INTRODUCTION
‘Sea bass’ is the common name for a variety of marine fish species worldwide. In Northeast Asia, Lateolabrax spp. are exclusively sold and consumed as sea bass. These fish are native to the western Pacific Ocean, occurring in waters from Korea and Japan to the South China Sea. Three sea bass species inhabit Korean coastal waters: the spotted sea bass (Lateolabrax maculatus), which lives from the southwest sea of Korea to the Yellow Sea of China; and the blackfin seabass (L. latus) and Japanese seabass (Lotus japonicus), both of which are distributed from the southeast sea of Korea to the western sea of Japan. In South Korea, L. maculates and L. japonicus are very important food resources. Both species have been massively maricultured via inshore net-cages over the last 20 years. The average annual sea bass mariculture production in South Korea is about 2,000 metric tons per year, a large proportion of which is L. maculates. Many aquaculturists have refined the mariculture and artificial reproduction of L. maculates using land-based tanks of indoor facilities. However, the mariculture of L. japonicus has relied on wild-collected eggs, fries, or juveniles, such that cultured production is erratic and insufficient.
The reproductive cycle of teleost is regulated by the endocrinological activity of the central nervous system, which depends on environmental factors such as temperature, salinity and the light cycle (Juntti & Fernald, 2016). To domesticate a fish species, it is necessary to gain control over all phases of its life cycle to achieve reproductive success under artificial rearing conditions. Many fish do not reproduce in captive conditions; females commonly fail to undergo oocyte maturation, ovulation, or spawning, while males produce milt in small volumes (or of low-quality). It is therefore critical to understand the reproductive biology of fish destined for domestication.
We know that a broad distinction can be drawn between the physio-ecological reproductive traits of different sea bass. The maturation and spawning of L. japonicus are unpredictable in captivity compared to L. maculates. We also have very limited data on protocols for the induction of spawning, making it difficult to consistently produce fertilized eggs within the rearing tanks of indoor facilities. Recently, we found that sea bass will spontaneously spawn fertilized eggs in inshore net-cages, implying that the fish may also breed indoors. The objective of this study is to provide basic knowledge on the reproductive biology of L. japonicus, and to found spontaneous reproduction without stress after transportation from net cage into indoor tanks and the possibility of producing mass fertilized eggs.
MATERIALS AND METHODS
Sea bass (female total length [TL]: 53.8±1.4 cm, body weight [BW]: 1.7±0.3 kg; male TL: 49.0±0.1 cm, BW: 1.1±0.1 kg) were selected from among brood stock reared in a commercial net cage. They were transported to indoor, 20-ton flow-through tanks in January of their first year. All fish were exposed to a photo- and thermo-period environment that gradually changed from 4.2±1℃ and 8 L:16 D in the winter to 25±1℃ and 16 L:8 D in the summer with daily light cycle (LED light) and temperature conditions (Fig. 1). They were fed a moist pellet diet (% protein) to satiation every day, except on sampling days.
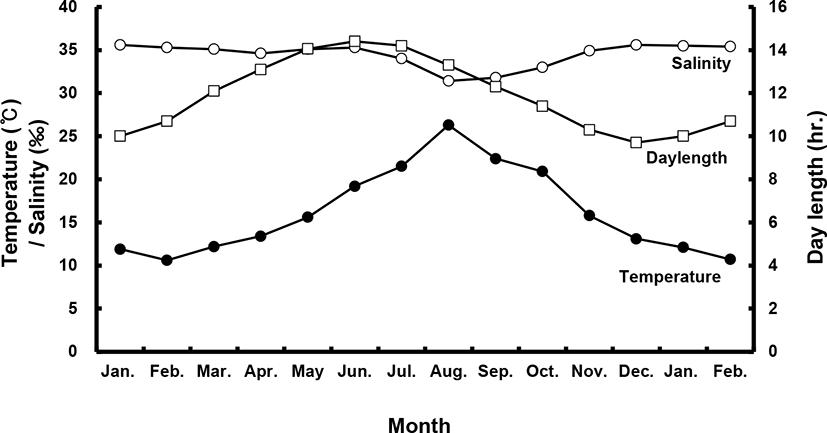
During the experimental period (2017–2018), all fish were categorized according to BW, and only medium-sized fish (47–53 cm, 1–1.5 kg) were sampled. All fish were maintained and sampled according to the following protocols. Monthly sampling of female and male populations occurred from January 2017 to February 2018. At each sampling time, 10–20 individuals per sex were randomly collected and anesthetized with 2-phenoxyethanol (1 mL/L; Sigma, St. Louis, MO, USA), and BW and TL were measured. Gentle abdominal pressure was applied to check for milt or eggs. Male fish were classified as “spermiating” if milt was present. Blood was collected from the caudal vein using a heparinized syringe and the plasma was stored at –80℃ until analysis. Fish (n=40) were sacrificed via decapitation; gonads, livers and intestines were then removed and weighed for calculation of the gonadosomatic index (GSI; % = [gonad weight / BW] × 102), hepatosomatic index (HSI; % = [liver weight / BW] × 102) and viscerosomatic index (VSI; % = [intestine weight / BW] × 102).
Fragments were taken from the mid-section of gonads and fixed in Bouin’s solution for 1 day. Following serial dehydration with ethanol, tissues were embedded in paraffin. Serial sections of 4–5 µm was obtained and stained with hematoxylin-eosin solution. The reproductive stages of the fish were classified; oocyte development classification was done according to the criteria of Alvariño et al. (1992), Selman et al. (1993) and Nagahama & Yamashita (2008); testis development classification was based on the studies of Loir et al. (2001).
Plasma concentrations of testosterone (T) and estradiol (E 2) were measured by T ELISA kits (cat. No. MBS 281909; MyBioSource, San Diego, CA, USA) and E 2 ELISA kits (cat. No. MBS283228; MyBioSource), respectively.
To assess fecundity and monitor spawning in females, ovaries were removed monthly from 10 females sacrificed by decapitation, and fixed in formalin. We measured the number and diameter of oocytes obtained from the brood stock during maturation and spawning between November 2017 and March 2018. Fragments of the formalin-fixed ovaries were mechanically separated, and then the oocyte diameters were measured with an ocular micrometer under a dissecting microscope. Fecundity (eggs×103) by fish size (TL and BW) was examined in hydrated ovaries taken from 40 females during spawning season. Briefly, the number of hydrated oocytes preserved in formalin was counted in pre-weighed ovaries, and fecundity was estimated per fish.
The potential effects of salinity on the spontaneous maturation and spawning of 2.5-year-old females were investigated by comparing females raised in normal salinity seawater (34.5±0.4 psu, n=40) and low salinity seawater (29.6±0.9 psu, n=40). This experiment lasted about 8 weeks and occurred between February and March 2017. The mean water temperature was 13.8±1.2℃. At the beginning of the experiment, fish were weighed, sorted by sex (outside observation), tagged, and placed in concrete tanks equipped with outlets for automatic drainage and collection of floating eggs. During spawning, the eggs collected were counted and measured, and the hatching rate was monitored.
RESULTS
The GSI of females reared in the indoor tank underwent marked cyclic variations: values in January and February 2017 were somewhat higher than those in other months, and then remained below 2.0% between April and September. The GSI of females increased again in November, reaching a maximum in next year February (Fig. 2). In January 2017, oocytes in the tertiary yolk stage first appeared, and oocytes in the final maturation and spawning stages were observed in February 2017. After ovulation and spawning between March and August, numerous atretic follicles and degenerative oocytes were present. Between September and October, oogonia were predominant, with a few small primary oocytes. Between November and December, the ovaries contained growing previtellogenic oocytes with dense, homogeneous cytoplasm and larger oocytes bearing cortical alveoli. In the vitellogenic stage during December, yolk globules appeared in the periphery of the oocytes and became more numerous with ovarian development. In January 2018, more than half of the females contained spherical oocytes in which yolk granules dispersed throughout the cytoplasm and zona radiata; by the end of January, oil and yolk globules had increased in number and size. In February 2018, many oocytes underwent final maturation with the migration of peripheral germinal vesicles. At that time, most females contained both small (<500 µm in diameter) and large oocytes (≥500 µm in diameter; Table 1; Figs. 3 and 4).
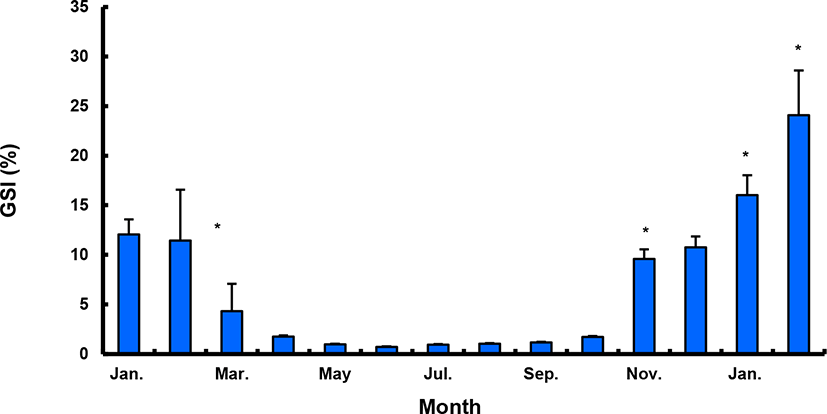
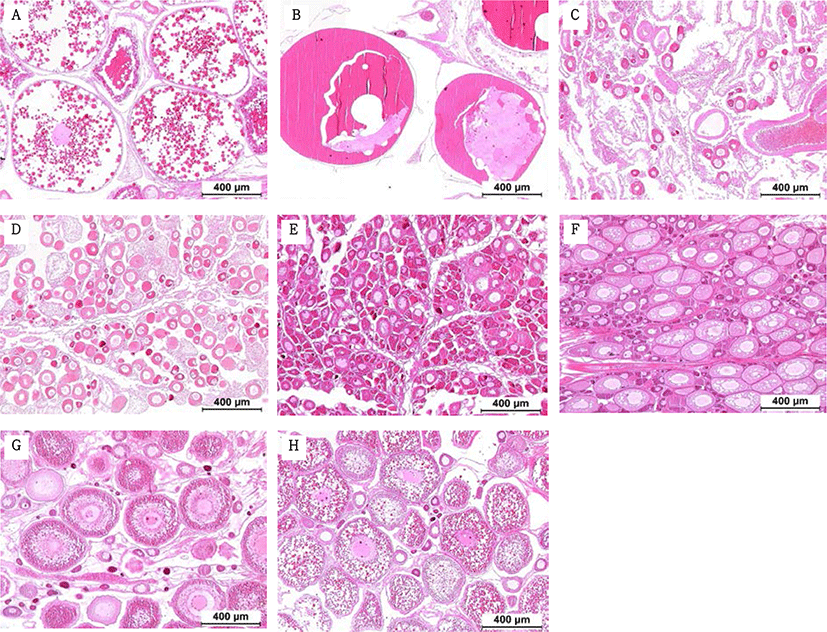
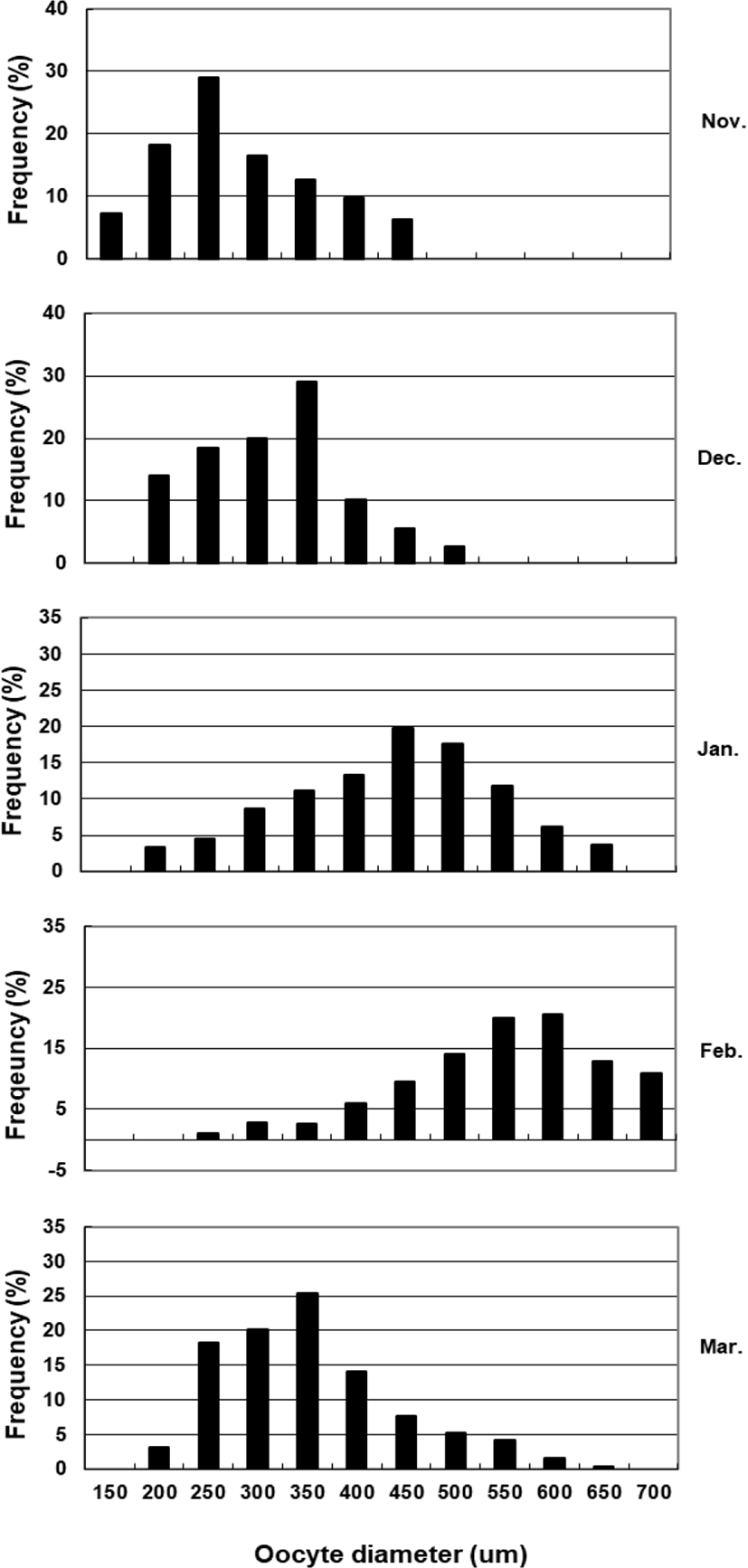
Symbols (from + to +++) indicates the relative abundance of each germ cell type; +/-, present in some individuals; (+), rare.
Although the number of eggs was different in each fish, the sea bass had oocytes ranging from the previtellogenic to vitellogenic stage; oocytes at the perinucleous stage (40–100 µm), primary oocytes (100–150 µm; transparent), and vitellogenic and mature oocytes (350–700 µm) were all observed. Hydrated eggs (700–1,230 µm) were clearly distinct from the advanced vitellogenic and maturate oocytes (<700 µm in diameter) due to the increase in volume associated with hydration (Figs. 3 and 4). Fecundity (calculated from the hydrated oocytes) ranged from 495×103 to 1,913×103 eggs per female. A significant positive linear relationship was found between fecundity and body size. The mean annual fecundity was 536,000±74,000 eggs per kg BW (Fig. 5).
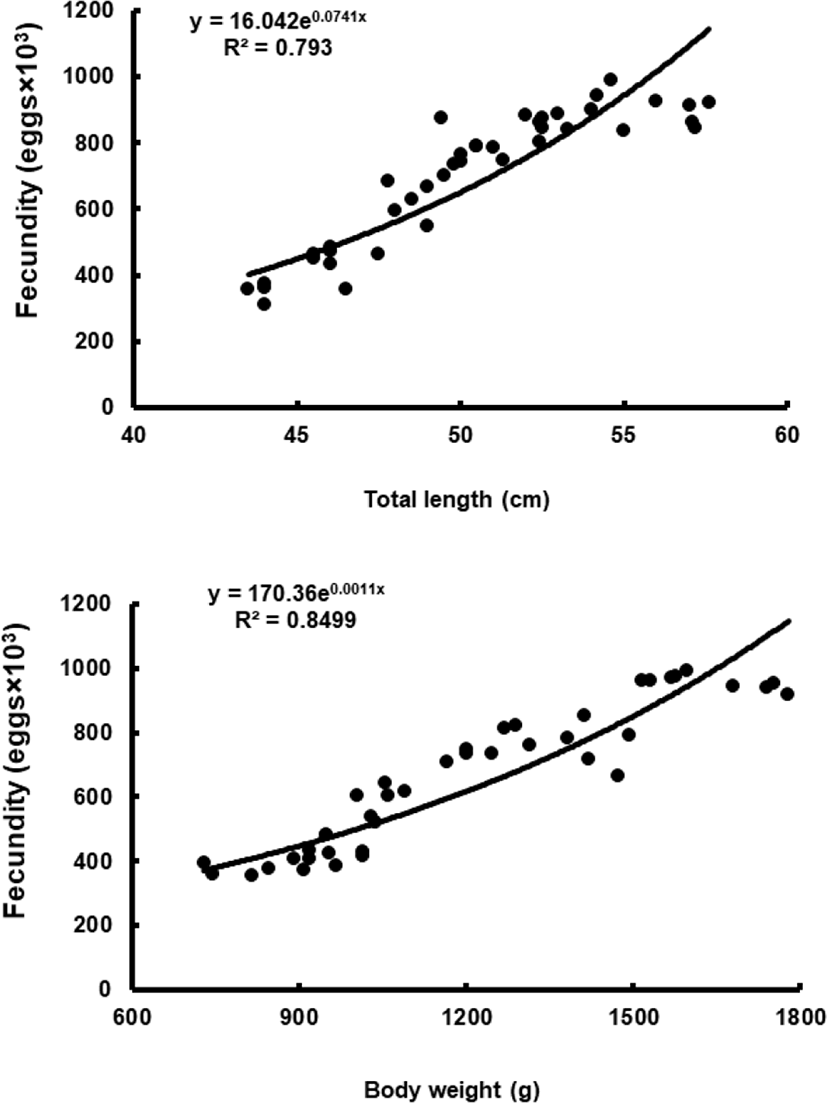
Males had the highest GSI in January and February 2017, which decreased in March and remained less than 1% between April and September. After November, the values increased again and reached a maximum in January (Fig. 6). Histological observation revealed that most males developed testes containing predominantly spermatocytes, spermatids, and spermatozoa in January, and mature testes with spermatids and spermatozoa in February. The testes preceded toward the degeneration stage in March and reached a resting stage (spermatogonia stage) in April. Between May and September, all males had undeveloped testes containing only spermatogonia lining the tubule wall. In October, spermatocytes became apparent in most males, indicating the initiation of a new reproductive cycle. In November, spermatogenesis was initiated in most males, accompanied by an increase in diameter of the testicular tubules and the production of all germ cells. Numerous spermatid and spermatozoa appeared and spermiation took place from December to next year February (Table 2; Fig. 7).
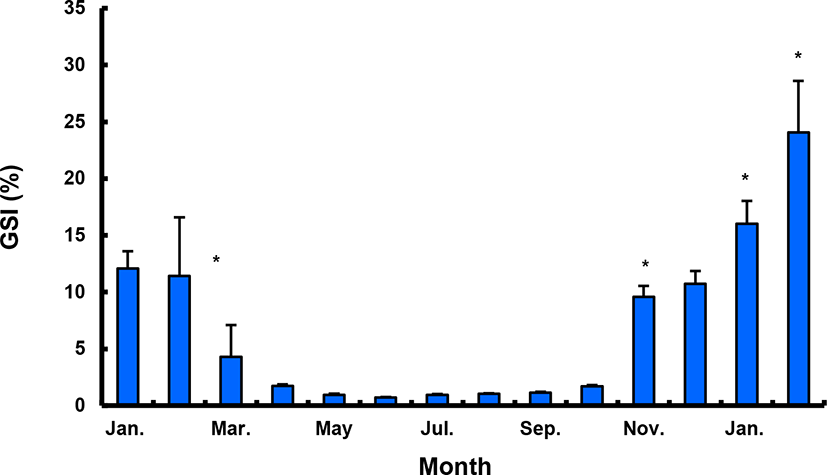
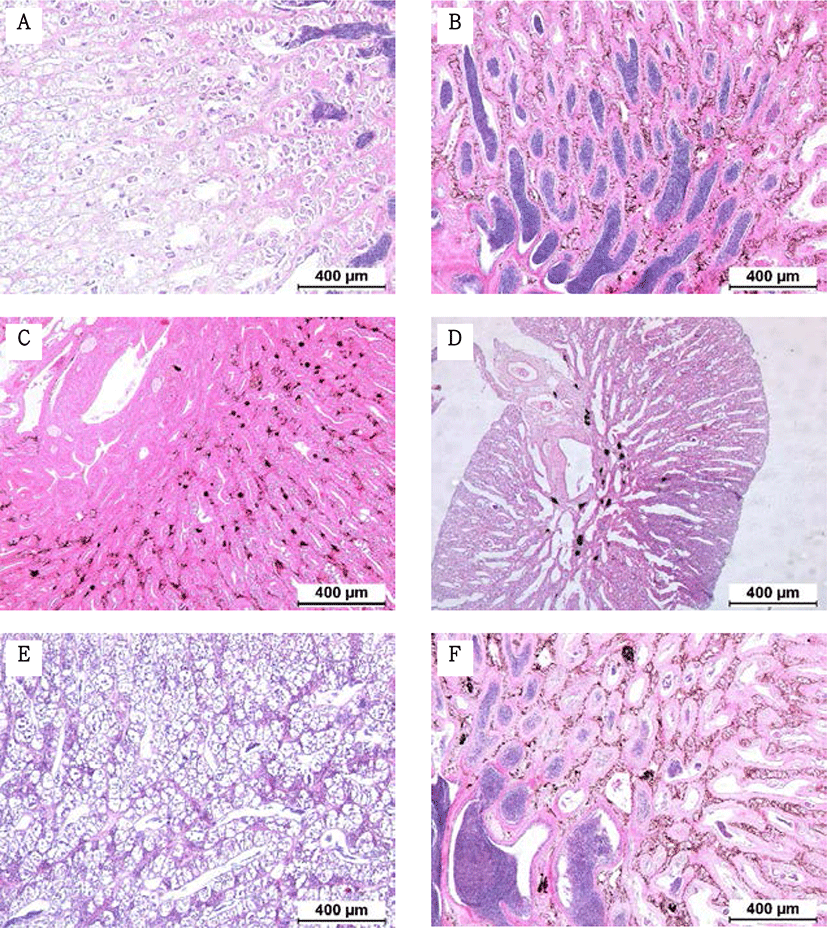
Symbols (from + to +++) indicates the relative abundance of each germ cell type; +/-, present in some individuals; (+), rare.
Plasma T level in female sea bass increased from 0.21±0.08 ng/mL in January 2017 to 0.60±0.45 ng/mL in February 2017, but decreased to the lowest level in May (0.01±0.01 ng/mL) and remained under 0.1 ng/mL until November. Female plasma T increased in December, reaching a peak (1.03±0.38 ng/mL) in January 2018. Female plasma E 2 showed a similar trend. The plasma E 2 levels were 0.03±0.01 ng/mL in January 2017, decreasing after March and reaching the lowest level in summer, then increasing in September and reaching the highest levels, of 1.03–1.07 ng/mL, in December 2017 and January 2018. However, the value decreased again sharply after February 2018 (Fig. 8).
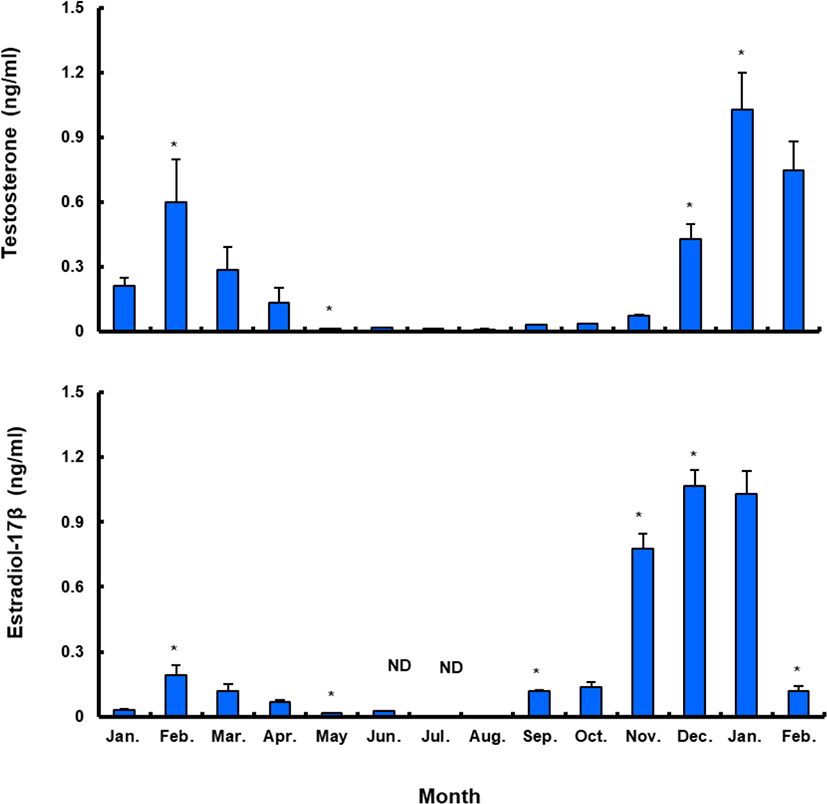
Between January and February 2017, T levels were 0.41–0.47 ng/mL in males, and then decreased significantly in March. From April to September, when testes from all males were undeveloped, the mean plasma T l level was less than 0.10 ng/mL. The level increased again in October and peaked in January 2018 (0.62±0.14 ng/mL), and then decreased significantly in February 2018 (Fig. 9).
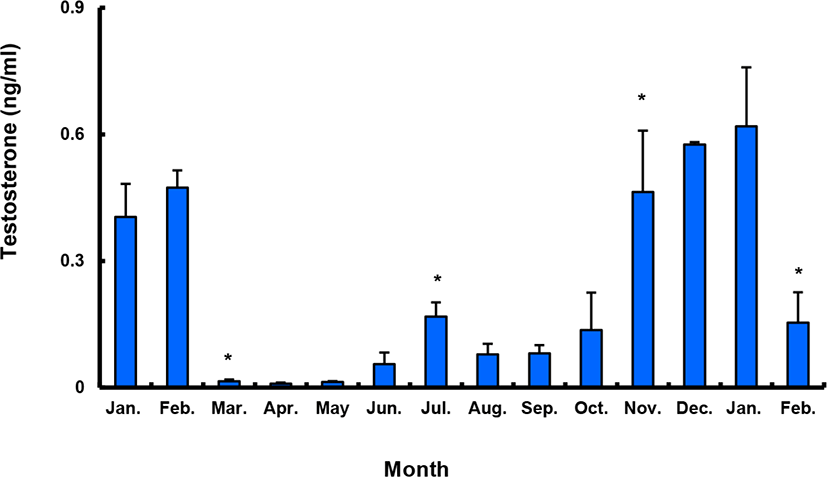
During maturation, females had oocytes in all maturity stages. The number of maturing fish, and the relative frequency of spawning females, was higher in the low salinity treatment than in the full salinity treatment. Fecundity, egg size, and hatching rate were also higher under low salinity conditions (Table 3).
DISCUSSION
In the beginning of annual sexual maturity, our examination showed that there are some differences with the past two studies, Kang et al. (2001) and Kang et al. (2002). Although there was no significant difference in the males, the results in females had some difference with Kang et al. (2001) examined wild the sea bass started maturation in December. The present study showed that females maturation started from November, indicating that there is a time lag between indoor cultured and wild fish. The difference may be due to habitat environment (e.g., water temperature, photovoltaic cycle and nutritional conditions).
In females, we did not found any difference with Kang et al. (2002). However, in males, we know that there is a significant difference between two studies in maturation peak timing. Compared with Kang et al. (2002) showed that maturation of the sea bass peaked at GSI 10% in January, the our found that the maturation peaked at more higher value of GSI 23% in February in indoor-reared the fish. We guess that this difference is also owing to environmental condition. Actually, our experimental was conducted under farming condition with natural daily photovoltaic cycle and water temperatures falling to 4℃ in winter. However, Kang et al. (2002) examined under indoor facility without providing artificial light and water temperatures drop to annual minimum of 9℃. However, in the present study, we did not study whether LED lighting, daily light cycle and annual low water temperature influence the maturation of seabass cultured in indoor. Therefore, we hope that further research will be done on these points in the near future.
Histological observation showed that L. japonicus undergo group-synchronous ovarian development and continuous vitellogenesis, similar to most teleost species (Asturiano et al., 2002; Juntti & Fernald, 2016; Saborido-Rey, 2016) including European sea bass Dicentrarchus labrax L. (Asturiano et al., 2002), Hemiodontidae Hemiodus microlepis (da Silva Brandão et al., 2003), and zebrafish Danio rerio (Ayetkin & Yüce, 2008). Most female L. japonicus were vitellogenic in early December, and some began spawning in mid-January. Although spawning in the wild usually occurs in March, most captive females spawned from February to early March. After the spawning season, ovaries regress until August. Thus, we confirmed that L. japonicus is a typical spring spawning species, in contrast with L. maculatus, which spawns in the fall (Kim et al., 2001).
We also found that plasma E 2 and T levels coincide with oocyte development and maturation in L. japonicus. Sexual development in female teleosts is highly correlated with plasma E 2, and major spikes in blood levels occur prior to ovulation (Norberg et al., 1989; Maruska & Gelsleichter, 2011; Awruch, 2015). Estradiol synthesis is initiated during previtellogenesis and reaches a maximum during vitellogenesis, which is 0.5–1.5 months prior to spawning (Wallace & Selman, 1981; Ng & Idler, 1983). In L. japonicus, E 2 synthesis peaked during vitellogenesis, perhaps similar to the vitellogenin production by the liver seen in other species. Testosterone has been shown to significantly inhibit normal embryonic development, and reduces the maturation of oocytes in mammals (Anderiesz & Trounson, 1995). It has since been demonstrated that testosterone is a promoter of mammalian oocyte maturation (Hammes, 2004). In teleosts, plasma T is related to early oocyte maturation via an increase of pituitary responsiveness to GnRH and ovary responsiveness to GtH (Nagahama & Yamashita, 2008). In addition, increasing plasma T during final oocyte maturation could affect the ovulation of female teleosts (Malison & Held, 1996; Hwang et al., 2012) and decrease during spawning (Barry et al., 1990; Malison et al., 1994).
Recently, T was reported to positively regulate the expression of reproductive genes (FSHb, LHb, and GnRHr) in maturing Atlantic cod (Gadus morhua) pituitaries (von Krogh et al., 2017). We also show that plasma T in female L. japonicus peaks just prior to the final maturation of oocytes and ovulation, as in the reproductive cycle of other fishes (Holland et al., 2000; Mylonas & Zohar, 2007). Thus, seasonal plasma E 2 and T profiles of female L. japonicus may be used as indicators of their reproductive cycle.
Some of the male L. japonicus developed spermatozoa in the lumen of the lobules during November, when most females are previtellogenic. However, the spermatogenic activity was very high during winter. Spermiation began in December and peaked in January. Spermatids and spermatozoa started to degenerate in March, and there was no spontaneous spermiation in April. Spermatogenesis resumed in autumn and ended at the onset of spring. The plasma T levels significantly increased between spermatogenesis and spermiation and decreased at spermiation termination. This pattern was also seen in carp Cyprinus carpio (Barry et al., 1990), rainbow trout Oncorhynchus mykiss (Liley et al., 1986), and white suckers Catostomus commersoni (Scott et al., 1984). Plasma T, the levels of which vary according to reproductive stage, induces the production of GTH by the pituitary and acts as a precursor of 11-KT, thus stimulating reproductive behavior in male fish (Stacey et al., 1986; Ouchi et al., 1988; Matsuyama et al., 1991). In some teleosts, increases in plasma T have been observed during maturation (Scott et al., 1984; Liley et al., 1986; Truscott et al., 1992), suggesting that T may play an indirect role in the control of spermatogenesis or spermiation (Prat et al., 1990; Jackson & Sullivan, 1995; Holland et al., 2000). Although spermiation is believed to be primarily regulated by specific progestins, such as 17,20β-dihydroxy-4-pregnen-3-one and 17,20β,21-trihydroxy-4-pregnen-3-one, it has been reported that spermatogenesis in fish is regulated by T. The levels of this hormone vary throughout the reproductive cycle and reach a maximum at the onset of spermiation (Woods & Sullivan, 1993). Significant increases in plasma T were also detected during the reproductive cycle of L. japonicus, similar to blue cod Parapercis colias (Pankhurst & Conroy, 1987) and European sea bass Dicentrarchus labrax (Prat et al., 1990). The peaks occurred at the resting stage (between June and July), and again between the spermatogenic stage and spermiation (between November and January). The first peak was not as dramatic as the second. However, we could not clarify why this peak appeared at this particular time; this phenomenon should be further studied to better understand reproductive patterns of male sea bass.
Stressors can affect fish reproduction, according to their nature and severity (Schreck, 2010). Moving or handling fish, and changing their environmental conditions, induces stress and inhibits their reproductive activity (Contreras-Sánchez et al., 1998; Haddy & Pankhurst, 2000; Pankhurst NW, 2011). Therefore, moving fish from an outdoor net cage into an indoor tank may influence their reproduction. However, the results of this study indicate that sea bass acclimatized and underwent gonadal development within their first year of moving. A spawning monitoring experiment showed that the fecundity of L. japonicus without a stressor is 536,000±74,000 eggs/kg BW (mean±SEM), and is positively related to body length and BW. In addition, the spontaneous maturation and initial spawning time of female L. japonicus were affected by salinity. The number of spawned eggs and egg quality were both higher in low salinity seawater than in normal seawater. Although we did not examine the effect of salinity on plasma steroid levels or gonad development, we found that lowering salinity could positively affect spawning in L. japonicus. While there is relatively little information on the effects of salinity on ovarian recrudescence and reproductive physiology in fish, it has been reported that salinity affects female maturation in some species (Tamaru et al., 1994; Haddy & Pankhurst, 2000; Paixão et al., 2013). However, because the initiation and maintenance of vitellogenesis in fish appear to be more dependent on the classical cues of photoperiod and temperature rather than salinity (Duston & Bromage, 1987; Okuzawa et al., 1989; Biswas et al., 2010), future studies should examine the effects of salinity on ovarian recrudescence and reproductive physiology.
We conclude that the final maturation of L. japonicus, after transportation from outdoor net cages to an indoor facility, occurs between December and February, and spawning takes place between February and March. We also found that female sea bass reared at lower salinity are able to produce more eggs, which could be successfully fertilized for further seedling production.