INTRODUCTION
Hair is a major skin appendage that plays an important role in protection, thermoregulation, and sensory function (Stenn & Paus, 2001). However, various damaging factors, such as aging, hereditary, inflammation, and physiological stress, induce excessive hair loss regardless of gender or age (Strazzulla et al., 2018). Given that hair deeply influences an individual’s appearance, unwanted hair loss can result in lower self-esteem and quality of life, leading to psychological problems (Grimalt, 2005). Many studies have been conducted on hair loss and treatments, and two Food and Drug Administration (FDA)-approved drugs have been developed: minoxidil and finasteride (Lee et al., 2018; Suchonwanit et al., 2019). However, the effects of the drugs vary from person to person, and there is often a variety of side effects (Rossi et al., 2012; Carreño-Orellana et al., 2016). In particular, finasteride is applied only to male patients as a treatment for male-pattern hair loss (Seale et al., 2016). Hence, developing effective therapeutics for all types of hair loss is an urgent task.
Hair grows up from the hair follicle (HF), a complex mini-organ that includes nerves, blood vessels, sebaceous glands, and arrector pili muscles (Schneider et al., 2009). After embryonic HF morphogenesis, the HF repeats a cycle consisting of the anagen, catagen, and telogen phases to regenerate hair for life (Stenn & Paus, 2001; Schneider et al., 2009). Anagen is referred to as an active growth phase because follicular cells actively proliferate and remodel the HF structure for maturation and pigmentation of hair fibers (Schneider et al., 2009). Mature HFs begin to regress as they move into the catagen phase, which features an increase in apoptosis (Schneider et al., 2009). Overall HF length is substantially reduced during the catagen phase, and the HF proceeds to the telogen, or resting phase (Schneider et al., 2009). In the telogen phase, HFs are inactivated and have minimal length (Müller-Röver et al., 2001). Cyclic conversion to the anagen phase follows the telogen phase (Chen et al., 2020a). Hence, for normal hair growth after embryonic HF morphogenesis, the transition from the telogen to the anagen phase is a key step for hair regeneration (Chen et al., 2020a).
The transition is controlled by the delicate balance of signaling molecules in HFs, which mediate the activation of HF stem cells (HFSCs) and mesenchymal dermal papilla cells (DPCs) (Chen et al., 2020a). Several signaling molecules, such as Sonic hedgehog (Shh), Wnt/β-catenin, and bone morphogenetic protein (BMP) 2/4, have been shown to be involved in hair regeneration by regulating the activities of HFSCs and DPCs (Rishikaysh et al., 2014). Shh and Wnt/β-catenin signaling induce the proliferation of HFSCs and maintain the differentiation potential of DPCs to hair, promoting hair regeneration (Rishikaysh et al., 2014; Avigad Laron et al., 2018). While BMP2 and BMP4 signaling results in inactivation of HFSCs and prevention of them from progressing to HF regeneration by holding HFs in a refractory telogen phase (Botchkarev et al., 2002; Zhang et al., 2006). However, the crosstalk between these stem cells via signaling molecules is complex and poorly understood. Even though several signaling molecules regulating the hair regeneration activities of HFSCs and DPCs have been studied, it remains unclear how the activation of these molecules is triggered. In addition, skin is a complex organ in which more than 50 cell types contribute to skin structures (Abaci et al., 2018); hence, the effects of the various cells surrounding HFs on stem cells are not fully proven.
Formyl peptide receptor 2 (FPR2), which belongs to the G protein-coupled receptor family, is an important regulator of inflammation (Chen et al., 2020b). Recent studies have investigated the expression and role of FPR2 in nonphagocytic cells, and several have reported that various types of stem cells and dermal fibroblasts express FPR2, and that activation of FPR2 promotes migration and proliferation of stem cells, such as neural stem cells and dental stem cells (VanCompernolle et al., 2003; Zhang et al., 2017; Gaudin et al., 2018). Tsuruki et al. showed that FPR2 prevented hair loss in rats that received a chemotherapy drug (Tsuruki & Yoshikawa, 2006). FPR2 agonists, such as N-formyl-Met-Leu-Phe (fMLP) and MMK-1, suppressed hair loss by activating nuclear factor kappa-light-chain-enhancer of activated B cells (NF-κB) signaling, which blocked apoptosis (Tsuruki & Yoshikawa, 2006). It was proved that NF-κB activated by the FPR2 agonist was necessary for HFSC proliferation and activation for the induction of the anagen phase (Krieger et al., 2018). Krieger et al. also reported that NF-κB activity was detected in anagen induction, and mice with HF-specific inactivation of NF-κB had a delayed transition of telogen to anagen phase compared to control mice (Krieger et al., 2018). Given that FPR2 is expressed in various types of stem cells and its protective role in hair loss, we hypothesized that FPR2 might be involved in the HF cycle and impact hair regeneration. We found that Fpr2 knockout (KO) mice experienced extensive hair loss in the dorsal skin 18 weeks after birth, and that the hair loss was caused by Fpr2 deficiency-induced inactivation of HFSCs and DPCs. These findings suggest the therapeutic potential of FPR2 in the treatment of hair loss.
MATERIALS AND METHODS
Male C57BL/6 mice (6-week-old) were purchased from Hyochang (Daegu, Korea) and used for wild-type (WT) controls. Male Fpr2 KO mice were generously donated from Dr. Kim (Pusan National University School of Medicine, Yangsan, Korea). Mice were housed with 12-hour light/dark cycle with free access to normal chow diet and water. 18-week-old mice were sacrificed to obtain skin sample. All animal care was carried out according to the provisions of the National Institutes of Health (NIH) guidelines for the care and Use of Laboratory. The animal protocol used in this study has been approved by the Pusan National University–Institutional Animal Care and Use Committee (PNU-IACUC) on their ethical procedures and scientific care (Approval Number PNU-2020-2574).
Total protein was extracted from dorsal skin tissues that had been stored at −80°C. Samples were cryo-pulverized and the tissue powder was mixed in triton lysis buffer (TLB) supplemented with protease inhibitors (Complete mini; Roche, Indianapolis, IN, USA) and centrifuged at 13,000 r.c.f. for 15 minutes at 4°C. The supernatants containing protein extracts were used in subsequent biochemical analysis. Protein concentration was measured by Pierce BCA Protein Assay Kit (Thermo Fisher Scientific, Waltham, MA, USA). To denature and reduce protein samples, proteins were boiled in 5X sample buffer containing β-mercaptoethanol and sodium dodecyl sulfate (SDS) at 100°C for 10 minutes. Total 70 μg of protein lysates was separated by SDS-polyacrylamide gel electrophoresis (PAGE) on 10% tris-glycine gel and transferred onto a 0.45 μm pore size polyvinylidene difluoride (PVDF) membrane (Millipore, Darmstadt, Germany). Primary antibodies used in this study were as follows: rabbit anti-FPR2 antibody (diluted 1:100; Novus Biologicals, Centennial, CO, USA), rabbit anti-Shh antibody (diluted 1:100; Santa Cruz Biotechnology, Dallas, TX, USA), rabbit anti-nonphospho β-catenin antibody (diluted 1:1,000; Cell Signaling Technology, Danvers, MA, USA), rabbit anti-BMP4 antibody (diluted 1:1,000; R&D Systems, Minneapolis, MN, USA), rabbit anti-BMP2 antibody (1:1,000; Bioss, Woburn, MA, USA), mouse anti-Keratin 15 antibody (1:1,000; Thermo Fisher Scientific), rabbit anti-CD133 (1:1,000; abcam, Cambridge, MA, USA) and mouse anti-glyceraldehydes 3-phosphate dehydrogenase antibody (GAPDH) (diluted 1:1,000; Bio-rad, Hercules, CA, USA) were used in this experiment. Horseradish peroxidase-conjugated anti-rabbit or anti-mouse IgG (Enzo Life Sciences, Farmingdale, NY, USA) was used as secondary antibody. Protein bands were detected using an EzWestLumi ECL solution (ATTO, Tokyo, Japan) as per the manufacturer’s specifications (ATTO, Ez-Capture II). Densities of protein bands were measured using CS Analyzer software (Version1.0.3, ATTO).
Skin specimens were fixed in 10% neutral buffered formalin (Sigma-Aldrich), embedded in paraffin and cut into 6-μm-thick sections. Sections were deparaffinized, hydrated, and stained in hematoxylin (Gill’s Hematoxylin V, Muto Pure Chemicals, Tokyo, Japan) for 15 min at room temperature. And then stained in eosin (1% Eosin Y Solution, Muto Pure Chemicals) for 5 min at room temperature. Slides were viewed with an Olympus CX41 light microscope (Olympus Optical, Tokyo, Japan) and morphometric analysis of stained regions in the tissue sections was performed using cellSens software (Olympus Optical).
For immunofluorescence staining, skin tissues were embedded in OCT (ScigenTM, Paramount, CA, USA) and immediately frozen on dry ice. Frozen blocks were cut into 10 μm using a cryostat (Leica 3050s, Leica Biosystems, High Peak, UK), and tissue sections were thaw mounted onto glass slides. Slides were fixed in and permeabilized with acetone and methanol, respectively. They were washed with tris-buffered saline (TBS) and incubated with blocking solution (Dako, CA, USA) for 30 minutes. Sections were incubated with primary antibody, rabbit anti-FPR2 antibody (diluted 1:1,000; Novus Biologicals), rabbit anti-Shh antibody (diluted 1:150; SantaCruz, Dallas, TX, USA), rabbit anti-β-catenin antibody (diluted 1:800; Cell Signaling Technology), rabbit anti-BMP4 antibody (diluted 1:100; R&D Systems), rabbit anti-BMP2 antibody (diluted 1:500; Bioss), mouse anti-Keratin 15 antibody (diluted 1:500; Thermo Fisher Science), rabbit-anti CD133 antibody (diluted 1:100; abcam) for 4°C overnight. Slides were washed in TBS and incubated with fluorescein labelled secondary antibody, Alexa Fluor 568 anti-rabbit IgG (Invitrogen, Carlsbad, CA, USA) and Alexa Fluor 488 anti-mouse IgG (Invitrogen) for 30 min at room temperature. For double immunofluorescence staining, slides were removed from secondary antibody solution and washed with TBS. Slides were incubated with blocking solution for 10 minutes, then with secondary primary antibody, α-SMA (diluted 1:500; abcam) for 2 hours at room temperature. Slides were washed in TBS and incubated with fluorescein-labelled secondary antibody, Alexa Fluor 488 anti-mouse IgG (Invitrogen) for 30 minutes at room temperature. Slides were mounted on slides antifade mounting medium with 4′,6-diamidno-2-phenylinole (DAPI, VectaShield, Burlingame, CA, USA). Slides were viewed with a Leica DMi8 microscope (Leica Microsystems, Wetzlar, Germany).
RESULTS
Although WT and Fpr2 KO mice had normal hair growth after birth, severe hair loss in the dorsal skin was observed in Fpr2 KO mice with an average age of 18 weeks, but not in WT mice (Fig. 1A). To examine histological differences in the dorsal skin of these mice, we conducted the H&E staining. The skin structure of the WT mice showed a thin hypodermis adipocyte layer and evenly distributed HFs in the dermis (Fig. 1B). All HFs were upright toward the epidermis, and appendages such as sebaceous glands also had normal structure. However, the skin structure of the Fpr2 KO mice was different from that of the WT mice. The hypodermis adipocyte layer was much thicker in KO mice than in WT mice, and HFs were clustered or irregularly distributed in KO mice. Moreover, the HF structure was abnormal, presenting a bent and cyst-like structure. Sebaceous glands also had cyst-like structures in the KO mice. The distorted structure of the dorsal skin in the KO mice indicated that Fpr2 deletion might be involved in generating abnormal HFs.
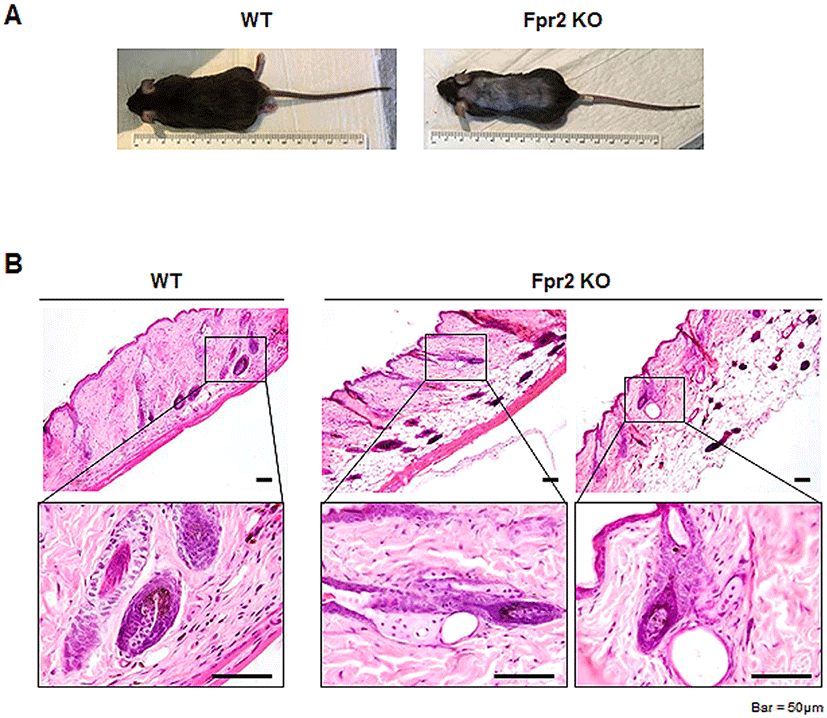
Before investigating the role of Fpr2 in the skin, we first assessed Fpr2 expression in dorsal skin tissue. Using western blot analysis, we found that WT mice had a significantly increased expression of Fpr2, whereas Fpr2 KO mice rarely expressed Fpr2 in the dorsal skin (Fig. 2A, B). In line with quantification data, Fpr2-expressing cells were evident in the outer layer of the lower HF and scattered in the dermis (indicated by arrows) of the WT mice (Fig. 2C). However, these cells were hardly detected in the Fpr2 KO mice. To identify the cells that were positive for Fpr2, we conducted double immunofluorescence staining for Fpr2 and α-Smooth muscle actin (α-Sma), a well-known fibroblast marker (Fang et al., 2013). Because FPR2 is reportedly expressed in dermal fibroblasts (VanCompernolle et al., 2003), we expected to detect Fpr2 expression in these cells. Expression of α-Sma colocalized with Fpr2-positive cells in the outer layer of HFs in WT mice (Fig. 2D). These double-positive cells were not observed in the Fpr2 KO mice. These results demonstrate that Fpr2 is expressed by dermal fibroblasts located in the outer layer of the HF and dermis.
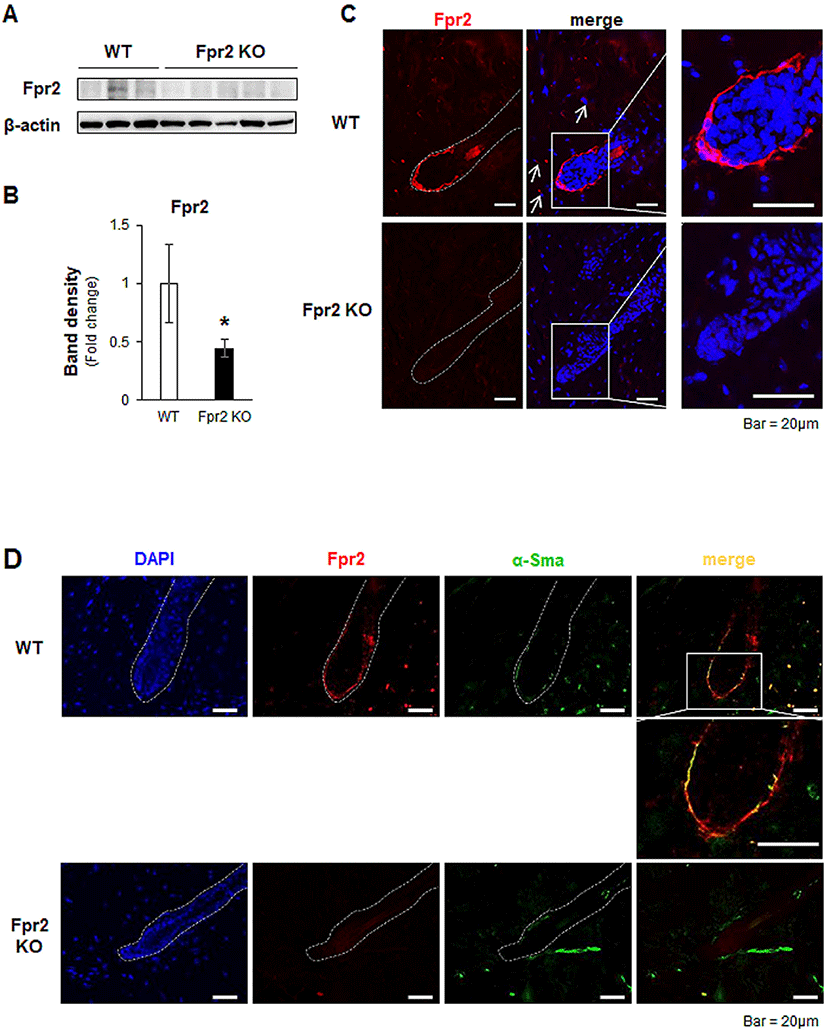
After HF morphogenesis, HFs undergo cycles of growth (anagen), regression (catagen), and rest (telogen) to produce new hairs for life (Schneider et al., 2009). The progression of the hair regeneration cycle is modulated by several signaling factors, such as SHH, Wnt/β-catenin and BMPs (Botchkarev et al., 2002; Zhang et al., 2006; Rishikaysh et al., 2014; Avigad Laron et al., 2018). Expression of Shh in adjacent DPCs and activation of Wnt/β-catenin signaling in HFs are necessary in the transition from the telogen to the anagen phase during hair regeneration (St-Jacques et al., 1998; Chiang et al., 1999; Cui et al., 2011). Deletion of these signaling molecules has been shown to result in dramatic hair shortening and/or loss (Cui et al., 2011). Given that Fpr2 KO mice showed hair loss after normal morphogenesis, we hypothesized that a lack of Fpr2 induced an alteration in these signaling molecules, and dysregulated the hair regeneration cycle. To prove our hypothesis, we evaluated Shh and β-catenin expression in the dorsal skin, including the HFs. The levels of Shh and β-catenin in the skin were significantly lower in the Fpr2 KO mice than in the WT mice (Fig. 3A, B). Shh (red colored) was expressed in DPC-like cells in the lower part of the HFs of the WT mice, whereas these cells were rarely detected in the Fpr2 KO mice, as assessed by immunofluorescence staining (Fig. 3C, left panel). Nuclear β-catenin, which indicates the activation of Wnt signaling, was apparent in the WT mice. However, in the Fpr2 KO mice, β-catenin was restricted to the cytoplasm (Fig. 3C, right panel).
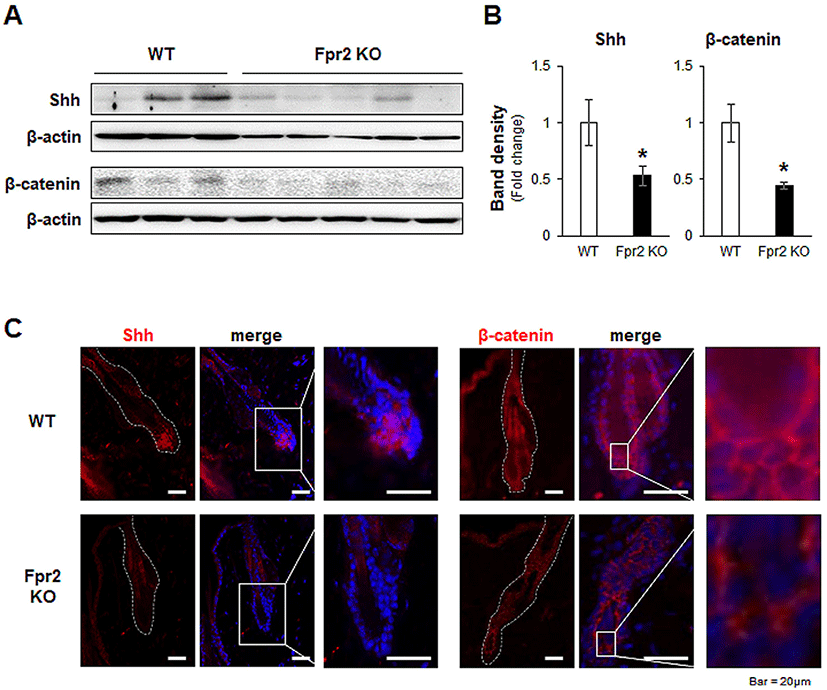
In the regulation of the hair regeneration cycle, BMP2 and BMP4 are critical, as they inhibit hair regeneration by counteracting Shh and Wnt/β-catenin (Genander et al., 2014). Their expression levels were low in the anagen phase and high in the telogen phase (Plikus et al., 2009). In the Fpr2 KO mice, Bmp2 and Bmp4 were significantly elevated compared to levels in the WT mice (Fig. 4A, B). Furthermore, Bmp2-positive cells were more obviously observed in the immunofluorescence staining of the Fpr2 KO mice, whereas these cells were hardly detected in the WT mice (Fig. 4C, left panel). In addition, Bmp4-expressing cells were more evident in the Fpr2 KO mice than in the WT mice, and these cells were observed in fibroblast-like cells located in the extrafollicular dermis (Fig. 4C, right panel). Taken together, these findings suggest that Fpr2 ablation arrests the hair regeneration cycle in the telogen phase by decreasing Shh and β-catenin and increasing Bmp2/4 expression.
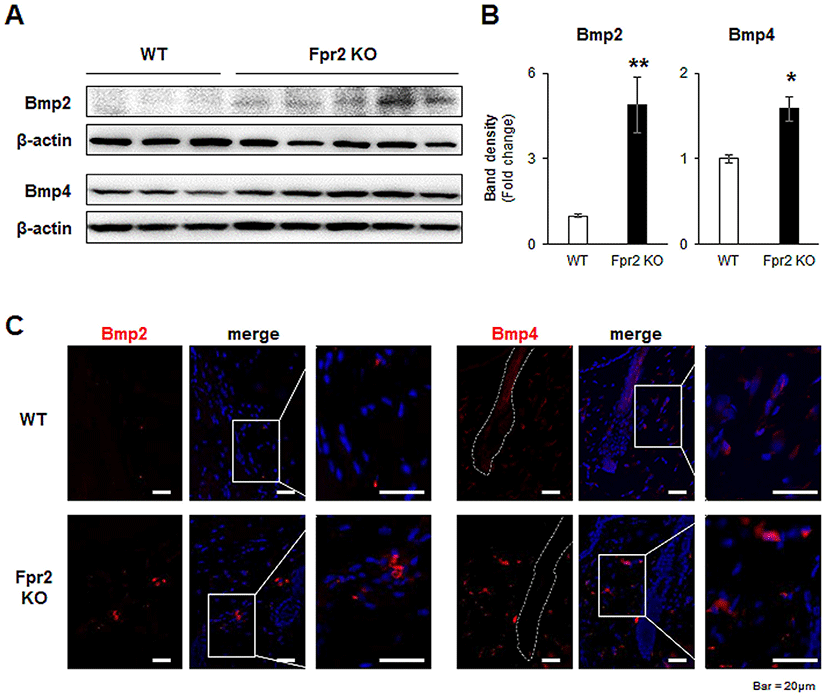
During hair regeneration, HFSCs proliferate and differentiate into HF components, and DPCs, which are now considered a reservoir of multipotent stem cells, regulate HF development and growth (Rahmani et al., 2014; Ji et al., 2021). HFSCs and DPCs undergo rapid proliferation to fuel the initial stage of hair growth that supports the prolonged growth of the hair (Rahmani et al., 2014; Ji et al., 2021). Based on the inhibited hair regeneration observed in the Fpr2 KO mice, we examined the activation of HFSCs and DPCs by assessing the specific activation markers for each cell type.
Keratin 15 (K15) is a stem cell marker of HFs, and K15-positive HFSCs preferentially proliferate during the anagen phase (Bose et al., 2013). The level of K15 was significantly downregulated in the Fpr2 KO mice compared with the WT mice (Fig. 5A, B). Immunofluorescence staining for K15 also showed that K15-positive HFSCs were present in the WT mice, but absent from the Fpr2 KO mice (Fig. 5C, left panel).
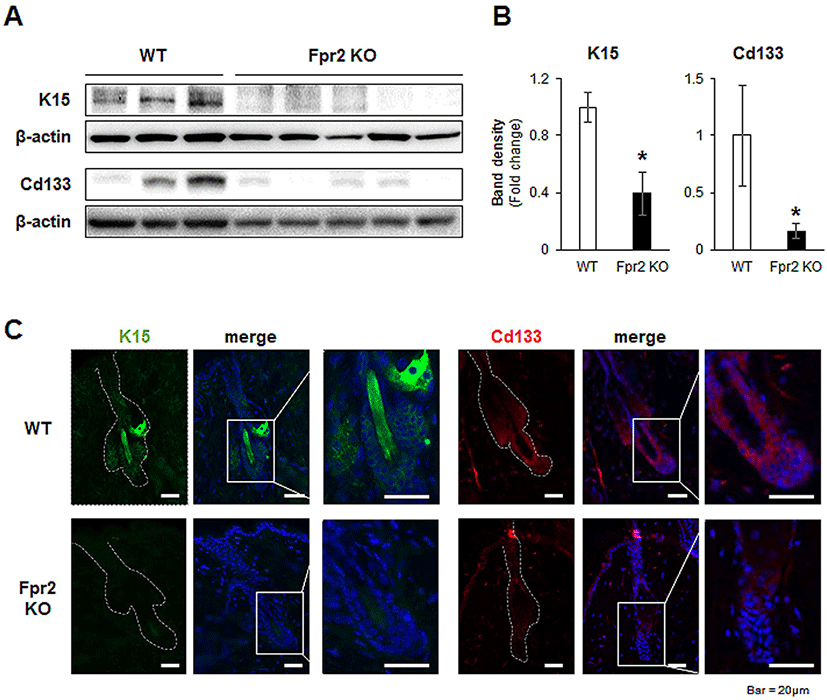
CD133 is expressed by a subpopulation of DPCs during the early stages of the anagen phase (Ito et al., 2007). Expression of CD133 was significantly lower in the dorsal skin tissue of Fpr2 KO mice than in WT mice (Fig. 5A, B). WT mice were found to have CD133-expressing DPC-like cells located in the lowest part of the HF, whereas these cells were not detected in Fpr2 KO mice (Fig. 5C, right panel). These data indicate that FPR2 is involved in the proliferation of HFSCs and DPCs, thereby impacting hair regeneration.
DISCUSSION
Hair loss occurs across all ages, sexes, and ethnicities (Strazzulla et al., 2018). There are no radical therapies that prevent or reverse hair loss. Despite many studies being conducted to elucidate the mechanism underlying hair loss, it remains a poorly understood condition. This limited understanding of hair loss hinders the development of applicable therapies for all types of hair loss. Therefore, it is necessary to discover novel mediators of hair loss. This study revealed the effect of FPR2 on hair regeneration using an Fpr2 KO mice model. Fpr2 KO mice displayed postnatal hair loss, and we observed obvious histological differences between the WT and Fpr2 KO mice. A thickened hypodermal adipocyte layer and dystrophic HFs with distorted shapes, cyst-like structures, and excessive accumulations of epithelial cells were apparent in the Fpr2 KO mice. To determine the role of Fpr2 in this HF impairment, we first examined Fpr2 expression in the dorsal skin tissue of mice, and revealed that Fpr2 was expressed by dermal fibroblasts in the outer layer of the surrounding lower parts in the HF. Dermal fibroblasts play a critical role in skin structure and integrity by synthesizing the extracellular matrix that supports the skin structure (Thangapazham et al., 2014). In addition, dermal fibroblasts were shown to have an inductive effect on HF neogenesis by promoting the activity of DPCs and HFSCs (le Riche et al., 2019). Given the actions of dermal fibroblasts in inducing hair regeneration, FPR2 expression in dermal fibroblasts suggests that FPR2 has the potential to regulate hair regeneration by influencing the activation of DPCs and HFSCs.
Hair regeneration is mediated by a cycle of structural changes (Stenn & Paus, 2001; Schneider et al., 2009). Hence, the activation and stability of the hair regeneration cycle are key factors in achieving an intact HF. However, HF loss is often accompanied by the termination and/or dysregulation of the hair regeneration cycle, such as a shortened anagen phase or a failed transition from the telogen to anagen phase (Ji et al., 2021). The activity of HFSCs and DPCs is the main driving force for the continuous hair regeneration cycle and is regulated by various signaling molecules (Rahmani et al., 2014; Chen et al., 2020a). Among these signaling molecules, SHH, Wnt/β-catenin, and BMP are the most prominent in hair regeneration (Rishikaysh et al., 2014). SHH accelerates HF growth by inducing the activation of HFSCs and DPCs (St-Jacques et al., 1998; Lim et al., 2018). After the anagen phase is initiated, the increased production of SHH promotes HFSC proliferation and DPC maturation (St-Jacques et al., 1998; Lim et al., 2018). Cui et al. reported that skin-specific Shh-depleted mice had total hair loss over their entire bodies (Cui et al., 2011). Ubiquitous Shh KO mice were unable to develop HFs during development despite having hair germs (Chiang et al., 1999). It was also shown that the administration of recombinant human SHH protein increased hair density and hair length in mice (Yu et al., 2019). Shh stimulates hair regeneration by modulating Wnt/β-catenin (Avigad Laron et al., 2018). Laron et al. revealed that Shh signaling in the DPCs fine-tunes the activation of Wnt/β-catenin signaling that results in the proliferation of HFSCs for hair regeneration (Avigad Laron et al., 2018). Wnt/β-catenin signaling is also implicated in the development of skin, hair, and related appendages (Millar et al., 1999). β-catenin is known to be essential for HFSC activation, via its collaboration with Lymphoid enhancer-binding factor-1 (LEF-1), and it controls their growth (van Genderen et al., 1994; Huelsken et al., 2001). Huelsken et al. demonstrated that β-catenin deletion led to subsequent hair loss and small cystic HFs after normal morphogenesis (Huelsken et al., 2001). In line with these findings, we found that the levels of Shh and β-catenin in HFs were remarkably lower in Fpr2 KO mice with extensive hair loss and small cystic HFs than WT mice (Fig. 1–3). In addition, expression of Bmp2 and Bmp4, which block anagen induction, increased in Fpr2 KO mice compared with WT mice (Fig. 4). Plikus et al. reported that Bmp2 and Bmp4 were produced by extra-follicular sources (Plikus et al., 2008). Given that most BMP2 is produced by hypodermis adipocytes, the thickened hypodermis adipocyte layer in the Fpr2 KO mice seems to be related to the increased expression of Bmp2 (Plikus et al., 2008).
In conclusion, we have demonstrated that the signaling molecules regulating the activity of HFSCs and DPCs are dysregulated in Fpr2 KO mice, and that hair regeneration is impaired, resulting in hair loss after morphogenesis. Although the mechanism by which FPR2 interacts with the signaling molecules in pathogenic hair loss remains unclear, our results indicate that FPR2 is a key modulator of HFs and has therapeutic potential for the prevention and/or treatment of hair loss.