INTRODUCTION
The epididymal fat is a part of gonadal adipose tissue and is located nearby the epididymis, as named (Berry et al., 2013; Lee, 2020). Based on histochemical characteristics and/or relative localization, the epididymal fat is discriminated into three types, distal, proximal, and tail epididymal fat (Tirard et al., 2007; Lee, 2020). The distal and proximal epididymal fat is the typically known epididymal fat pad, and differential gene expression between these epididymal fat parts has been observed from our previous and other researches, indicating functional differences between distal and proximal epididymal fat parts (Tirard et al., 2007; Lee & Kim, 2019; Lee, 2019). The tail epididymal fat is localized on the caudal epididymis and is continuously enlarged in size as aged (Lee, 2020). Even though the histochemical characteristics and function of tail epididymal fat have not been defined yet, expression patterns of some adipocyte-associated molecules in the tail epididymal fat are distinct from those detected in the distal and proximal epididymal fat pads (Lee, 2020). These observations suggest that the tail epididymal fat would have different functional roles from distal and proximal epididymal fat pads.
The epididymal fat is consisted of fully mature lipid-filled adipocyte and other types of cells, including preadipocyte, vascular cells, and extracellular matrix cells (Cleary et al., 1977). Like other fat tissues, the weight of epididymal fat is continuously increased during postnatal period (Cleary et al., 1977; Gruen et al., 1980), and such growth of epididymal fat is likely due to expansion of mature adipocyte in size and number (Cleary et al., 1977). Numerous factors, including transcription factors, micro RNAs, and growth factors, involve in development of adipocyte (Kuri-Harcuch et al., 2018). In addition, differentiation of preadipocyte into mature adipocyte and proliferation of preadipocyte are precisely controlled by a harmony of regulated expression of various adipogenic genes (Ntambi & Kim, 2000; Kuri-Harcuch et al., 2018). For examples, CCAAT enhancer binding protein (C/EBP) β seems to be the first transcription factor initiating the differentiation of preadipocyte into adipocyte and mediates the expression of C/EBP α at the later stage of differentiation (Ntambi & Kim, 2000). It is generally considered that C/EBP β also influences on the expression of peroxisome proliferator-activated receptor (PPAR) γ, which is a key regulator of downstream adipogenic gene expression for preadipocyte differentiation (Ntambi & Kim, 2000; Kuri-Harcuch et al., 2018). Even though differential expression patterns of adipocyte marker genes among different epididymal fat parts during postnatal development have been evaluated (Lee, 2019; Lee & Kim, 2019; Lee, 2020), the presence and expression patterns of differentiation-related genes of preadipocyte in the epididymal fat have not been examined in detail.
The effect of androgen and estrogen on the adipogenesis has also been extensively examined. It is generally considered that estrogen promotes hyperplasia of adipocyte and androgen likely involves in hypertrophy of adipocyte (Gavin & Bessesen, 2020). Because androgen and estrogen chiefly play their roles by binding with androgen receptor (AR) and estrogen receptor (ER), respectively, it is reasonable to examine expression of these receptors in the epididymal fat parts. In fact, Dieudonne et al. (1995) have demonstrated the presence of AR in the epididymal fat, especially higher level than in subcutaneous adipose tissue, and differential regulatory effect of testosterone via AR depending on proliferation and differentiation of preadipocytes. The expression of ERα (Metz et al., 2016) and ERβ (Cheon et al., 2020) in the epididymal fat has also been shown. Together, even though a direct role of steroid hormones on the epididymal adipose tissue has not been revealed, expression of AR, ERα, and ERβ in the epididymal fat pad suggests the possibility of functional role(s) of steroid hormones on the growth of epididymal fat pad during postnatal period.
Thus, the present research was focused to examine expression of transcription modulators involved in the differentiation of preadipocyte, such as C/EBP family genes [CCAAT enhancer binding protein alpha (Cebpa), CCAAT enhancer binding protein beta (Cebpb), and CCAAT enhancer binding protein gamma (Cebpg)] and sterol regulatory element binding transcription factor 1 (Srebp1), and steroid hormone receptors, including Ar, estrogen receptors (Esr1), and Esr2, in the different epididymal fat parts during postnatal period.
MATERIALS AND METHODS
The C57BL/6N male mice at 1 month of postnatal age purchased from Samtako (OSan, Korea) were randomly separated and assigned into one of 4 postnatal age groups, 2 (n=6), 5 (n=6), 8 (n=6), and 12 (n=6) months of age. Free access to food and water of experimental animals under controlled environment were permitted for entire experimental period. All animal care procedures were performed in accordance with the guidelines for the care and use of laboratory animals of National Research Council in Korea.
The animal was euthanized by CO2 stunning in a chamber at proper age, and the male reproductive tract was taken through a lower abdominal incision and was placed in cold PBS (Gibco, Grand Island, NY, USA). The epididymal fat pad separated from the epididymis was dissected and divided into the distal and proximal epididymal fats. The tail epididymal fat adjacent to caudal epididymis was collected and pooled in same age group to obtain total RNA extract sufficient for quantitative real-time polymerase chain reaction (PCR). Isolated epididymal fat tissues were quickly frozen in liquid nitrogen and stored in −80°C freezer for further use.
The total RNA pellet from epididymal fat tissue homogenized in TRI REAGENT solution (Molecular Research Center, Cincinnati, OH, USA) was extracted by consecutive exposure to chloroform and isopropanol. The total RNA was dissolved and resuspended in DNase/RNase-free dH2O. The concentration of total RNA extract was determined by UV spectrophotometry (NanoDrop Lite, Thermo Scientific, Wilmington, DE, USA), and the general quality of total RNA extract was checked by 1.2% agarose gel electrophoresis.
One microgram of total RNA was utilized to generate the first-strand of cDNA with reverse transcription (RT) mixture (iScrip™ Reverse transcription Supermix for RT-qPCR, Bio-Rad Laboratories, Hercules, CA, USA) and nuclease free-dH2O to adjust 20 μL in a final reaction volume. The condition of RT reaction was at 25°C for 5 min, 46°C for 20 min, and then 95°C for 1 min.
One microliter of cDNA solution was mixed with 10 pmol of each primer, 7 μL of iQ™ SYBR® Green Supermix (Bio-Rad Laboratories), and nuclease free-dH2O to make a final PCR volume of 20 μL. The sequence and other information of each primer are summarized in Table 1. The PCR was carried out in a sequence of a pre-denaturation at 95°C for 5 min, cycles of a denaturation at 95°C for 30 sec, an annealing at Tm for 30 sec, and an extension at 72°C for 30 sec. An extension step at 72°C for 10 min was added at the end of each PCR. Agarose gel electrophoresis was always performed to check the size of PCR product. The 18s ribosomal RNA (Rn18s) gene was included as an internal control of PCR analysis.
PCR, polymerase chain reaction; Cebpa, CCAAT enhancer binding protein alpha; Cebpb, CCAAT enhancer binding protein beta; Cebpg, CCAAT enhancer binding protein gamma; Srebp1, sterol regulatory element binding transcription factor 1; Ar, an-drogen receptor; Esr1, estrogen receptor alpha; Esr2, estrogen receptor beta; Rn18s, 18s ribosomal RNA.
Independently triplicated RT reactions and PCRs were accomplished to get a mean and standard error for each target gene at a particular postnatal age. Expression level of each molecule was normalized to that of Rn18s, and the data in a graph were presented in the relative ratio against transcript level of a specific gene at 2 months of age. The statistical comparison for expression level of a target gene among postnatal age groups was determined by one-way ANOVA. If there was a statistical significance present within experimental age groups for expression level of the target gene, Duncan’s test, a post-hoc analysis, was then employed to evaluate statistical significance among different age groups. Less than 0.05 of p-value was considered as the existence of statistical difference on the transcript level of the gene among different age groups, represented with different letters on bars in the graph.
RESULTS
The expression level of Cebpa in the distal epididymal fat at 5 months of age was significantly higher than that at 2 months of age (Fig. 1A). A tremendous increase of Cebpa transcript level in the distal epididymal fat was observed at 8 months of age, followed by an additional increase of Cebpa expression at 12 months of age (Fig. 1A). In the proximal epididymal fat, expression level of Cebpa was not changed until 5 months of age (Fig. 1B). However, expression of Cebpa was significantly increased at 8 months of age, and there was no significant difference of Cebpa transcript level between 8 and 12 months of age (Fig. 1B). Like the proximal epididymal fat, there was no significant change of Cebpa expression at 5 months of age in the tail epididymal fat, compared with that at 2 months of age (Fig. 1C). An increase of Cebpa transcript level in the tail epididymal fat was detected at 8 months of age (Fig. 1C). Expression level of Cebpa in the tail epididymal fat at 12 months of age was significantly higher than that at 8 months of age (Fig. 1C).
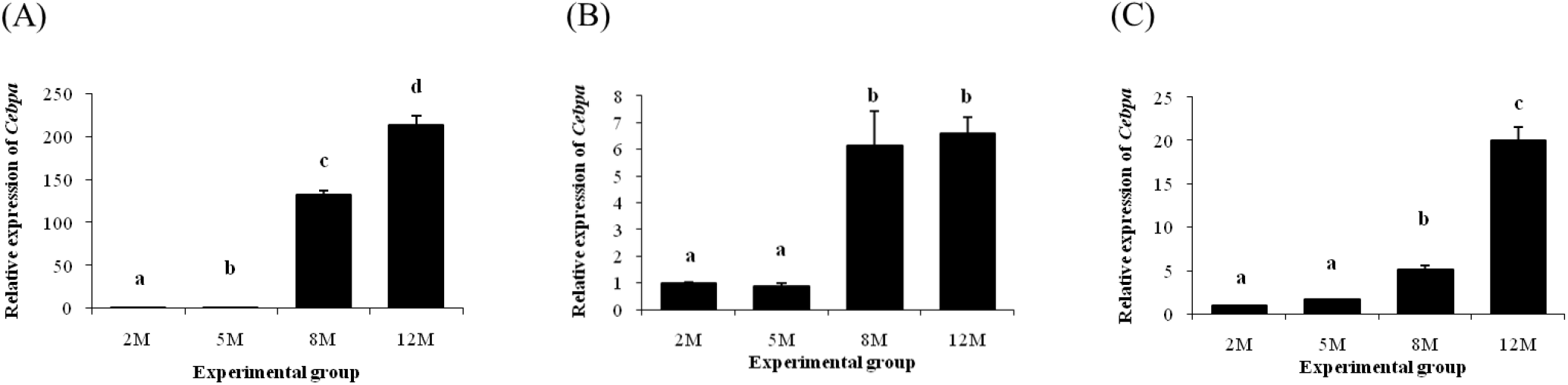
Fig. 2 shows expression patterns of Cebpb in different epididymal fat parts at several postnatal ages. In the distal epididymal fat, expression level of Cebpb was significantly increased with age (Fig. 2A). But, there was no change of Cebpb transcript level in the proximal epididymal fat at 5 months of age, compared with the level at 2 months of age (Fig. 2B). Then, expression level of Cebpb in the proximal epididymal fat was significantly increased at 8 months of age, followed by a decrease at 12 months of age (Fig. 2B). Expression of Cebpb in the tail epididymal fat was significantly increased at 5 months of age, along with an additional increase of Cebpb transcript level at 8 months of age (Fig. 2C). However, there was no further significant change of Cebpb expression level at 12 months of age (Fig. 2C).
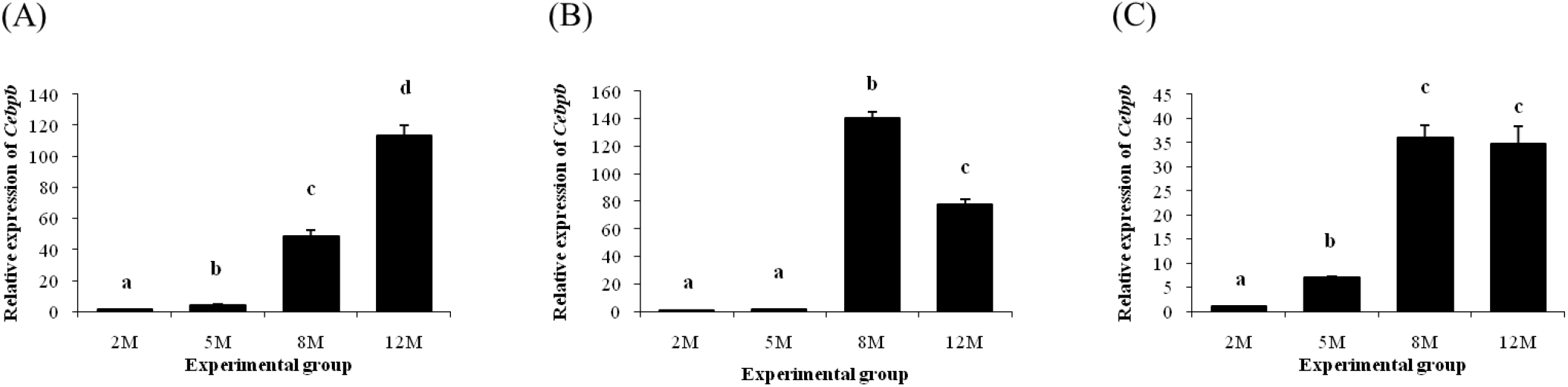
A significant increase of Cebpg in the distal epididymal fat was observed at 5 months of age, compared with at 2 months of age (Fig. 3A). Further increases of Cebpg transcript levels in the distal epididymal fat were also detected at 8 and 12 months of age (Fig. 3A). In the proximal epididymal fat, an increase of Cebpg expression was found at 8 months of age, followed by a significant drop at 12 months of age (Fig. 3B). Expression pattern of Cebpg in the tail epididymal fat was quietly similar with that in the distal epididymal fat, which showed continuous increase of Cebpg transcript level with age (Fig. 3C).
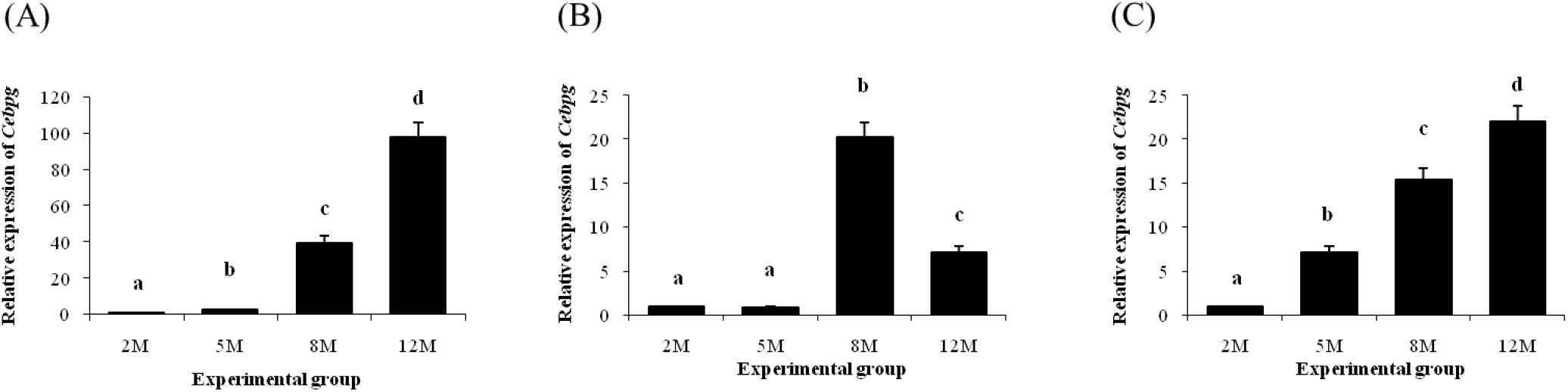
An increase of Srebp1 transcript level in the distal epididymal fat was detected at 8 months of age (Fig. 4A). Expression level of Srebp1 at 12 months of age was higher than that of 8 months of age (Fig. 4A). Expression pattern of Srebp1 in the proximal epididymal fat was almost same with that in the distal epididymal fat (Fig. 4B). An increase of Srebp1 transcript level was first observed at 8 months of age, followed by another significant surge of Srebp1 expression at 12 months of age (Fib. 4B). The level of Srebp1 transcript in the tail epididymal fat was significantly increased as aged (Fig. 4C).
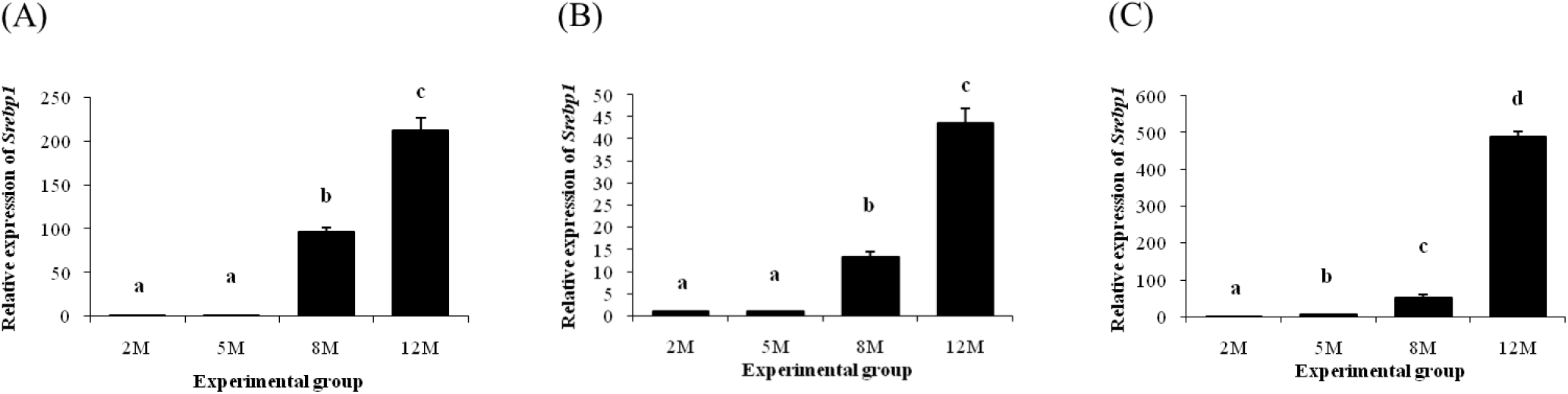
Expression of Ar in the distal epididymal fat was significantly increased at 5 months of age (Fig. 5A). The level of Ar transcript in the distal epididymal fat was further increased at 8 months of age, followed by another increase of Ar transcript level at 12 months of age (Fig. 5A). The level of Ar transcript in the proximal epididymal fat at 5 months of age was also higher than 2 months of age (Fig. 5B). An additional increase of Ar expression level in the proximal epididymal fat was detected at 8 months of age, but a significant decrease of Ar transcript level at 12 months of age was followed in the proximal epididymal fat (Fig. 5B). Expression of Ar in the tail epididymal fat was significantly increased at 5 months of age, even though there was no change of Ar transcript level between 5 and 8 months of age (Fig. 5C). The abundance of Ar transcript in the tail epididymal fat at 12 months of age was higher than that at 8 months of age (Fig. 5C).
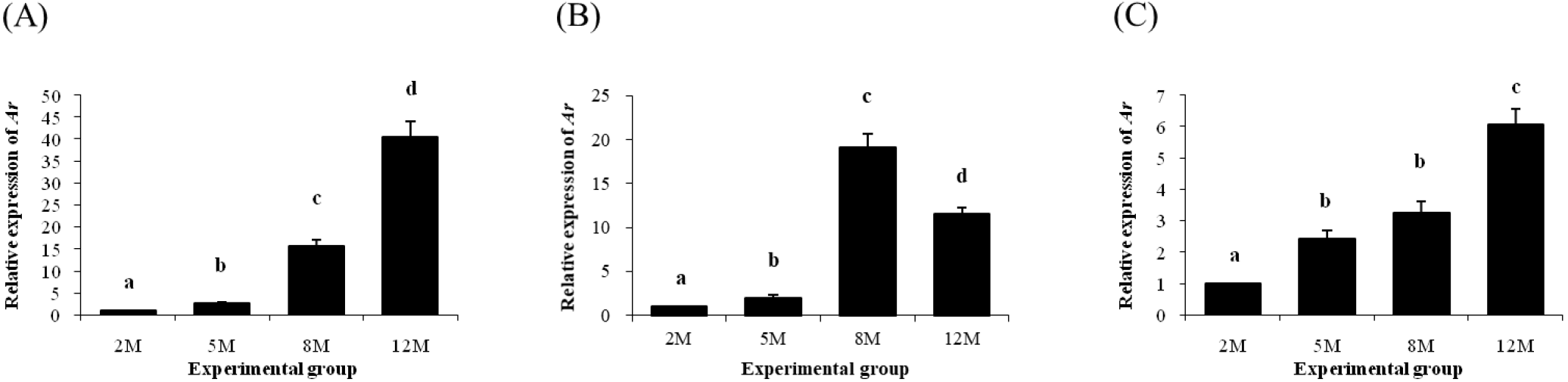
Expression of Esr1 in the distal epididymal fat was first detected at 2 months of age, followed by a significant drop of Esr1 transcript level at 5 months of age (Fig. 6A). The presence of Esr1 expression in the distal epididymal fat at 8 and 12 months of age was not observed (Fig. 6A). Expression pattern of Esr1 in the proximal epididymal fat during postnatal period was almost same as in the distal epididymal fat (Fig. 6B). There was no Esr1 expression detected in the tail epididymal fat during the entire postnatal period (Fig. 6C).
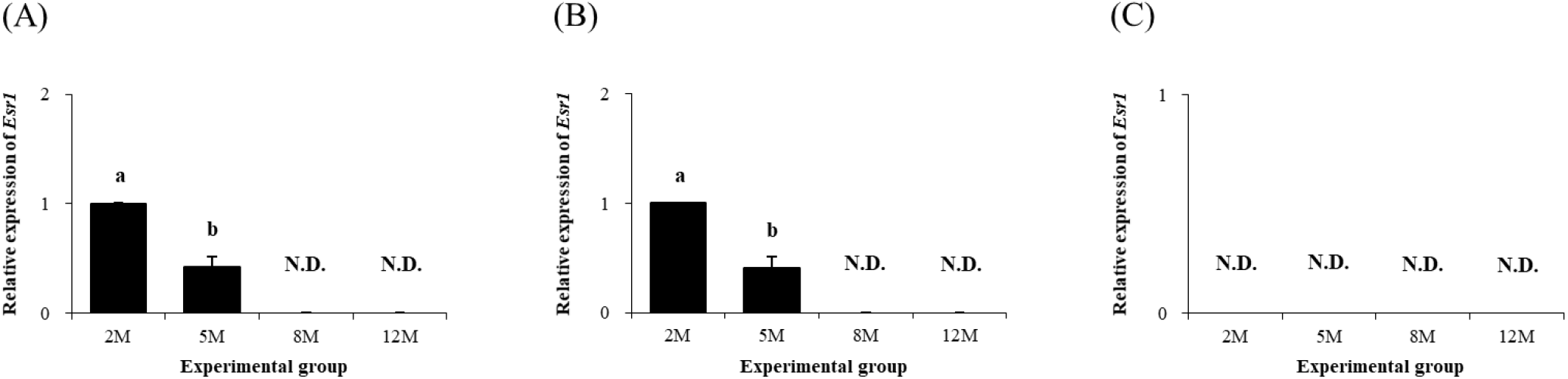
Expression pattern of Esr2 in different epididymal fat parts during postnatal period is shown in Fig. 7. Expression of Esr2 in the distal epididymal fat was significantly increased at 5 months of age, followed by continuous increases at 8 and 12 months of age (Fig. 7A). In the proximal epididymal fat, the transcript level of Esr2 at 5 months of age was higher than that at 2 months of age (Fig. 7B).
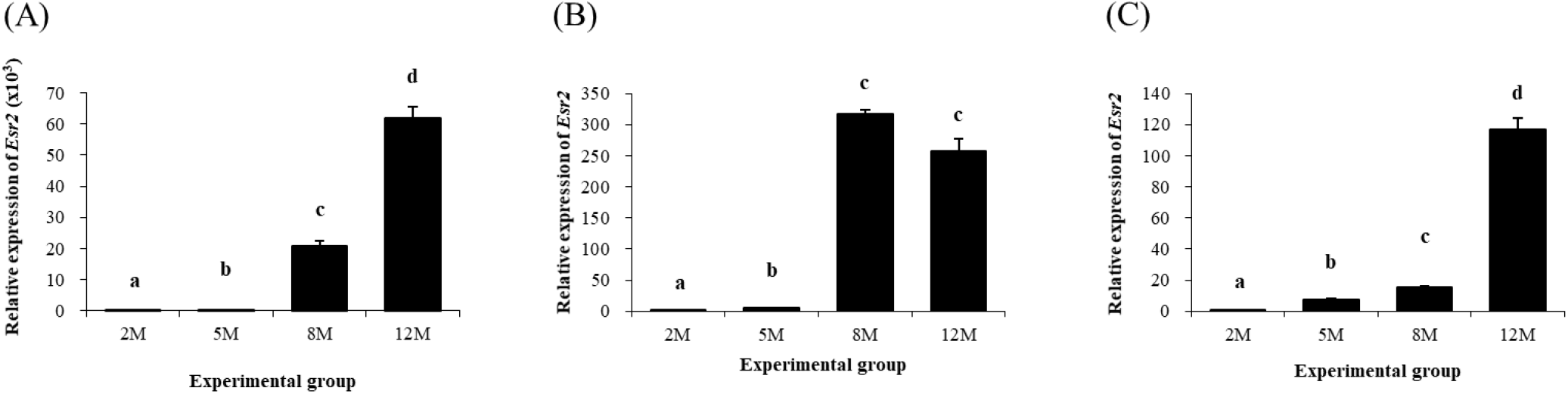
A surge of Esr2 transcript level was also detected at 8 months of age, followed by no change of Esr2 expression level at 12 months of age (Fig. 7B). Expression of Esr2 in the tail epididymal fat was also detected and significantly increased as aged (Fig. 7c).
DISCUSSION
The differentiation of preadipocyte into differentiated mature adipocyte requires the coordinated actions of a variety of transcription factors and modulators, as well as growth factors and hormones. It is generally acknowledged that the induction of C/EBPβ and C/EBPδ in the early stage of adipogenesis promotes expression and activation of PPARγ and C/EBPα, key regulators for establishing terminal differentiation of adipocyte and maintaining mature adipocyte phenotypes (Lee et al., 2018). In addition, SREBP1, a transcription factor involved in the differentiation of preadipocyte, participates in adipose differentiation via an increase of transcriptional activity of PPARγ (Kim et al., 1998). Moreover, the concern of actions of androgen and estrogen on the development of epididymal fat tissue has been suggested (Dieudonne et al., 1995; Metz et al., 2016; Gavin & Bessesen, 2020). Thus, in the current research, expression patterns of these transcription factors and steroid hormone receptors within different epididymal fat regions during postnatal development have been examined using quantitative real-time PCR analysis.
The C/EBP family is a group of transcription facts involved in regulation of various physiological processes, including adipogenesis (Renfro et al., 2022). Of C/EBP proteins, C/EBPβ is an initiation regulator for the differentiation of preadipocyte into mature adipocyte by stimulating expression of C/EBPα and PPARγ (Lee et al., 2018). Then, C/EBPα and PPARγ promote a variety of adipogenic factors, required for terminal differentiation of immature adipocyte into mature one and maintenance of mature status (Ali et al., 2013; Lee et al., 2018). Even though functional roles of C/EBPα and C/EBPβ are extensively studies, less attention has been caught on molecular functions of C/EBPγ. It seems that C/EBPγ contributes its major roles on cell proliferation, development of hematopoietic and nervous system, and integrated cell stress response (Renfro et al., 2022). In addition, a possible role of C/EBPγ in energy homeostasis has been suggested (Renfro et al., 2022). Thus, it is supposed that C/EBPγ in adipocyte would involve in expression of marker molecules expressed from fully differentiated adipocyte and in conservation of mature status, rather than differentiation of preadipocyte. In the distal and tail epididymal fat parts, expression of Cebpa, Cebpb, and Cebpg is continuously increased until 12 months of age. These results have been expected because such expression patterns of Cebp genes would be necessary for increase of mature adipocyte in size and number with age (Cleary et al., 1977). However, expression of Cebp genes in the proximal epididymal fat between 2 and 5 months of age was not different. Moreover, transcript levels of Cebp genes at 12 months of age was not changed or even decreased, compared with that at 8 months of age. Such regional differences of the epididymal fat on expression patterns of Cebp genes during postnatal period imply that postnatal development of the proximal epididymal fat would be distinguishable from those of the distal and tail epididymal fat. In addition, to our knowledge, this is the first time to show expression patterns of Cebpg in the epididymal fat tissue. The physiological role of Cebpg in the epididymal fat is not clearly suggested at this point. Detailed molecular examination of Cebp genes in different epididymal fat parts is suggested to provide detailed information about functional roles of these genes.
Along with C/EBPs, SREBP1 is another factor acting in the early stage of differentiation of preadipocyte into adipocyte by stimulating expression of adipogenic genes (Kim & Spiegelman, 1996; Niemelä et al., 2008). Expression of Srebp1 in the rat whole epididymal fat has been observed from our previous research, showing a higher level of Srebp1 transcript level at the old age than that at the young age and the fluctuation of Srebp1 expression during the early postnatal period (Lee & Kim, 2018). In the present research, the levels of Srebp1 transcript in the distal and proximal epididymal fat remain unchanged, followed by significant increases at 8 and 12 months of age. On the other hand, expression of Srebp1 in the tail epididymal fat was continuously increased during postnatal period. Because the size and number of mature adipocyte become increased with age, it is reasonable to consider that extended and escalated action of SREBP1 in the epididymal fat would be required to express adequate amount of adipogenic genes at the old age. Examination of expression levels of adipogenic genes after selective inhibition of Srebp1 expression would provide valuable information for functional role of SREBP1 in the epididymal fat.
The adipogenesis and development of adipose tissue are also regulated by testosterone and estrogen (Gavin & Bessesen, 2020). The effects of estrogen on the development of adipocyte are not straightforward. Estrogen promote proliferation of adipocyte precursor by inhibiting adipogenesis, while another evidence indicates stimulatory effect of estrogen on adipocyte differentiation (Newell-Fugate, 2017). Thus, Gavin & Bessesen (2020) have addressed that the effect of estrogen on adipocyte development is likely different, depending on the stage of adipocyte development. In spite of such complicate effects of estrogen on the development of adipocyte, it is generally agreed that estrogen exerts its effect via ESR1 (Newell-Fugate, 2017; Gavin & Bessesen, 2020). Testosterone via AR action has a suppressive effect on preadipocyte differentiation and overall reductive effect of adipose tissue mass (Newell-Fugate, 2017; Gavin & Bessesen, 2020). Increased expression of Ar and decreased expression of Esr1 with age have been detected in the distal and proximal epididymal fat. Even no Esr1 expression during postnatal period has been observed in the tail epididymal fat. An increase of Esr2 transcription level with age has been found in all types of the epididymal fat. In fact, opposite expression patterns of Ar and Esr1 in the epididymal fat have been expected. Such unexpected outcomes on Ar an Esr1 expression patterns would be due to different types of adipose tissue. Indeed, differential responses depending on types of white adipose tissues (WAT) to testosterone and/or estrogen have been frequently suggested from other researches (Newell-Fugate, 2017; Gavin & Bessesen, 2020). Moreover, it is possible that these steroid hormones have unknown specific roles restricted to the development of epididymal fat. Thus, it is encouraged to examine the effects of testosterone and estrogen on postnatal development of the epididymal fat, which are separated from those on other types of adipose tissue.
The findings from the present study remain several questions to be answered for the development of epididymal fat during postnatal period. Despite, the current research has a value on the detection of differential expression patterns of several transcription factors-associated with preadipocyte differentiation and steroid hormone receptors in different epididymal fat parts at several postnatal ages. More attention has to be paid for evaluation of molecular biological roles of these transcription factors and steroid hormones within each epididymal fat part.