INTRODUCTION
Nonylphenol (NP) is a member of alkylphenol ethoxylates and widely used in the industrial field as additives of lubricating oil, plasticizers of plastics and antioxidants (USEPA, 1990). Since the original studies that NP has the estrogenic activity, many previous studies demonstrated and suggested that NP is an endocrine disrupting chemical (EDC) and can interfere with reproductive system in mammals inducing changes in gonadal function (Nagao et al., 2001; Gong & Han, 2006; Su et al., 2018; Urriola-Muñoz et al., 2018). Furthermore, the reproductive toxicities of NP were also observed in second generation of rats exposed to in utero (Chapin et al., 1999).
Meanwhile, the current EDC research subjects have been expanded to include neurotoxicity, immunotoxicity, and metabolic toxicity in addition to reproductive toxicity (Gore et al., 2015). For example, it has been reported that NP exposure could induce adverse effects in brain (Lotfi et al., 2021), spleen (Fu et al., 2022), and pancreas (Yu et al., 2018). In addition, it was found that NP has an endocrine disrupting effect in the adrenal gland, such as synthesis and secretion of steroid hormones, as in the gonads (Chang et al., 2010, 2012). Based on these findings, we hypothesized that the changes in adrenal endocrine function of NP–treated animals were due to the structural changes of the gland. To prove this, the present study was conducted using a low-dose NP administration model in advance (Kim et al., 2020; Lee et al., 2021), and histological analyses of adrenal substructure were performed.
MATERIALS AND METHODS
Sprague-Dawley rats were provided by DBL (Eumseong, Korea) and reared in Sangmyung University animal facility under photoperiods of 12 h light/dark with lights on at 7 AM and constant temperature of 21°C–23°C. Food and tap water were supplied ad libitum. The animal protocols were approved by the Animal Care and Use Committees at Sangmyung University (2018-03; approval number R-1801-1). All the animals received human care in accordance with the guides for animal experiments of the Association for Assessment and Accreditation of Laboratory Animal Care (AAALAC) International.
In this study, we conducted in vivo and in vitro study. For in vivo study, 8 weeks-old male and female rats were purchased from DBL. After acclimation, breeding pairs of rats were divided into 3 groups; (1) Control (CTL), supplied tap water, (2) NP-50, treated with low-dose NP (50 μg/L; Sigma-Aldrich, St. Louis, MO, USA), (3) NP-5000, treated with high-dose NP (5,000 μg/L) via drinking water. The parental (P) generation rats had pre-mating period for 2 weeks. After pre-mating period, female and male rats were placed in the breeding cage until a vaginal plug of female rats was observed. The rats were treated with NP from pre-mating period to weaning of offsprings (F1). The F1 generation was weaned at postnatal day (PND) 21 and consistently treated with the NP until sacrifice. F1 females and males were sacrificed at PND 56. All sacrifices and sample collection were done between 18:00-19:00 pm. After weighing, and the tissues were fixed in 4% paraformaldehyde (Bio PURE, Havant, UK) and stored at 4°C overnight.
Fixed tissues were dehydrated in graded concentrations of ethanol (70%, 80%, 90%, 95%, and 100%; Duksan, Ansan, Korea) for 1 hour 30 minutes in each with gentle shaking and soaked in absolute ethanol overnight. The tissues were immersed in xylene (Samchun Chemical, Seoul, Korea) for 30 minutes, 3 times and in paraffin (Avantik Biogroup, Springfield, NJ, USA) at 56°C for 30 minutes, 3 times. The tissues were embedded in paraffin and sectioned (Microm, Walldorf, Germany) at 5 μm. The samples were attached on microscope slides (Paul Marienfeld GmbH & Co. KG, Lauda-Königshofen, Germany) and the slides were stained with hematoxylin (Sigma-Aldrich) and eosin (Across, Carson, CA, USA) for 5 minutes, respectively.
RESULTS
The terminal body weight of F1 female rats was measured at PND 38. The terminal body weight of NP-50 group was significantly increased (Table 1; p<0.05), however, that of NP-5000 group was significantly decreased (p<0.001). The kidney weight of NP-5000 group was significantly decreased (p<0.001) and ratio of kidney weight to body weight (i.e., relative weight) in NP-5000 was also decreased (p<0.001). The adrenal weight of NP-5000 group was significantly declined (p<0.01), however, ratios of adrenal weights to body weights were not significantly changed (Table 2; NP-50, p<0.01; NP-5000, p<0.05).
Values are shown as mean±SE.
Parents rats were supplied with a tap water or NP from pre-mating to offspring birth. The F1 generation rats were also treated with NP until sacrifice at postnatal day (PND) 38. The terminal body weights were measured at sacrifice. The organ weights were also recorded and organ to terminal body weight ratios were then calculated. All experiments were performed at least three times.
Significant differences are marked with asterisks (* p<0.05, ** p<0.01, *** p<0.001; compared with the CTL group).
CTL, control group; NP-50, group of rats treated with 50 μg/L nonylphenol; NP-5000, group of rats treated with 5,000 μg/L nonyl-phenol.
Values are shown as mean±SE.
Parents rats were supplied with a tap water or NP from pre-mating to offspring birth. The F1 generation rats were also treated with NP until sacrifice. The parameters were measured by ImageJ software. All experiments were performed at least three times.
Significant differences are marked with asterisks (* p<0.05, ** p<0.01, *** p<0.001; compared with the CTL group).
CTL, control group; NP-50, group of rats treated with 50 μg/L nonylphenol; NP-5000, group of rats treated with 5,000 μg/L nonyl-phenol.
The terminal body weight of F1 male rats was measured at PND 56. Unlike females, the terminal body weights of male rats were not shown any significant difference (Table 3). The kidney weight of NP-5000 group was significantly lighter than that of CTL group (p<0.01) and ratios of kidney weights to body weights of high-dose NP-treated groups were also significantly declined (p<0.001). Unlike female kidney, ratios of kidney weights to body weights of low-dose NP-treated groups were significantly decreased (p<0.001). In contrast, the adrenal weights of NP-treated groups were not significantly changed compared to controls.
Values are shown as mean±SE.
Parents rats were supplied with a tap water or NP from pre-mating to offspring birth. The F1 generation rats were also treated with NP until sacrifice at postnatal day (PND) 56. The terminal body weights were measured at sacrifice. The organ weights were also recorded and organ to body weight ratios were then calculated. All experiments were performed at least three times. Significant differences are marked with asterisks (** p<0.01, *** p<0.001; compared with the CTL group).
CTL, control group; NP-50, group of rats treated with 50 μg/L nonylphenol; NP-5000, group of rats treated with 5,000 μg/L nonyl-phenol.
The adrenal glands of F1 female and male rats were sectioned and stained with hematoxylin and eosin (Fig. 1). The thickness of zona glomerulosa (zG) layers of female rats in NP-5000 group was significantly declined (Table 2; p<0.001) but zona fasciculata (zF) layers were not changed. The zona reticularis (zR) layers of NP-treated group were significantly thinner than those of CTL group (NP-50, p<0.05; NP-5000, p<0.01). In male adrenal glands, there was no significant change of zG layers in NP-treated groups while the thickness of zF in NP-5000 group was significantly decreased (p<0.01). Like female rats, the thickness of zR in NP-treated groups was significantly decreased (NP-50, p<0.001; NP-5000, p<0.05).
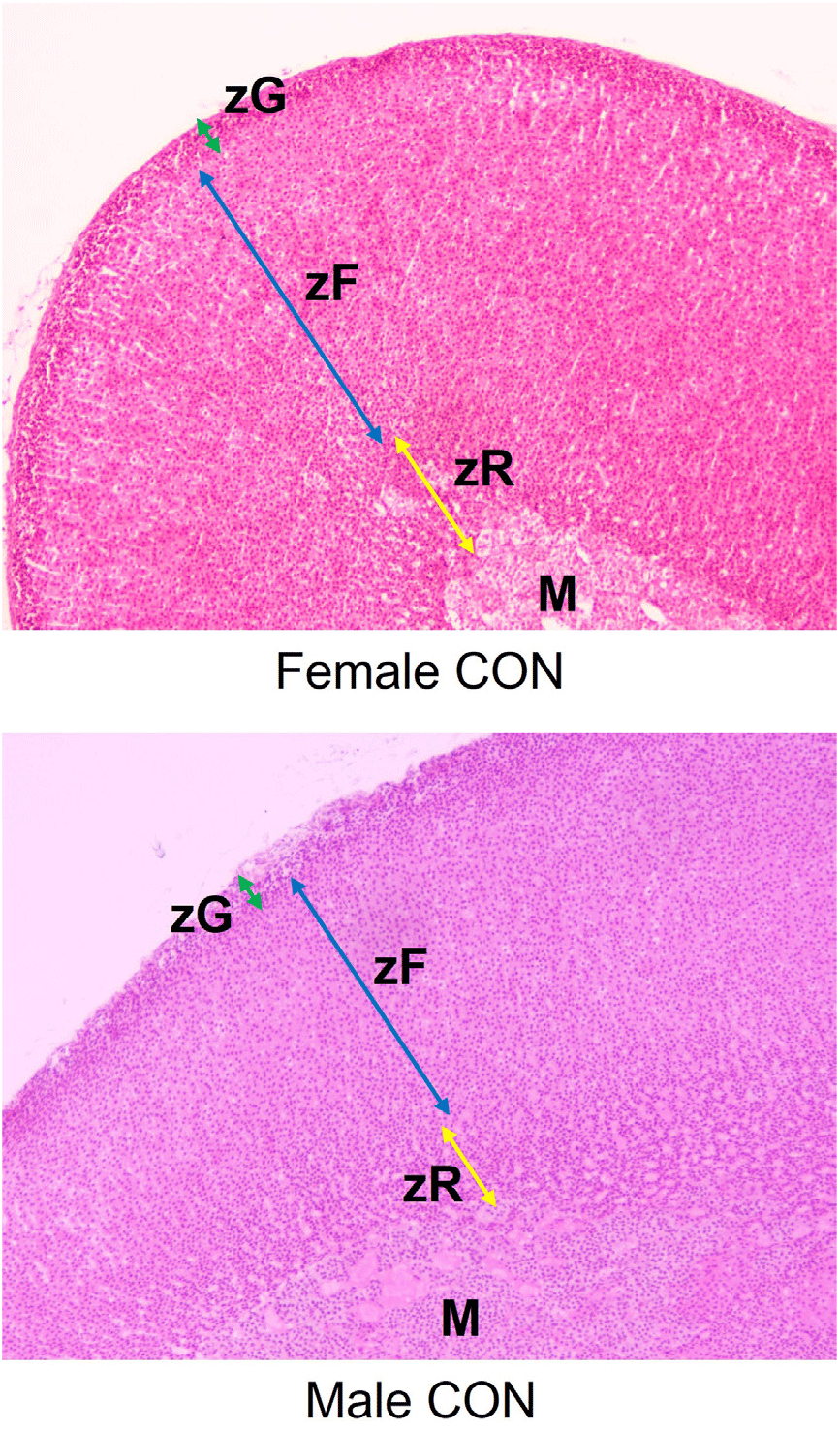
DISCUSSION
The adrenal glands are composed of two heterogenous types of tissue, medulla and cortex. The adrenal cortex is further divided into three main zones: the zG, the zF and the zR. These zones produce three main types of steroid hormones: mineralocorticoids (aldosterone), glucocorticoids (cortisol and corticosterone), and weak androgen dehydroepiandrosterone, respectively (Miller & Auchus, 2011). Since most of EDCs affect the mechanism of steroidogenesis in the gonads, it is highly likely that EDCs also affect steroidogenesis in the adrenal gland. Indeed, there is a wide number of EDCs with recorded in vitro and in vivo adrenal effects covering most of their classes: pesticides, plasticizers, dioxins, PCBs and polycyclic aromatic hydrocarbons (Ribelin, 1984; Szabo & Lippe, 1989; Sanderson et al., 2006; Whitehead & Rice, 2006). Although relatively few studies have been conducted when compared to other EDCs, NP also affect adrenal function in fishes and frogs (Capaldo et al., 2012; Di Lorenzo et al., 2020), lizard (de Falco et al., 2014; Di Lorenzo et al., 2020), bovine (Liu et al., 2006), and rodents (Chang et al., 2010, 2012, 2014; Kim et al., 2019; Lee et al., 2021). Previous and present studies conducted by our group also revealed that the significant changes were found in adrenal glands of low-dose NP-treated rodents, such as adrenocortical histology and steroidogenesis-related gene expressions (Kim et al., 2019; Lee et al., 2021).
The adult adrenal cortex is a dynamic organ in which senescent cells are replaced by newly differentiated ones, and this constant renewal facilitates organ remodeling in response to physiological demand for steroids or certain pathophysiological conditions (Pihlajoki et al., 2015).
Zones can reversibly enlarge, shrink, or alter their biochemical profiles to accommodate physiological needs or in response to experimental manipulations, including [Na+] and [K+] in diet, hormones and/or analogs such as ACTH and dexamethasone, physiological events such as adrenarche and gonadectomy (Pattison et al., 2009; Yates et al., 2013; Pihlajoki et al., 2015). The remarkable regenerative capacity of the adrenal cortex mainly come from distinct pools of stem/progenitor cells exist in the adrenal capsule, subjacent cortex, juxtamedullary region, and other sites (Walczak & Hammer, 2015). In the rat adrenal cortex, an undifferentiated zone between the zG and zF is thought to contain progenitor populations that supply renewal of all steroidogenic zones (Mitani, 2014), while cells expressing oct4 scattered throughout the cortex have been reported (Yaglova et al., 2019). In this context, the following events could happen sequentially; EDC exposure – adrenocortical remodeling – altered or impaired morphogenesis/function of cortical zone(s). In this regard, recent paper that developmental low-dose exposure to dichlorodiphenyltrichloroethane, an organochlorine pesticide class EDC, induced slower development and lower functional activity of the zG during the pubertal period associated with higher expression of oct4, a transcriptional hallmark of stem cell, supports this idea (Yaglova et al., 2021). The present study demonstrated that low-dose NP exposure could alter the adrenocortical histology, showing significant shrinkage in zG and zR but not in zF of F1 female rats and significant shrinkage in zF and zR but not in zG of F1 male rats.
It is intriguing that many adrenocortical diseases are more prevalent in women than in men, but the reasons underlying this sex bias are still uncertain (Lyraki & Schedl, 2021). For example, the overall female-to-male ratio in ACTH-dependent and independent Cushing’s is estimated at 3:1 (Lindholm et al., 2001; Steffensen et al., 2010). Cell renewal is also subject to sexual dimorphism, and female mice display ~3 times higher adrenocortical turnover than males, owing partly to higher proliferative activity in all proliferating compartments (Grabek et al., 2019). In male adrenals, this population is mostly dormant, suppressed by circulating gonadal androgens. Another evidence corroborates the suppressive effect of androgens on the adrenocortical renewal rate using a constitutive lineage tracing approach (Dumontet et al., 2018). Our results on the sexually dimorphic pattern of adrenocortical shrinkage in NP-treated F1 rats suggests that the dimorphic pattern of the adrenocortical steroids in the animals.
In the present study, we demonstrated that the adrenal histology could be altered by low-dose NP exposure in F1 rats, and the effect might be sexually dimorphic. Further study will be helpful for understanding possible adrenal pathophysiology induced by EDC exposure, and EDC-related sexually dimorphic phenomena in rodent adrenals.