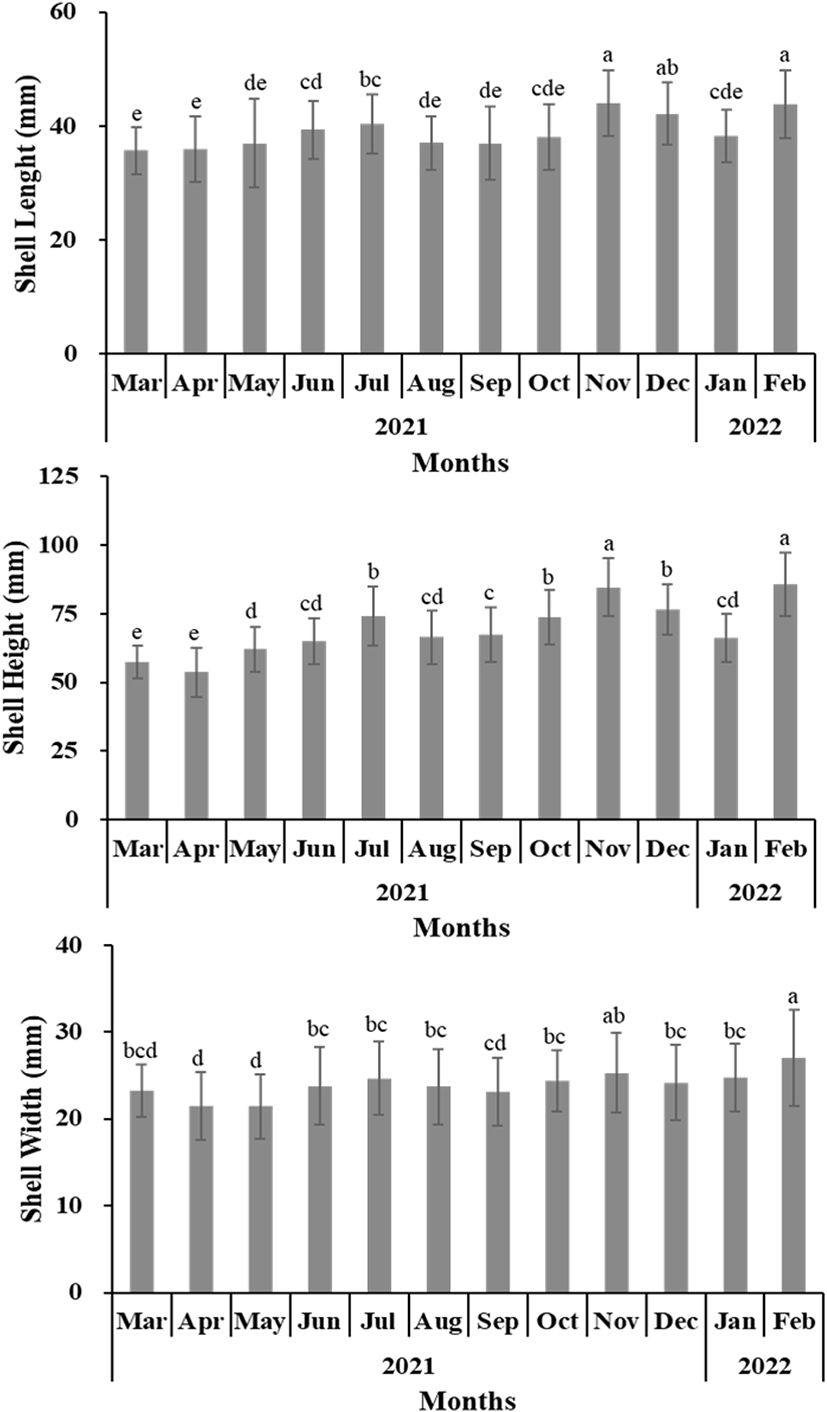
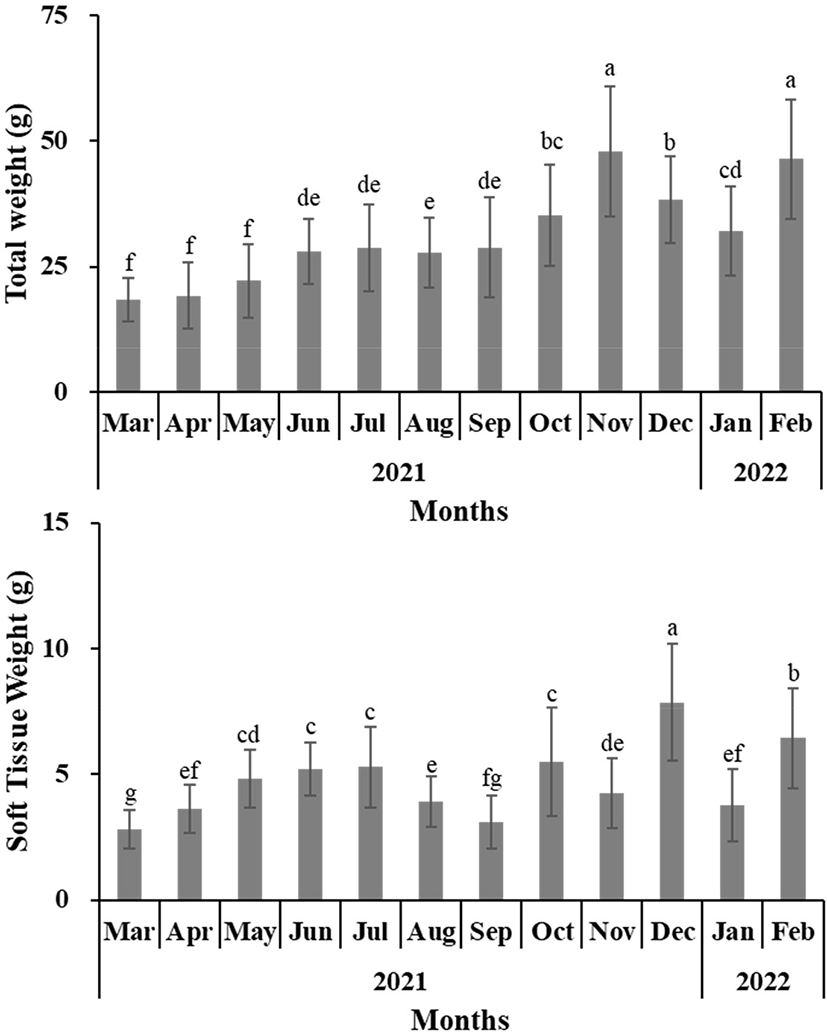
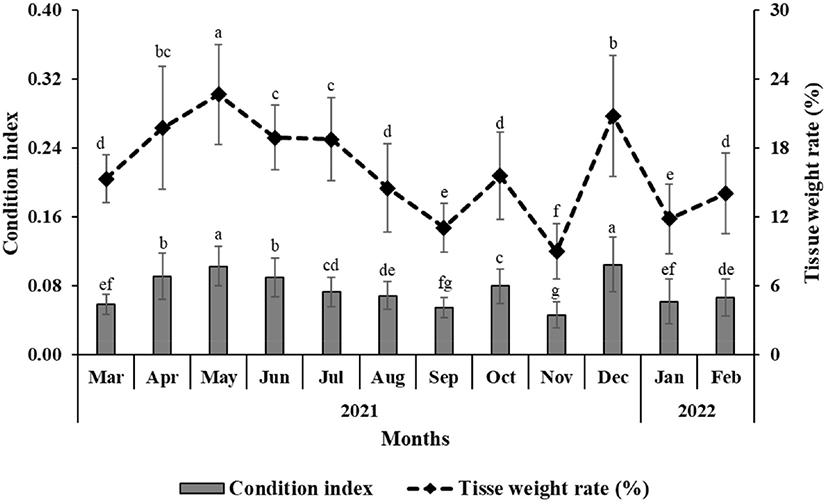
The developmental stages of oyster gonads could be divided into five stages: early development, late development, ripe, spawned, and spent/inactive, based on the shape, size, and histological characteristics of gametes.
In female oysters, the ovaries were composed of ovarian follicles, the germinal epithelium was thick, and early developing oocytes were observed along with oocytes (Fig. 5J–L). In the case of male oysters, the testis is composed of seminiferous tubules, and several developing spermatocytes have been observed (Fig. 6J–L). Both males and females were observed during the early development period from December to February.
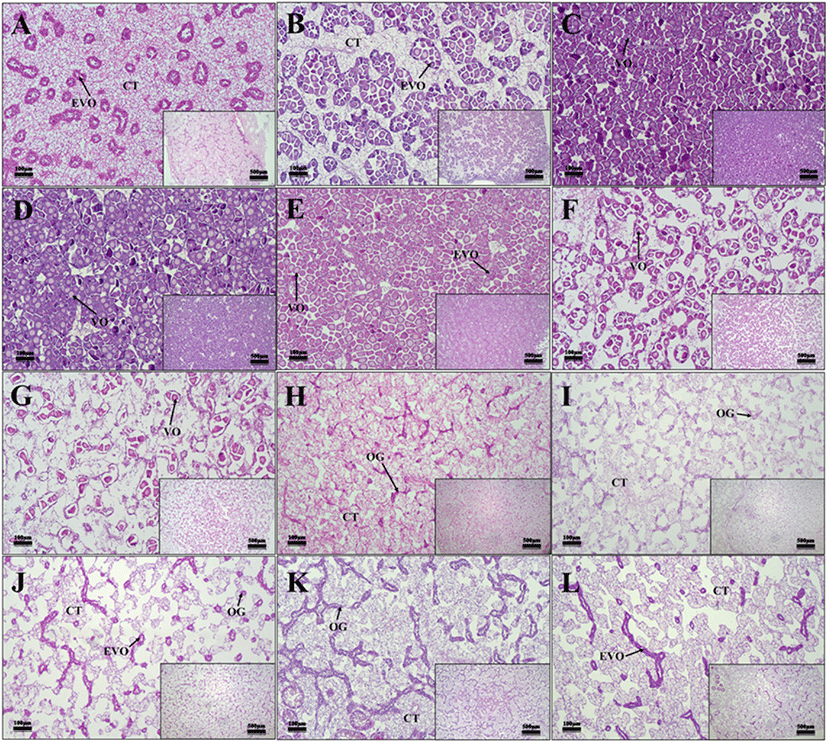
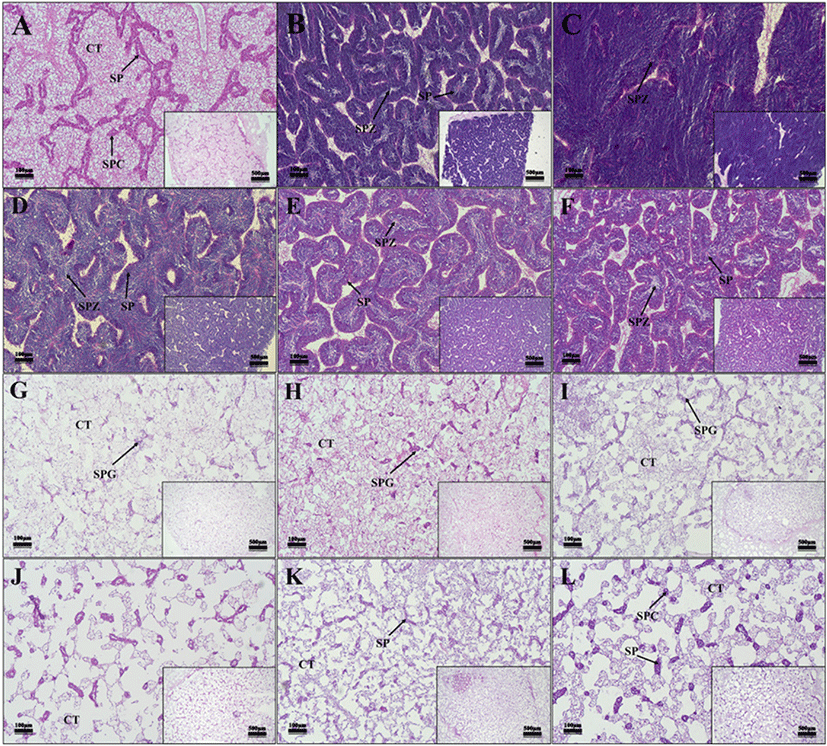
In female oysters, developing oocytes were observed (Fig. 5A and B). In male oysters, sper-matogonium and spermatocytes were observed in the lumen of the seminiferous tubules (Fig. 6A and B). Both male and female oysters were observed in the late development period from March to April.
In the case of female oysters, the germinal epithelium was thinned, and most of them were filled with fully ripened oocytes, confirming that the connective tissue disappeared (Fig. 5C–E). In the case of male oysters, like female oysters, the germinal epithelium was thinned, and ripened sperm were concentrated (Fig. 6C–E). All males and females were observed to be fully ripe from May to July.
In the case of female oysters, oocytes were released and unreleased oocytes were observed in the lumen (Fig. 5F–H). In the case of male oysters, spermatocytes were released, and unreleased sperm were observed in the lumen of the testis (Fig. 6F–H). Both males and females were observed during the spawning season from August to October.
In the case of female oysters, degeneration, or resorption of some remaining oocytes in the vesicles after spawn was observed (Fig. 5H–L). In the case of male oysters, degeneration or resorption of sperm cells after ejaculation was observed (Fig. 6H–L). Both males and females completed spawn in October and November, degeneration and absorption were observed from December to February, and gonads with empty lumen were identified.
The size of the oyster oocytes was measured from March 2021 to February 2022 (Fig. 7). Starting with an average of 15.51±7.87 μm in March 2021, mature oocytes with an average of 37.34±3.39 μm were observed in May, the maturity period. During spawning in September, the average was 4.48±1.03 μm.
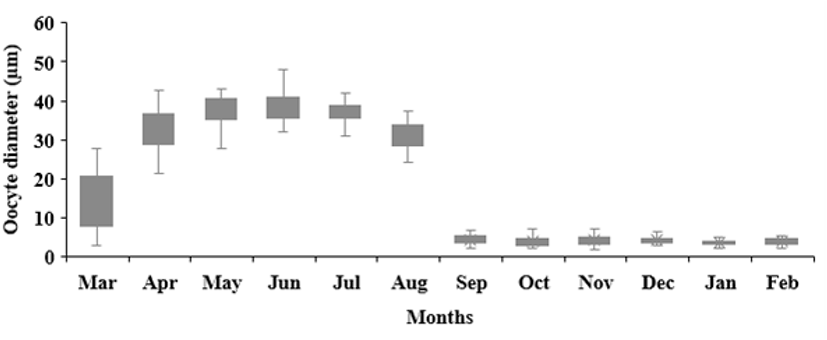
Table 1 show the results of analyzing the changes in moisture, ash, crude lipid, and crude protein content of oysters every month from March 2021 to February 2022. Moisture content was 70.26%–71.55%, with a maximum value of 71.55% in May and a minimum value of 70.26% in July (p<0.05). Ash content ranged from 3.45%–7.61%, with a maximum value of 7.61% in August and a minimum value of 3.45% in July (p<0.05). Crude lipid ranged from 1.18%–4.33%, with a maximum value of 4.33% in October and a minimum value of 1.18% in January (p<0.05). Crude protein ranged from 15.54%–18.71%, with a maximum value of 18.71% in May and a minimum value of 15.54% in September (p<0.05).
Table 2 shows the results of analyzing the fatty acids of total lipids extracted from oysters in Gadeok-do from March 2021 to February 2022. Major fatty acids were analyzed such as C14:0, C16:0, C18:0, C20:5n3, and C22:6n3, and the composition ratio was confirmed in the order of saturated fatty acid (SFA), polyunsaturated fatty acid (PUFA), and monounsaturated fatty acid (MUFA). The content of fatty acids according to seasonal changes was the highest in August (74.07%) for SFA, February (37.12%) for PUFAs, and January (6.11%) for MUFAs. SFA were lowest in January (57.09%), PUFAs in August (20.84%), and MUFAs in November (4.15%). In addition, C20:5n3, an n-3 series of PUFAs, was highest in February (21.78%) and lowest in July (8.19%). C22:6n3 also showed the highest value in February (11.52%) and the lowest value in July (5.08%).
% of DM basis, n=30 of pooled homogenates.
DM, dry matter; SFA; saturated fatty acid; MUFA, monounsaturated fatty acid; PUFA, polyunsaturated fatty acids.
Table 3 shows the results of analyzing the total amino acid content of oysters in Gadeokdo Island from March 2021 to February 2022. The major amino acids included aspartic acid, glutamic acid, lysine, arginine, leucine, proline, glycine, alanine, and other amino acids were also included. Among them, glutamic acid showed the highest ratio, showed the highest content in December, and showed the lowest content in March.
% of DM basis, n=30 of pooled homogenates, g/100 g dry weight.
DM, dry matter.
DISCUSSION
Methods for investigating the reproductive cycle of shellfish include estimating using CI and histologically confirming the degree of gonadal development (Corte, 2015; Machado et al., 2018). CI is an important indicator of the physiological state of bivalves and is related to the growth and reproductive cycles (Lucas & Peter, 1985; Matias et al., 2009). The CI increases during gametogenesis and decreases during spawning (Machado et al., 2018). In addition, the CI varies according to the season and the region where it is cultivated (Ngo et al., 2006). In the case of oysters inhabiting Stansbury, obesity increased rapidly from February to May of the following year and decreased simultaneously with spawning (Li et al., 2009). The CI of oysters cultivated in Gadeok-do was highest in May when ripening occurred, then decreased during spawning in summer, and increased again in December when early development occurred. In this study, the CI was highest in May and December 2021, which is the result of maturity in May and early development in December, which is the time to accumulate a lot of glycogens.
The reproductive cycle, including gametogenesis and spawning, differs among bivalve species, and the timing and duration of each stage of the reproductive cycle in oysters vary according to the season and geographical location (Cotter et al., 2010; Hassan et al., 2018). In this study, when the annual reproductive cycle of Gadeok-do oysters was confirmed through histological analysis, males and females could not be distinguished due to degeneration and inactive phases in autumn. After autumn, gametogenesis started in early winter, and gamete development, ripe, and spawning took place in spring and summer. This showed a pattern like the reproductive cycle reported in C. gigas (Lango-Reynoso et al., 2000).
One of the important factors in the reproductive cycle of marine invertebrates is water temperature (Kim et al., 2009). C. gigas follow a reproductive cycle in which gametogenesis begins in winter when the water temperature is low, continues until spring, and matures and spawns in summer (Fabioux et al., 2005). The seawater temperature measured at Gadeok-do was the highest at 27.5°C in summer and the lowest at 8.1°C in winter. As in the above study, in this study, the gonads of oysters matured with the rise in water temperature, and it was confirmed that spawning occurred in both males and females in August when the water temperature reached the highest.
Since histological analysis is a quantitative method, oocyte size measurement, was performed to confirm the pattern observed in the qualitative analysis. In shellfish, the oocyte size is an indicator related to the reproductive cycle including gametogenesis and is one of the methods for evaluating gamete development (Lango-Reynoso et al., 2000). Oocyte size is known to be influenced by the environment, age, and propagation strategy (Mladineo et al., 2007). As with other bivalves, the largest oocytes in oysters in this study were identified in individuals at the time of maturation and spawning (Lango-Reynoso et al., 2000; Mladineo et al., 2007).
The reproductive cycle of shellfish and energy storage and utilization are closely related (Camacho et al., 2003). It is controlled and influenced by temperature and food availability, and influences growth and maturation (Camacho et al., 2003; Joaquim et al., 2011). This cycle of energy storage and use varies depending on the species and habitat, and the biochemical composition varies depending on the season (Matias et al., 2009). In this study, changes in general components of oysters from Gadeok-do were highest in moisture and crude protein in May, crude ash content in August, and crude lipid in October. Protein acts as an energy storage material during the maturation process of oysters, and lipids are known to decrease during the summer, the spawning season, because they affect the development and spawning of germ cells (Barber & Blake, 1981; Dridi et al., 2007). In addition, in winter and spring, energy is converted into lipids and stored (Liu et al., 2010). At the same time, the oyster gonads mature in the spring and internal lipid storage increases the weight and lipid content of oysters (Berthelin et al., 2000). In this study, as in the above study, the protein was high in May, when oysters matured, and it is thought that it was used as an energy substance for maturation. In addition, in this study, the lipid content of oysters showed a tendency to continuously decrease during the summer spawning season, which is thought to have been used as energy during spawning.
SFAs provide energy and serve as precursors for unsaturation and extension reactions during the synthesis of long-chain fatty acids, including PUFAs (Renaud et al., 2002). Fatty acids, as components constituting the basic structure of lipids, serve as precursors of substances that exhibit physiological activity and perform structural and functional roles in organisms such as bioregulation and stress response (Makoto et al., 1989). The SFA showing high content in this study is C16:0, which is produced through the fatty acid metabolism of all animals and plants and is known to have a high content because PUFA is synthesized from it (Gabbott, 1983). Fatty acids stored in the body are greatly affected by food, and C18:2n-6, and C18:3n-3 are found to produce C20:4n-6, C22:6n-3 through desaturation and elongation reactions of the same precursor (Mennicken et al., 2005). Also, in the case of bivalve, the content of DHA (22:6n-3) and EPA (20:5n-3) is high (Jeong et al., 1999). Both EPA and DHA show a low content in the spawning period and a high content in the maturation period, and it is known that DHA acts on the structure and function of the cell membrane according to the development of gametes and EPA acts on the energy function (Dirdi et al., 2007). As in the above study, in this study, the EPA and DHA content of oysters was high from March to May, from late development to ripe, and low from June to September, from ripe to spawning.
Amino acids are important as constituents of bioactive substances and are known to affect taste themselves (Hayashi et al., 1981). In oysters grown in Tongyeong and Goseong, glycine, alanine, and glutamic acid were identified as major amino acids, which are mainly known as taste components (Choi et al., 2017). Oysters from the west coast mainly contained amino acids such as taurine, glutamic acid, aspartic acid, and alanine (Hwang et al., 2016). In this study, aspartic acid, glutamic acid, proline, alanine, leucine, lysine, and arginine were the main amino acids in the Gadeok-do oyster, and other amino acids were evenly contained. Park et al. (1994), arginine is known to be involved in energy metabolism in shellfish. In this study, the contents of arginine, aspartic acid, and lysine were highest in spring when late development began and low in summer when maturation and spawning occurred.
In conclusion, this study is the first report on the reproductive cycle of oysters in Gadeok-do, Gyeongsangnam-do. Gadeok-do oysters had a breeding cycle that went through five stages: early development, late development, ripe, spawned, and spent/inactive. In Gadeok-do oysters, the CI decreased during the midsummer and early autumn, the spawning season, and increased again when they entered the degenerative period after spawning. The protein content of Gadeok-do oysters was high in May, the ripening season, and the lipid content continued to decrease in summer, the spawning season. It is considered that proteins and lipids are used as the main energy in the reproductive development stage as the main energy materials and change in relation to the reproductive cycle of oysters. Both major fatty acids, EPA, and DHA, were low during the spawning period and high during the ripe period. Among amino acids, arginine, aspartic acid, and lysine in Gadeok-do oysters were high in spring, the late stage of development, and low in summer, when full ripening and spawning took place. As such, it was confirmed that there is a close relationship between the growth and biochemical changes of oysters and the reproductive cycle. Through this study, it is considered will be used as important basic data in understanding the reproductive cycle of Gadeok-do oysters.