INTRODUCTION
Humanized mouse model is defined as immunodeficient mice engrafting functional human cells and tissues (Walsh et al., 2017). Generally, the humanized mouse model is reconstituted with human blood derived CD34+ stem cells from cord blood (CB), bone marrow (BM), and peripheral blood (PB) (Mosier et al., 1988; Lapidot et al., 1992; Shultz et al., 1995). Owing to continuous development of humanized mouse model, investigators have been able to study for immune response between human immune cells constructed from stem cells and therapeutic cell sources in vivo. Recipient mice are used as surrogates of human biology and diverse pathologic condition such as leukemia, breast cancer, autoimmune disease, and regenerative medicine (Lee et al., 2015). However, the mouse environment is incongruent with that seen in humans, for example, de novo drugs and human pathogens are species-specific and innate immune system (Walsh et al., 2017), thus, the humanized mouse model is considered as an important tool for biomedical research. In particular, it is an emerging as an in vivo platform to screen immune responses. Irradiated immunodeficient mice that receive human stem cells via intravenous injection and CD34+ stem cells migrated and engrafted into the BM, and subsequently circulated into PB after hematopoiesis. In order to model human immune systems, investigators first have been studying immunodeficient mice using engrafted human cells from hematopoietic stem cells (HSCs) (McCune, 1988; Shultz et al., 2012). Additionally, humanized mice can facilitate studies on immune response caused by human leukocyte antigen (HLA) mismatching and on human diseases such as cancer (Henderson, 1994; Hu, 2016; Morton, 2016; Serr et al., 2016; Luce et al., 2018). A humanized model is required to study immune responses in vivo because results of in vitro culture conditions cannot properly represent immune crosstalk. Despite this requirement, humanized mouse models have limitations in terms of reconstruction of the hemato-lymphoid system due to insufficient availability of human epitopes. Although humanized mice maintain cross-reactivity by supporting the tolerance of the mouse cells towards transplanted human thymic tissues, regenerated T cells do not show adequate development for maturation with the HLA, which results in the failure of T cell activation (Obenaus, 2015; Kooreman et al., 2017). First, Ito M et al. and Shultz LD et al. developed advanced Nod scid gamma (NSG) (IL2rgnull, NOD/ShiLtSz-scid/IL2Rgnull(NOD. Cg-PrkdcscidIl2rgtm1Wjl/SzJ) mice, with a mutation in the IL-2Rr chain, which optimally support human T cell development (Ito et al., 2002; Shultz et al., 2005). Development of non-obese, diabetic mice with the Nod-scid gamma mutation was an advancement compared to the NSG mice developed previously. This proved to be a breakthrough in the development of humanized mouse models. This strain is more suitable for the generation of human T cells, as compared to the previous mouse strains such as C.Cg-R ag2 t mFwa I l2rg t m Sug and C; 129S4- Rag2 t mFlv I l2rgt m 1Flv/J. (Traggiai et al., 2004; Song et al., 2010). All strains showed enhanced engraftment of human HSCs, long life span, and production of functional immune responses, but, their efficacy was low. It was difficult to evaluate the crosstalk between lymphoid cells and pathogens. In this review, we summarize basic T cell development in the thymus of humanized mice, and suggest a solution to generate functional human T cells in a humanized mouse model. Also, we briefly review the achievements, recent trends, and the challenges in development of the humanized mouse model, which closely mimics human physiological environments in terms of hematology, immunology, and regenerative medicine.
T CELL DEVELOPMENT IN HUMANIZED MICE
Since Strowig T et al. showed that cytotoxic and cytokine releasing T cells can target latent Epstein-Barr virus (EBV) antigens using HLA-A2 transgenic mice (Strowig et al., 2009), Leonard D. Shultz et al. also reported the development of a homozygous major histocompatibility complex-I (MHC-I) expressing humanized mouse model, and demonstrated the functionality of cytotoxic human T cells (CTLs) in EBV infected humanized mice (Shultz et al., 2010). Many approaches using MHC mutations have allowed the establishment of HLA restricted humanized mouse models to study human immune systems (Watanabe et al., 2009; Jaiswal et al., 2012; Billerbeck et al., 2013; Thomas S, 2014). In the early stages of development of a humanized mouse model for T cell development, disease causing viruses, such as the EBV and the dengue virus that infect only humans, were mainly used to induce functional lymphocytes. Major or minor histocompatibility complexes including HLA-A2 and HLA-DR4 are common targets to induce genetic defects in a humanized mouse model. Danner R et al. reported the importance of HLA class II molecules in developing human lymphocytes, in a humanized mouse model (Danner, 2011). Several studies have consistently reported generation of HLA-DR restricted T cells with enhanced immunity in humanized mouse models (Suzuki, 2012; Billerbeck et al., 2013; Masse-Ranson et al., 2019). Although development of functional T cells in humanized mouse models has shown advancement in comparison to previous experiments, the production of T cells stimulated by human epitopes failed in xenograft models. To overcome this issue, amp-peptide with poly I:C, artificial mimetopes, and human viruses were directly injected into humanized mice (Serr et al., 2016; Coleman et al., 2018; Kruse, 2018). Also, the tumor associated antigen, Wilms tumor 1 (WT1), was recognized by human CD8 T cells in NSG mice (Najima et al., 2016). It was the first study to show that human mature T cells recognizing specific antigens can be generated in the humanized mouse model without genetic modification. These approaches have partly enabled us to reveal details of the dynamics of T cell reconstitution in humanized mice. To effectively investigate the crosstalk between immune cells and pluripotent stem cell derived cells in vivo, functional lymphocytes, which show HLA-restricted cytotoxicity, can be used. Although these trials have continuously enhanced cancer antigen specific T cells as well as HLA restricted T cells for regenerative medicine, the development of long-term hemato-lymphoid cells, including cytotoxic T lymphocytes (CTLs), in humanized mice is still in its infancy due to low alloreactivity (Andre, 2010; Kooreman et al., 2017). In vivo experiments for immune rejection by HLA types are considered crucial for the verification of stem cell derived therapeutic source safety in regenerative medicine. Because of obstacles related to in vitro studies in screening omnidirectional dynamics of immune responses, development of in vivo platforms such as humanized mouse models is essential. So far, there has been considerable advancement in the development of humanized mouse models and we propose the importance of HLA mis- and matched cells as a novel strategy to stimulate thymocyte development in advanced humanized mouse models, given the effective usage of HLA mis- and matched cells in stimulating the CD4+CD8+ thymocytes (Fig. 1). We provide a simplified table with explanation for pros and cons of humanized mouse model by cell sources (Table 1).
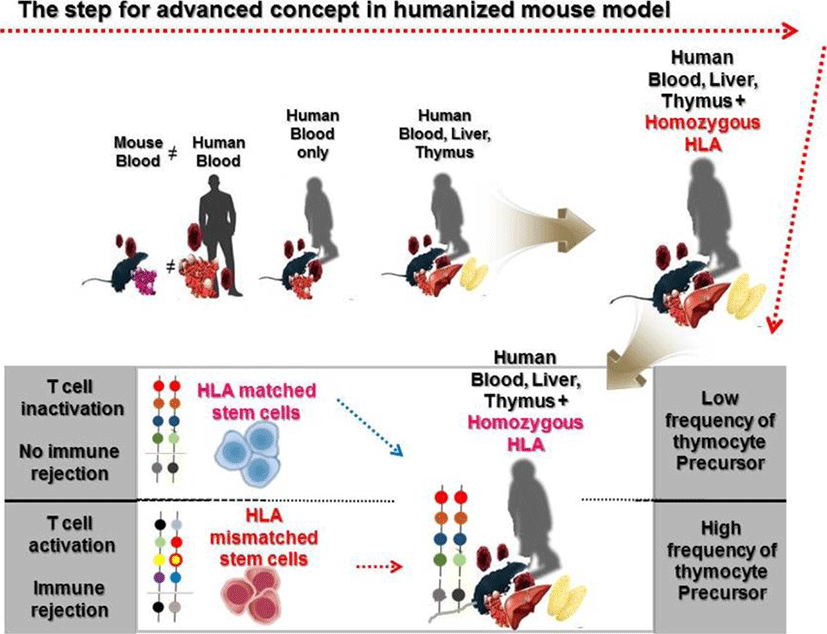
Cell sources | Pro | Con | Ref. | |
---|---|---|---|---|
PBL | Human PB lymphocyte | 1. Easy to establish 2. GVHD model | 1. Inability of human LT-HSC | Mosier et al., 1988; Tary-Lehmann et al., 1995 |
2. Good model for xenogeneic GVHD | 2. Anergic naïve and memory T cells | Gleichmann et al., 1984; Garcia et al., 1997; Tary-Lehmann and Saxon, 1992 | ||
3. Initiation to generate lymphoid cells | 3. The lacks of functional human lymphoid cells | Tsuchida et al., 1997; Dessureault et al., 1997; Tary-Lehmann and Saxon, 1992 | ||
4. Uncontrolled B cell activation | Williams et al., 1992; Abedi et al., 1992; Gleichmann et al., 1984 | |||
SRC | Hematopoietic stem cells from a variety of sources | 1. Establishment of LT-HSC | 1. The lacks of educated human T cell | Hiramatsu et al., 2003; Ishikawa et al., 2005; Melkus et al., 2006 |
2. Generation of human immune system | 2. Restriction system for genetic traits | Cany et al., 2013; McCune et al., 1988; Smith et al., 2016 | ||
3. High engraftment of NK cells and CD3 lymphoid cells | 3. Uncontrolled or spontaneously generation of B cells without maturation | Manz, 2007; Melkus et al., 2006 | ||
BLT | Human BM, liver, thymus | 1. Environmental mimicking and complete human immune system | 1. Inadequate for all HLA types | McCune et al., 1988; Wege et al., 2008; Smith et al., 2016; Melkus et al., 2006 |
2. Generation of HLA restricted T cells | 2. Requirement of human epitopes for high diversity | Shultz et al., 2010; Takaki et al., 2006; Niens et al., 2011; Ishikawa et al., 2005 | ||
3. Human mucosal immune system completion | 3. The developmental failure of GVHD | McCune et al., 1988; Lan et al., 2006; Karpel et al., 2015; Lavender et al., 2013 |
PBL, peripheral blood lymphocyte; PB, peripheral blood; GVHD, graft-versus host disease; LT-HSC, long term hematopoietic stem cell; SRC, scid repopulating cells; NK, natural killer; BLT, bone marrow liver thymus; BM, bone marrow, HLA, human leukocyte antigen.
GENERAL DEVELOPMENT OF T LYMPHOCYTES AND INEFFICIENT ENVIRONMENT OF THYMUS IN HUMANIZED MICE
In a humanized mouse model, blood cell reconstitution from adult HSCs was already confirmed in various conditions (Traggiai et al., 2004). HSCs are derived from human origins such as cord blood, bone marrow, and peripheral blood, but, thymic epithelial cells for human cell generation in a humanized mouse model are of mouse origin. Secondary lymphoid tissues (SLT) such as the thymus and lymph nodes are essential to completely mimic the human immune system in the mouse model (Takahama, 2006; Li et al., 2018). Amid these, human thymic epithelial cells, which express MHC molecules, have an affinity for CD4+ and CD8+ T cells and play a role in the recognition, maturation, and development of lymphocytes (Li et al., 2005; Danner, 2011). Mouse has thymic epithelial cells with mouse MHC class molecules, such as H-2K, H-2D, H-2L, I-A, and I-E, which affect the development of T cells. Because these molecules are not presented in a similar sequence as that of human MHC molecules, mouse thymic epithelial cells are fundamentally limited in mimicking human organs. However, mouse thymic epithelial cells are fundamentally limited in mimicking human organs, because mouse MHC molecules are not presented in a similar sequence as that of human HLA molecules. Lympho-stromal interactions in the thymus play a pivotal role in T cell development and maturation based on the expression of T cell receptors (TCRs) during negative selection. The mouse thymic environment can partly provide specified cytokines required for the development of human T cells. However, the mouse MHC class is limited for the development of specific human HLA molecules, which ultimately results in failure of T cell development. These T cells are often biased and it failed to successfully present active human cell phenotypes, expressing CD45RO due to insufficiency of human epitopes (Kooreman et al., 2017). T cells are derived from HSCs, which can differentiate into various lineages of blood cells. In the postnatal stage, HSCs are first generated in the bone marrow, and then migrate into the thymus via peripheral blood, and begin to develop into T cells (Lambolez, 2006; Takahama, 2006). The progenitors of T cells within the thymus are known as thymocytes, which undergo several steps of maturation. The degree of maturation of thymocytes can be identified in terms of expression of some cell surface markers. Early T lymphocyte progenitors in the cortex of the thymus are defined as double negative (DN) thymocytes, which do not express CD4 and CD8 co-receptors (Punt JA, 1996). These DN cells with the CD44+CD25+ phenotype in the cortex can further differentiate into CD4 and CD8 double positive (DP) cells via TCR beta-selection (Prinz et al., 2006; Fayard et al., 2010). As selected cells have a stably rearranged TCR-β chain locus, these cells successfully acquire CD3 molecules, and express the CD4 and CD8 co-receptors (Fehling, 1995; Bruno, 1996). In contrast, cells which cannot undergo beta-selection die via apoptosis. T cells that have survived beta-selection rearrange their TCR-alpha chain loci to produce an αβ-TCR (Chan, 1993; Davis et al., 1993). Re-arrangement of TCRα locus has been conducted; T cells expressing the full TCR as well as CD4+CD8+ cells are termed ‘double-positive’ (DP) cells. DP T cells are capable of interacting with MHC molecules. This involves the signal by double-positive precursors that express either MHC Class I or II restricted receptors. DP cells selected on MHC class I and II will become CD8+ and CD4+ cells, respectively. The cells will become single-positive, either CD4+ or CD8+ T cells (SP cells), after downregulation of the non-selected co-receptors. SP cells then undergo negative selection, which is defined as tolerance of self by elimination of T cells binding with self MHC molecules in the medulla region. T lymphocytes become naïve CD4 or CD8 cells which express CD45RA via negative selection step and then enter peripheral circulation (Fig. 2, left panel). Since T cells which have completely accomplished their development in thymus have functional acquirements in the peripheral circulation, negative selection in humanized mouse models must therefore be performed entirely by the human MHC molecules. To acquire the immune susceptibilities with high diversity for human cells, education of T cells by repetitively injecting mismatched human cells, as well as by transplantation of the human bone marrow, liver, thymus (BLT) transplantation. NSG mouse for humanized model partially supported the maturation of T and B cells (Peault et al., 1991; Ishikawa et al., 2005; Brainard et al., 2009); but thymus of NSG mice do not express HLA molecules (Thomas et al., 2014). Thus, NSG humanized mouse with T cell development lacks the capability to recognize human antigens in an HLA-restricted manner. To overcome the technical limitation, Shultz et al. developed the NSG-HLA-A2/HHD strain expressing HLA class I and showed the functional HLA-restricted cytotoxic T cells in the EBV infection, which is restricted HLA-A2 antigen. This indicates the recognition and education of human HLA in functional thymus of NSG (Shultz et al., 2010). Differentiation of T cells in NSG-HLA-A2/HHD mouse recapitulates the development of normal human T cells in vivo. The human T progenitors as well as humanized microenvironment are required to educate human T cells. From NSG-HLA-A2/HHD mouse, advanced NSG mouse expressing the HLA class I molecules including HLA-A*0201 and A*2402 was established and developed by injection of human specific antigen WT1 peptides (Najima et al., 2016). This humanized mouse model led us to an advanced platform to study for immune stress response in terms of HLA mis/matched therapeutic stem cells and human viruses such as hepatotropic virus (Billerbeck et al., 2013). Although it is commonly believed that mouse thymus is not enough to entirely develop human T cells, the human mimicking signals, critical factor DLL4, and chemokines including Ccl25 and Cxcl12 may directly work to improve the thymic function and support diverse TCR repertoire (Zakrzewski et al., 2006; Halkias et al., 2013; Velardi et al., 2014). However, because mouse thymic environment for negative selection of human cells is insufficient, regulatory T cells (Treg cells) fail to functionally develop in NSG mice (Halkias et al., 2015); implying that T cell development still remains immature. To compensate thymic environment from humans, BLT seeding was applied in the humanized mouse model (Peault et al., 1991; Covassin et al., 2013; Brugman et al., 2015; Chung et al., 2015; Tuckett et al., 2017). Although BLT transplantation showed that donor specific HLA molecule is confirmatively augmented in medullary lymphocytes, lymphocytes are rapidly saturated in terms of transplanted cell numbers, suggesting the vitality of thymic epithelium rather than high HSCT cell numbers in T cell development. A brief schematic diagram for T cell development in the thymus is shown in Fig. 2 (right panel).
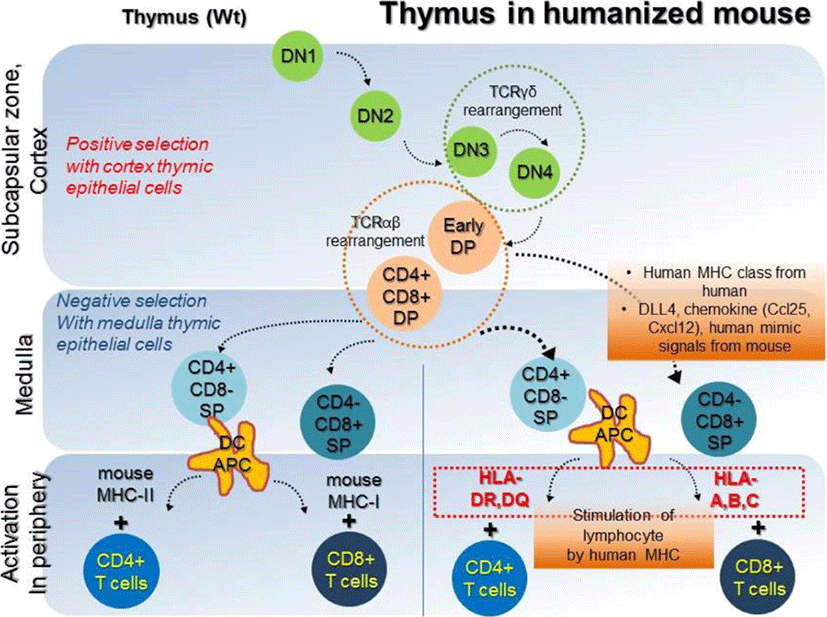
STRATEGIES TO STIMULATE EFFECTOR/MEMORY T CELLS IN PERIPHERAL CIRCULATION
CD3+ T cell responses are initiated in peripheral lymphoid organs by activated antigen presenting cells (APCs) such as dendritic cells and macrophages (Steinman, 1991; Ridge, 1998). Antigens need to migrate to lymphoid tissues via blood, lymph, and APCs. APCs ultimately provide antigen epitopes, also known as signal I, co-stimulatory cytokines such as CD28, CD80/CD86, CD40/CD40L, known as signal II, and cytokines including IFN-gamma, IL-12 and IL-4, known as signal III to naïve T cells (Fitzpatrick et al., 1996; Lenschow et al., 1996; Schoenberger, 1998; Murphy & Reiner, 2002). Three factors are required to stimulate human CD3+ T cells. After binding of MHC molecules with antigens to TCRs on T cells, T cells get activated, differentiate, and expand into effector T cells. The roles of effector T cells in cell- and humoral-mediated immune responses have dynamic effects. Cytotoxicity against pathogens by CD8+ T cells, induction of antibody production in B cells by CD4+ helper T (Th1) cells, and suppression of regulatory T cells (Tregs) to enhance T cell function are mainly regulated by mature immune system. To investigate the immune stress response of CD3+ T cells in a humanized mouse model, development of immunity should be preceded by development of the thymus and lymphocyte development mediated by human MHC molecules. Melkus MW et al. first used superantigen toxic shock syndrome toxin 1 (TSST-1) recognizing dendritic cells in a humanized mouse model to reconstitute human T cells, suggesting that human antigens directly stimulate human T cells in humanized mice (Melkus et al., 2006). Based on this, Lan P et al. first reported successful establishment of a BLT humanized mouse model, wherein they transplanted human tissues including bone marrow derived blood cells, thymic epithelial cells and liver cells (McCune et al., 1991; Lan et al., 2006). An important achievement due to the BLT tissue transplant model as compared to stem CD34+ cells only transplant model, is the ability of engrafted mice to show high antiviral immune responses in vivo (Traggiai et al., 2004; Dudek et al., 2012; Klenerman & Oxenius, 2016). However, this model does not allow long-term survival of humanized mice, and results in a failure of long-term immune response in vivo. Additionally, a long duration is required to achieve significant peripheral human effector T-cell repopulation in humanized mice. Most importantly, such mice show tolerance to human T cells along with lack of HLA restriction. However, the success of the model still remains undefined due to lack of fully stimulation by human HLA antigens. Recently, Kooreman NG et al. emphasized the necessity of allogenized mice, not that of humanized mice, to model the human immune system via allogeneic human embryonic stem cells (hESCs), implying that the humanized status without effector T cells is not completely adequate to achieve T lymphocyte activation to investigate immune rejection by HLA types (Kooreman et al., 2017). For an effective response, HLA molecules and anti-CD3/CD28 antibodies are required as specific ligands for activation of human T lymphocytes, which leads to an allogenized status. Alternatively, reconstruction of TAP-LAP was used to screen immunity by HLA alleles in humanized model. This transgenic mouse model enabled HLA-A molecules to be transported into endoplasmic reticulum, results in increased stability of humanized status in mouse (Huang et al., 2016). Tobias D et al also reported that generation of transgene expressing hypoimmunogenic cells, which modified with depletion of B2M, CIITA and overexpression of CD47, from induced pluripotent stem cells (iPSCs). They first carried out Elispot assay to address hypoimmunogenic properties of their modified iPSCs with splenocytes of humanized mouse model. This is the first evidence for HLA alleles of human cells in humanized mouse model (Deuse et al., 2019). A CD45RO–CD45RA+CCR7+ phenotype is a typical marker of naïve T cells. Expression of CD45RO+CD45RA–CCR7+ from human CD8+ cells (TCM), is widely considered to be a marker of central memory T cells. In contrast, the CD45RO+CD45RA–CCR7– phenotype is regarded as a marker for T effector memory (TEM) cells, which function as cytotoxic T cells. In particular, CD27 and CD28 are not expressed in TEM cells (Lanzavecchia & Sallusto, 2000; Romero et al., 2007). Thus, optimized lymphoid reconstruction should allow verification of expression of the mature effector CD45RO+CD45RA–(CCR7+/ CCR7–) phenotype in T cells of the humanized mouse model for the alloimmune response of pluripotent stem cell derived cells.
Conclusion
Development of a humanized mouse model faces several barriers. Suppression of mouse immunity is required to effectively generate human lymphoid cells. Also, expression of HLA alleles is required for the development of human lymphoid immunity. Human specific factors and epitopes, which are not found in mice, must be used for functional lymphocyte development. In addition, reconstitution of a long-term lymphoid cell lineage is possible by the use of human cells. It will be important to investigate whether pluripotent derived stem cells can alter immune rejection responses, in terms of the HLA mis- or matched status, and if a humanized model presents an allogenized status to human HLA molecules. An advanced humanized mouse model will contribute to the analysis of the machinery underlying immunological interactions and recapitulating the humanized mouse model with effector T cells will provide insights for the development of therapeutic strategies.