INTRODUCTION
Stem cells possess the unique ability to self-renew in addition to generating differentiated progeny. The Drosophila ovary offers an incisive genetic system that allows for dissection of the molecular mechanism of stem cell self-renewal (Xie & Spradling, 2000). Two female germline stem cells (GSCs) reside at the apical tip of the germarium, a structure located at the anterior tip of the ovariole, and are physically linked through adherent junctions to niche cells, called cap cells (Xie & Spradling, 1998). During self-renewal stem cell division, one stem cell daughter attached to the cap cells remains as a stem cell while the other, which is one cell distant from the cap cells, starts to differentiate due to reduction of niche signals (Xie & Spradling, 2000). It becomes a cystoblast (CB), which undergoes incomplete mitotic cell division four times to produce a cyst composed of 16 germline cells. Ultimately, 15 of those cells differentiate to nurse cells and one becomes an oocyte (Lin & Spradling, 1993).
In addition to signals from the cap cells, maintaining the stemness of GSCs also requires stem-cell-specific intrinsic factors (Parisi & Lin, 1999; Wang & Lin, 2004). The RNA binding and translational repressor Pum is expressed in GSCs and is required for stemness (Parisi & Lin, 1999; Harris et al., 2011). In pum mutant ovary, GSCs lose their stemness and undergo differentiation, depleting the germarium (Lin & Spradling, 1997; Parisi & Lin, 1999). This suggests that Pumilio (Pum)’s role is to block differentiation of stem cells by inhibiting intrinsic differentiation-promoting factors, though the specific factors it targets remain mostly unknown (Szakmary et al., 2005). The C-terminal Puf motif of Pum recognizes the Pum binding sequence (PBS) in the 3’UTR of target mRNAs (Wharton et al., 1998; Edwards et al., 2001; Weidmann et al., 2016; Qiu et al., 2019), while its N-terminal domain mediates translational repression through molecular mechanisms that are as yet poorly understood (Kim et al., 2010; Weidmann & Goldstrohm, 2012; Arvola et al., 2020).
Bag-of-marbles (Bam) is not expressed in GSCs, but is expressed in the 2-, 4-, and 8-cell CBs, wherein Bam promotes differentiation (McKearin & Spradling, 1990; Lavoie et al., 1999). In the bam mutant ovary, GSC daughters fail to undergo differentiation, culminating in population of the germaria with stem-cell-like cells (McKearin & Spradling, 1990). Forced ectopic expression of Bam in GSCs promotes their differentiation (Ohlstein & McKearin, 1997; Ohlstein et al., 2000), raising the hypothesis that Bam might inhibit Pum activities. We previously tested this hypothesis in vitro and found that Bam physically interacts with the N-terminus of Pum (Kim et al., 2010). We further showed that this physical interaction is essential to inhibition of Pum translational repression activity in cultured cells (Kim et al., 2010). In this work, we design a GFP-based translational reporter bearing the PBS in its 3’UTR and show that this translational reporter is a Pum activity sensor that faithfully monitors Pum activity in the germarium. We furthermore use this sensor to show that Bam inhibits Pum activity in vivo in the Drosophila ovary.
MATERIALS AND METHODS
All strains were grown at 25°C on standard yeast, cornmeal agar medium. pum1/TM6 and pumMsc/TM6 flies were obtained from Dr. Wharton. hs-bam flies were a gift from Dr. McKearin.
The Pum activity sensor was constructed by introducing the PBS into the p2035 vector (Brennecke & Cohen, 2003). The PBS, which is present in the nanos response element (NRE) (+97 to +148) of the hunchback 3’UTR, was amplified by PCR (using primers ctagaattattttgttgtccaaaattgtacataagccgaattcc, tcgaggaattcggcttatgtacaattttggacaacaaaataatt) and inserted between the XbaI and XhoI restriction sites of the p2035 vector. The vector construct was then injected into w1118 embryos to produce the Pum activity sensor transgene.
Ovaries were dissected, fixed, and stained as described previously (Ohlstein & McKearin, 1997). In brief, ovaries were treated for 30 min with fixing solution (4% paraformaldehyde, 0.1% Tween 20). After several washes using PBS with 0.1% Tween 20 (PBST), the samples were preabsorbed for one hour with PBST containing 10% normal goat serum, then incubated with the primary antibody overnight at 4°C. After washing with PBST, the ovaries were incubated with the secondary antibody for two hours at room temperature. After again washing with PBST, the samples were mounted in Vectashield (Vector Laboratories, Road Burlingame, CA, USA). Confocal images were taken and processed by a Zeiss LSM510 Microscope. The GFP level in the area at the apical tip of each germarium was quantified by ImageJ (National Institutes of Health, Bethesda, MD, USA) and the data were graphed using Sigma Plot.
Mouse monoclonal anti-1B1 antibody (1:20) was purchased from Developmental Studies Hybridoma Bank (Iowa City, IA, USA). Rabbit polyclonal anti-GFP (1:2,000) was obtained from Invitrogen (Carlsbad, CA, USA). Goat anti-mouse and goat anti-rabbit IgG conjugated to Alexa 488 or Alexa 555 (1:200) were purchased from Molecular Probe (Eugene, OR, USA).
RESULTS AND DISCUSSION
We constructed a translational reporter to monitor translational repressive activity of Pum in vivo (Fig. 1A), henceforth termed the Pum activity sensor. This reporter consists of the tubulin promoter upstream of the GFP coding sequence which has the PBS in its 3’UTR. The PBS (UGUAXAUA) was taken from the NRE of the hunchback 3’UTR (Wharton & Struhl, 1991; Murata & Wharton, 1995; Zamore et al., 1997; Kim et al., 2010). We expected that Pum would bind the PBS in the reporter and repress translation of GFP. If so, GFP would not be expressed in cells with active Pum, but would be expressed in cells lacking Pum activity, thereby enabling the monitoring of Pum activity.
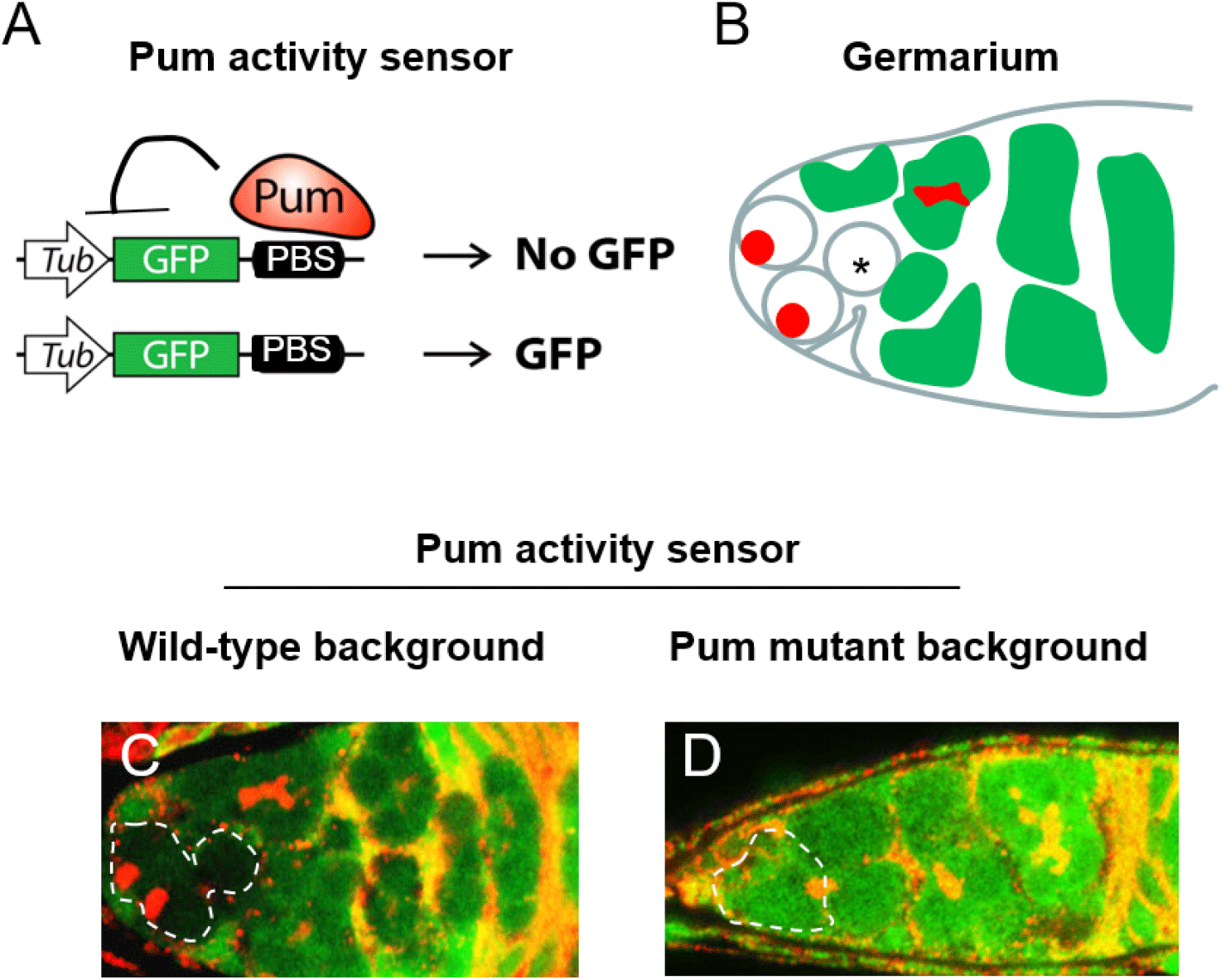
Two cells located at the apical tip in the germaria of the Drosophila ovary are GSCs that possess a spectrosome (or round fusome) recognizable by 1B1 antibody (Fig. 1B) (Deng & Lin, 1997). Mitotic cell division of the GSCs produces differentiating germline cysts (consisting of two, four, eight, and 16 cells successively) that are recognized by the presence of a branched spectrosome or fusome, which is also detectable with 1B1 antibody (Lin & Spradling, 1995; Deng & Lin, 1997).
We examined whether the Pum activity sensor faithfully monitors Pum activity in the germaria of the ovary. First, transgenic flies harboring the Pum activity sensor were generated. Ovaries from those transgenic flies were dissected and co-stained with GFP and 1B1 antibodies. In wild-type ovary, GFP expression was severely reduced in the GSCs and in immediate daughter cells derived from their mitotic cell division, which is consistent with reports that Pum is active in the GSCs and immediate daughter cells (Fig. 1C). Meanwhile, GFP was strongly expressed in the germline cyst cells, consistent with reports that Pum is not active in those cells.
To further validate the Pum activity sensor, we examined whether the observed repression of GFP expression requires Pum. Ovaries from transheterozygous mutant (pum1/pumMsc) flies (Wharton et al., 1998) were stained with GFP and 1B1 antibodies. GFP was found to be expressed in all cells (Fig. 1D), demonstrating that its repression requires Pum activity. Combined with the above, these findings confirm that the Pum activity sensor faithfully reports Pum activities in the ovary.
We previously showed that Bam inhibits Pum activity in cultured cells (Kim et al., 2010). Here we examined the in vivo relevance of this finding using the Pum activity sensor. To overexpress Bam in all cells including GSCs, we used the hs-bam transgene (Ohlstein & McKearin, 1997), which consists of a heat-shock promoter upstream of the bam coding sequence and enables ubiquitous expression of bam upon a brief heat shock. hs-bam flies also harboring the Pum activity sensor were subjected to a brief heat shock (37°C, two hours with one-hour interval) and their ovaries were dissected and co-stained with GFP and 1B1 antibodies at 1, 2, 6, 15, 24, 30, 42, and 48 h post-heat shock (PHS).
Control (no heat shock) flies exhibited no GFP in the GSCs (Fig. 2A). At 2 h PHS, a slight increase of GFP occurred in GSCs at the anterior tip (Fig. 2B, E). At 24 h PHS, peak GFP expression was observed (Fig. 2C, E, and Table 1). At 48 h PHS, GFP expression was severely reduced (Fig. 2D, E, and Table 1). In short, induction of Bam by a brief heat shock induced gradual increase of GFP in the GSCs, followed by a decrease (Fig. 2E and Table 1). This suggests that a gradual increase of Bam in turn gradually inhibits Pum activity in a temporal manner. It is of note that the gradual increase of GFP, which reflects a gradual decrease of Pum activity, is accompanied by a gradual decrease in the number of GSCs at the apical tip of the germaria, which is consistent with Pum activity being required for stem cell maintenance.
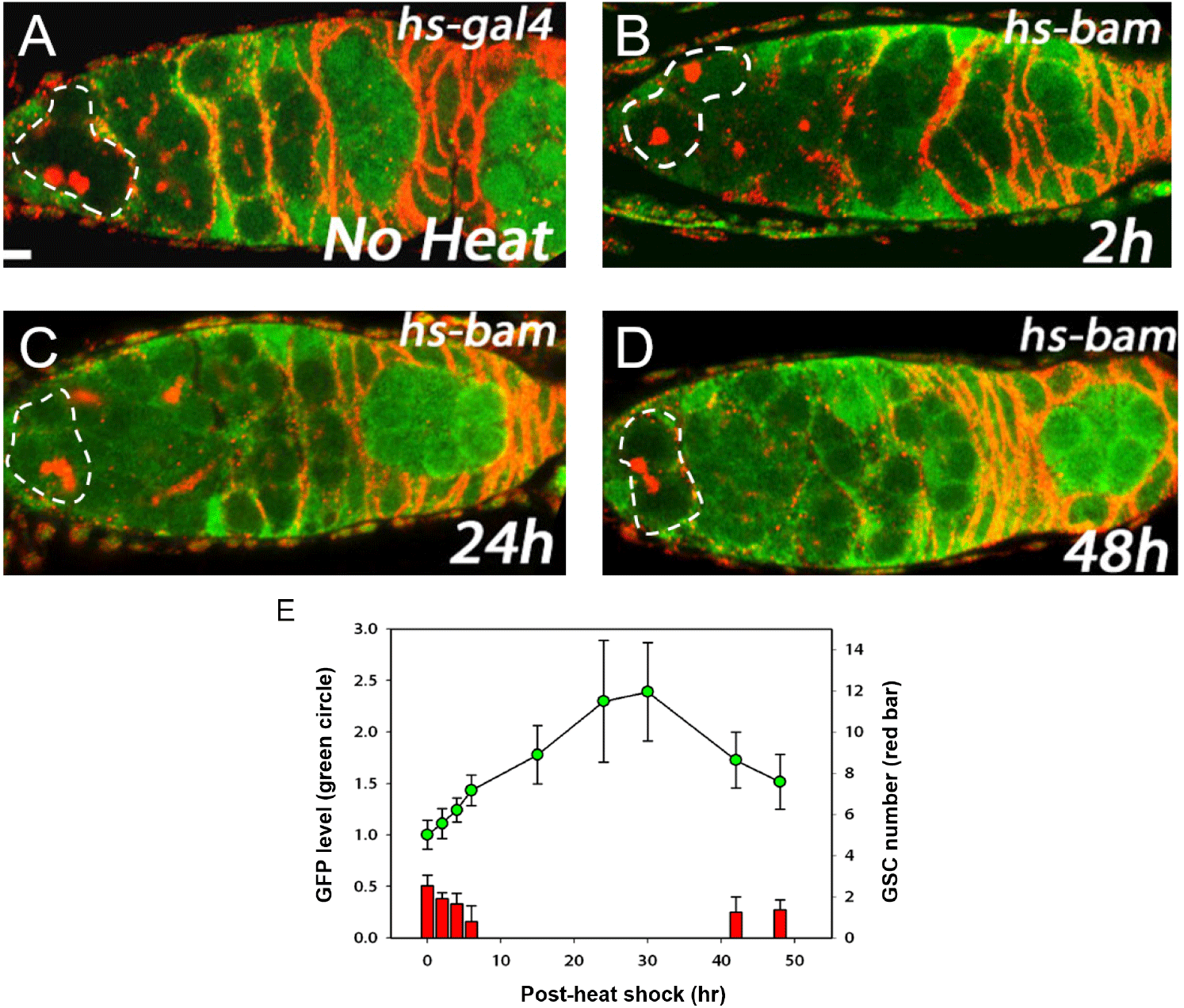
GFP levels in the area of the GSCs at the apical tips of germaria (circled areas in Fig. 2A–D) were quantified using Image J (NIH).
GFP level was normalized to the observed in the no heat shock control. Spectrosome-containing cells at the apical tips of germaria were counted as GSCs. PHS denotes post-heat shock. n indicates the number of germaria examined.
Data are presented as mean (±SD).
GFP, green fluorescence protein; GSC, germline stem cell; PHS, post-heat shock.
Here we examine the physiological relevance of previous findings that Bam inhibits Pum activity in cultured cells. We generated an in vivo Pum activity sensor consisting of a translational GFP reporter that harbors the PBS in its 3’UTR. The sensor faithfully reported Pum activity in the GSCs of the Drosophila ovary; namely, GFP expression was severely reduced in the GSCs, which contain active Pum. In addition, we used the sensor to show that ectopically expressed Bam inhibits Pum activity, which was monitored as increased GFP level. Bam physically interacts with the N-terminus of Pum, which mediates translational repression, possibly through interaction with other proteins (Fig. 3A). Our findings suggest that Bam inhibits Pum activity through blocking such interactions (Fig. 3B).
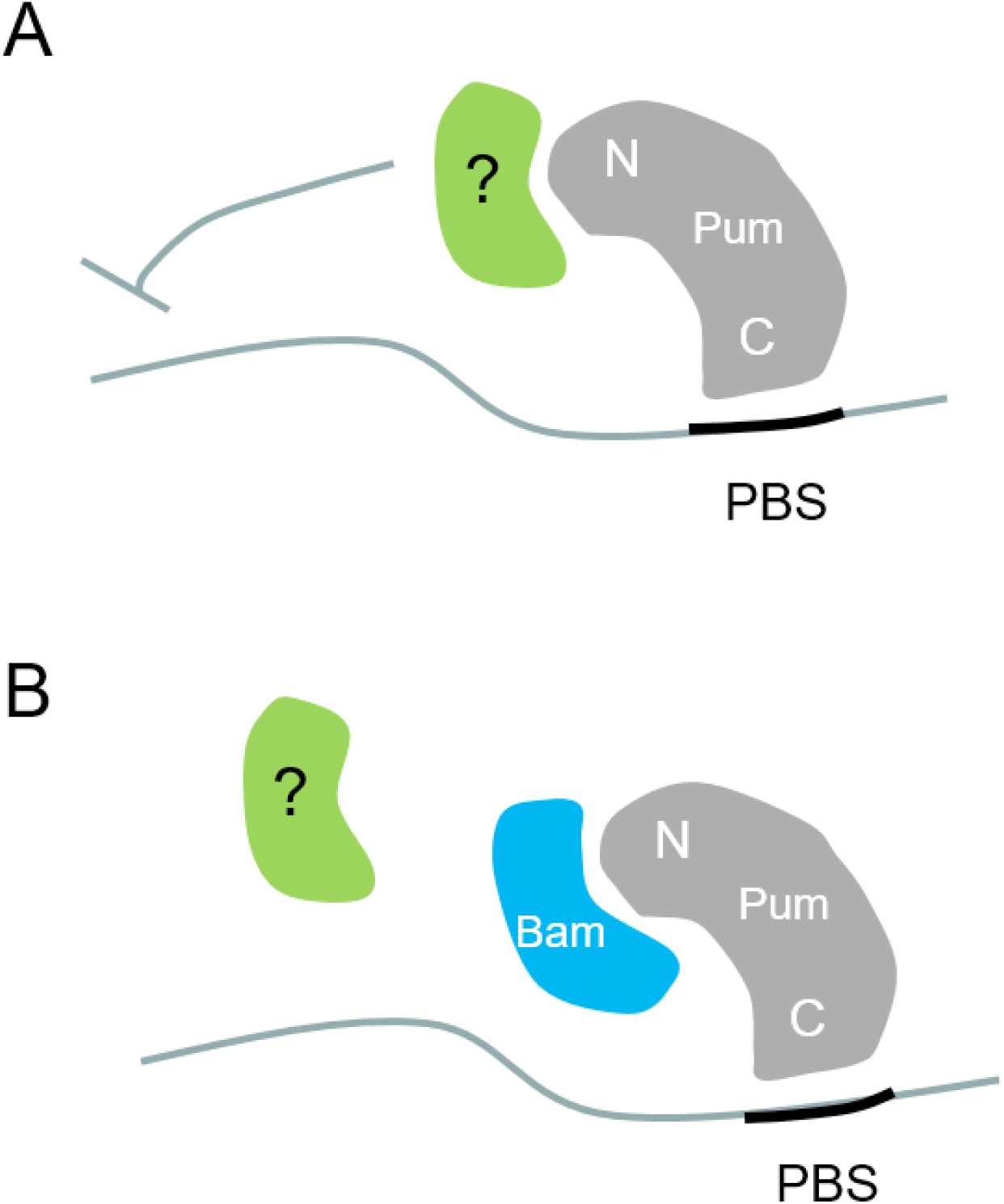