INTRODUCTION
There are two different strains of the Oncorhynchus mykiss species, anadromous and land-locked strains (Moyle, 2002). Steelhead trout is an anadromous strain of O. mykiss and is also called steelhead salmon (more biological perspective) due to its salmon-like life history (Quinn & Myers, 2004). In Korea, landlocked domesticated strain of O. mykiss has mainly been cultured in freshwater but recently seawater farming of the O. mykiss has been attracting more interest due to the larger harvest sizes in sea cages (Shin et al., 2011). Aquaculture of steelhead has been attempted in an effort to improve seawater acclimation of O. mykiss (Xiong et al., 2020). Although steelhead trout is generally known to be more readily adaptive to seawater than rainbow trout (Wagner, 1974; Prunet & Boeuf, 1985; Johnsson & Clarke, 1988; Xiong et al., 2019), specific information on elements affecting seawater adaptability of steelhead trout is crucial for the seawater transfer to be successful.
Parr-smolt transformation (also called smoltification) is a series of developmental changes juvenile anadromous salmonids undergo, during which the juvenile salmon acquires hypoosmoregulatory capacity to survive in salt water before seaward migration (McCormick & Saunders, 1987; Hoar, 1988; Boeuf, 1993; Bjӧrnsson et al., 2011). The complete parr-smolt transformation usually includes morphological (silvering), behavioral (pelagic schooling), and physiological (hormonal and enzymatic) changes (Hoar, 1976; McCormick & Saunders, 1987; Hawkings et al., 2004; Riley et al., 2014). Changes in environmental factors (photoperiod, temperature, and salinity) trigger parr-smolt transformation in nature when juvenile fish reach a threshold size (Conte & Wagner, 1965; Ewing et al., 1979; Bjӧrnsson et al., 2011; Kendall, 2015), which widely varies depending on the species (Clarke, 1982; Varnavsky et al., 1991). Some studies have reported that development of seawater adaptability in O. mykiss occurs before the manifestation of smoltification (Conte & Wagner, 1965; Wagner, 1974). These studies suggest that parr-smolt transformation and development of seawater adaptability may be two distinct physiological processes (Conte & Wagner, 1965; Wagner, 1974). Furthermore, seawater adaption of steelhead trout is reported to be a function of size rather than chronological age (Houston, 1961; Conte & Wagner, 1965). Thus, when steelhead trout reach a threshold size, they can acclimate to seawater independent of smoltification (Wagner, 1974; Morro et al., 2019; Xiong et al., 2020).
In many O. mykiss farms in Korea, juvenile fish produced in freshwater are geographically separated from grow-out in sea cages, similar to salmon farming in the Yellow Sea of China (Xiong et al., 2020). Thus, if fish farmers were able to transfer smaller juveniles, they would have more advantages in terms of transportation as well as stress levels in fish. Although O. mykiss can adapt to a wide range of environmental salinity, when O. mykiss are transferred from freshwater to seawater, they immediately undergo water efflux (dehydration) and sodium influx. To regain internal hydromineral balance, after drinking seawater O. mykiss selectively excrete excess salts (particularly Na+ and Cl−) through their gills and retain water (Kültz, 2015). Na+/K+ ATPase (NKA, sodium pump) in chloride cells of the gills play a major role in the excretion of excess Na+ and Cl− from the body. High NKA activity is an indicator of seawater adaptability of salmonids including O. mykiss (Bystriansky et al., 2006; Kennedy et al., 2007). However, if O. mykiss are transferred from freshwater to seawater before they develop efficient osmoregulatory capacity, the fish cannot increase NKA activity to a sufficient level, which results in prolongable elevation of salts (e.g., plasma Na+ and Cl−) and dehydration (e.g., muscle moisture decrease) internally, which could lead to the interruption of normal biochemical processes and physiological homeostasis (Bystriansky et al., 2006; Kennedy et al., 2007; Kültz, 2015). In a previous study, Gorie (1993) has reported that 0+ landlocked strain of O. mykiss need to reach a 150 g size to acquire a high level of hypoosmoregulatory capacity in seawater. In the current study, we hypothesized that under-yearling steelhead trout acquire a high level of seawater adaptability in a relatively smaller size compared to landlocked rainbow trout. The objective of the current study was to determine seawater adaptability of under-yearling steelhead in three different sizes trout for optimal seawater transfer. The 96 h seawater challenge test included determination of plasma Na+ and Cl− concentration, muscle moisture content, and NKA activity as indicators of seawater adaptability (Leray et al., 1981; Clarke, 1982; Madsen & Naamansen, 1989; Morro et al., 2019).
MATERIALS AND METHODS
Fries of steelhead trout strain (Troutlodge, WA, USA) were purchased and reared in 300-liter freshwater recirculating tanks for 5 months in a laboratory at Sejong University, Seoul (temperature: 15.29±0.06°C; photoperiod: 12L: 12D; dissolved oxygen: 7.73±0.04 mg/L; pH: 7.86±0.02; conductivity: 992.67±27.13 μS/cm). During the rearing period, fish were fed a commercial diet (protein 45%, lipid 12%–13%, ash 14%, fiber 3%) at a daily optimal ration of total body mass, twice a day (Austreng et al., 1987). 10%–20% of the water was changed daily with dechlorinated filtered freshwater and additional aeration was provided to each tank. All experimental procedures using fish followed the guidelines approved by the Institutional Animal Care and Use Committee of Sejong University, Korea.
The average body weight of fish and seawater parameters are presented in Table 1. As the batch of fish grew, three separate 96 h seawater challenge tests were carried out in chronological order ( June, August, and November of 2019 for 50, 100, and 150 g size groups, respectively). For each test, 39 fish of similar size were selected and randomly transferred to 3 separate recirculating rectangular glass aquaria (300 liters of seawater), which resulted in 13 fish per aquarium (triplicate). All fish were fasted from 24 h before being transferred to seawater (32 ppt, Table 1) until the end of the test (96 h after the transfer to seawater). At 0, 24, 48, and 96 h after the transfer, three fish from each aquarium (9 fish per each sampling time) were randomly caught, euthanized in a buffered tricaine methanesulfonate (MS-222, 200 mg/L), and weighed. After blood was collected from the caudal vein using syringes and heparinized tubes, plasma was separated by centrifugation at 13,000 g, 4°C for 15 min and then stored at −80°C for plasma Na+ and Cl− analyses. Six to seven gill filaments from the second arch were surgically removed, put in cold SEI buffer (250 mM sucrose, 10 mM Na2EDTA, 50 mM imidazole, pH 7.3), and instantly frozen on dry ice and stored at −80°C. White muscle without skin was surgically removed from the muscle mass between the dorsal fin and the lateral line and stored at −80°C.
Plasma Na+ and Cl− concentrations were determined using an automated blood chemistry analyzer (Dri-CHEM 7000i, Fuji, Tokyo, Japan).
The ouabain sensitive hydrolysis of adenosine triphosphate (ATP) leads to the oxidation of nicotinamide adenine dinucleotide (NADH), by pyruvate kinase and lactate dehydrogenase (LDH), which is directly measured in a microplate reader at 340 nm (McCormick et al., 1993). Levels of NKA activity were determined based on previously established protocol (McCormick et al., 1993). A frozen gill sample in SEI buffer was thawed briefly and homogenized on ice using a motorized homogenizer (Power masher II, Nippi, Tokyo, Japan) in SEID buffer (0.5 g of sodium deoxycholate in 100 mL of SEI) for 20 seconds. After centrifugation (5,000 g, 4°C, 1 min), supernatant was used for the assay: 10 μL of homogenate to each of the four wells for every sample. The remainder of the homogenate was saved and later analyzed for protein concentration (BCA protein assay, Pierce, Thermo scientific, Rockford, IL, USA). 200 μL of assay mixture A (4 U LDH/mL, 5 U pyruvate kinase/mL, 2.8 mM phosphoenolpyruvate, 0.7 mM ATP, 0.22 mM NADH, and 50 mM imidazole, pH 7.5)+salt mixture was added to two wells and 200 μL of assay mixture B (assay mixture A+0.5 mM ouabain)+salt mixture was added to the other two wells for each sample. The microplate was put into a plate reader (25°C, Epochi 2 microplate reader, BioTek) and the decrease of NADH was measured at 340 nm for 10 min. All assay procedures were completed within 30 min of homogenization. NKA activity was calculated by the difference in ATP hydrolysis in the presence and absence of ouabain as micromoles of ADP per mg of protein per hour. All chemical reagents were purchased from Sigma-Aldrich, St. Louis, MO, USA, unless noted otherwise.
All data was statistically analyzed using SPSS Statistics 24 (IBM, Chicago, IL, USA). A two-way ANOVA model was used to test the main effects of size and time with the interaction term. Interaction between the size and time was also tested based on the statistical significance of the interaction term in the ANOVA analyses. The assumptions (homogeneity of variance and normality) were tested before the ANOVA analyses. For post-hoc multiple comparison, Duncan’s multiple range test was performed for comparison among size groups and time points. Statistical differences were determined at a 0.05 probability level, and all results were denoted as mean±SE of the mean unless noted otherwise.
RESULTS AND DISCUSSION
The seawater challenge (direct transfer to seawater) test has been widely considered as a good indicator of seawater adaptability of juvenile salmonids (Wagner, 1974; Clarke, 1982; Johnsson & Clarke, 1988; Madsen & Naamansen, 1989; Bystriansky et al., 2006). The seawater adaptability of under-yearling steelhead trout was evaluated by determining the plasma Na+ and Cl− levels, muscle moisture, and gill NKA activity for 96 h after direct transfer to seawater. Despite the minimal mortality of all size groups, other parameters showed significant effects of size group and time (0, 24, 48, and 96 h) after transfer.
The average body weight, mortality, and water parameters during the 96 h seawater challenge test are presented in Table 1. The overall mortality was minimal (Table 1). No mortality was observed in the 100 g weight group, while mortality in both the 50 and 150 g weight groups was 2.6% (Table 1). In a previous study of a landlocked O. mykiss strain, Gorie (1993) reported a 29% mortality in the 50 g size class compared to 3% and 6% mortality in 100 and 150 g size classes, respectively, 96 h after seawater transfer. In comparison with the previous study by Gorie (1993), under our experimental conditions the current mortality results after seawater transfer indicate that steelhead trout has a higher tolerance to seawater (32 ppt) than landlocked O. mykiss of the same size. This agrees with the 96-h LC50 salinity test results reported by Johnsson & Clarke (1988). The current low mortality in steelhead trout suggests that 50 g size fish may have developed at least partial seawater tolerance, which corresponds to the results on development of seawater adaptation in juvenile steelhead trout ( Johnsson & Clarke, 1988; Ward & Slaney, 1988; Shin et al., 2011).
Plasma Na+ and Cl− levels during the 96 h seawater challenge test are presented in Fig. 1. Two-way ANOVA analyses with the interaction terms showed a significant weight group effect (p<0.05; Table 2) and a time effect (p<0.05; Table 2) but did not show a significant interaction between the weight group and time (p>0.05; Table 2) for the both plasma Na+ and Cl− concentrations (Fig. 1A & B). Plasma Na+ levels in the 50 g size group increased to 180 mmol/L 48 h after the transfer to seawater and reached almost 190 mmol/L after 96 h (Fig. 1A). However, plasma Na+ levels in the 100 g size group increased to 165 mmol/L 24 h after seawater transfer and then leveled off later (Fig. 1A). Plasma Na+ levels in the 150 g size group increased to about 170 mmol/L 24 h after seawater transfer and then leveled off as well (Fig. 1A). Overall, although plasma Na+ levels in both the 100 and 150 g size groups reached a plateau after 24 h after seawater transfer, those in the 50 g size group continued to increase until 96 h (Fig. 1A). Also, the plasma Na+ levels in the 50 g size group were significantly higher than those of the 100 or 150 g size groups 96 h after seawater transfer (p<0.05, Fig. 1A). Plasma Cl− levels in the 50 g size group rapidly increased to about 150 mmol/L 24 h after the transfer and then gradually increased up to 160 mmol/L after 96 h (Fig. 1B). However, plasma Cl− levels in the 100 g size group increased to around 142 mmol/L 24 h after seawater transfer and then leveled off later (Fig. 1B). Similarly, plasma Cl− levels in 150 g size group reached a plateau after 24 h and then leveled off (Fig. 1B). The plasma Cl− level in the 50 g size group was significantly higher than that in 100 or 150 g size groups 96 h after seawater transfer (p<0.05, Fig. 1B).
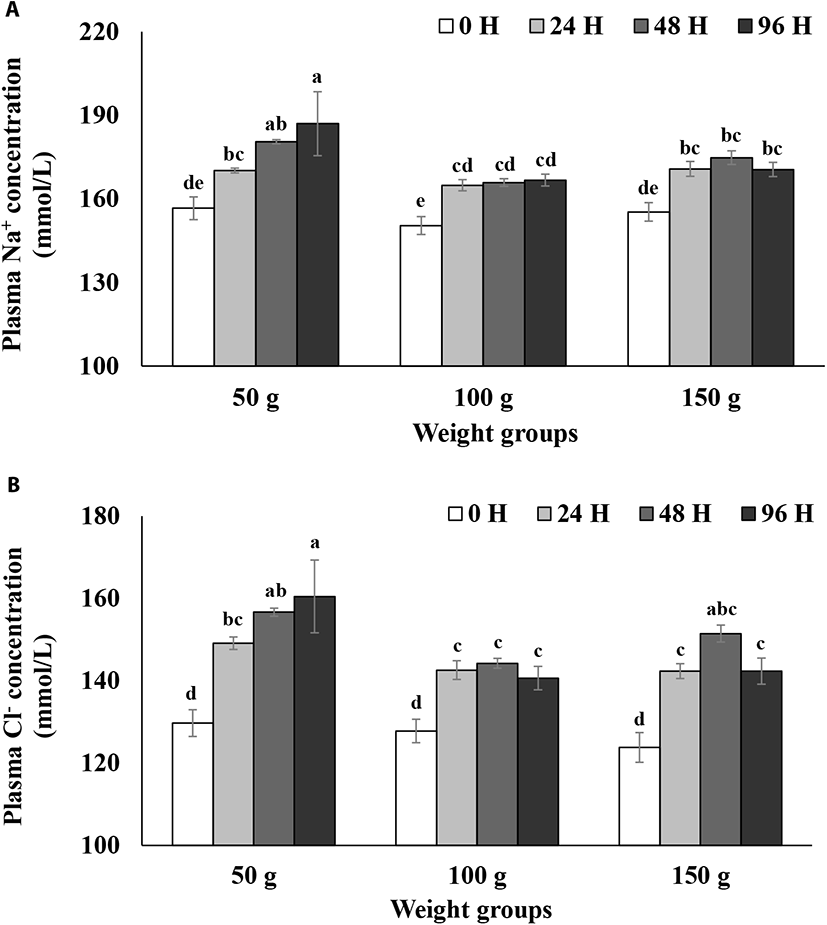
Bath & Eddy (1979) reported that rainbow trout (mean weight: 13.3 g) did not survive for more than 2 days in seawater (33 ppt), but showed increased mortalities and complete adaptation within 7–10 days after abrupt transfer to two-thirds seawater. Bath & Eddy (1979) concluded that increased plasma Na+ and Cl− concentrations in the adaptation phase is the result of initial increased drinking and diffusing of salts into the gills. In a previous study, Gorie (1993) showed that landlocked rainbow trout over 150 g size develop high level hypoosmoregulatory capacity, but trout of 50 and 100 g sizes did not develop a high level. Thus, in comparing the steelhead trout with its landlocked counterpart, steelhead trout seems to develop a higher level of hypoosmoregulatory capacity at a smaller size than landlocked rainbow trout in terms of plasma ionic regulations. The current results on plasma Na+ and Cl− level results suggest that while steelhead trout over 100 g size have a high level of hypoosmoregulatory capacity, steelhead trout at 50 g size did not develop a high level of the capacity.
Skeletal muscle moisture contents during the 96 h seawater challenge test are presented in Fig. 2. A two-way ANOVA analysis was performed to test for the main effects of seize and time with the interaction term (Table 2). Muscle moisture contents in the 100 g size group did not show significant decrease during 96 h after the transfer to seawater (p>0.05; Fig. 2). However, muscle moisture content in the 150 g size group decreased significantly between 48 h to 96 h after seawater transfer (p<0.05; Fig. 2). Moisture contents in 50 g size group rapidly decreased to about 75% 24 h after seawater transfer and did not recover until 96 h (p<0.05; Fig. 2). Although there was a significant decrease in muscle moisture contents in the 150 g size group, the percentage of the decrease was relatively small (less than 1.5%) (Fig. 2). Thus, steelhead trout in the 100 and 150 g size groups seem to maintain moisture contents relatively well compared to the 50 g size group. The current results on muscle moisture changes in 50 g steelhead trout after the seawater challenge are comparable to the results on the changes in 150–340 g rainbow trout at the same time after transfer to seawater (32 ppt, 13°C) reported by Leray et al. (1981). The rainbow trout in a 200 g size class maintained significantly higher levels of muscle moisture content after the transfer to seawater than did the other smaller size classes (Gorie, 1993). Again, in comparison to the previous studies on rainbow trout reported by Leray et al. (1981) and Gorie (1993), steelhead trout in the current study seem to be able to maintain muscle moisture better than landlocked rainbow trout of the similar size.
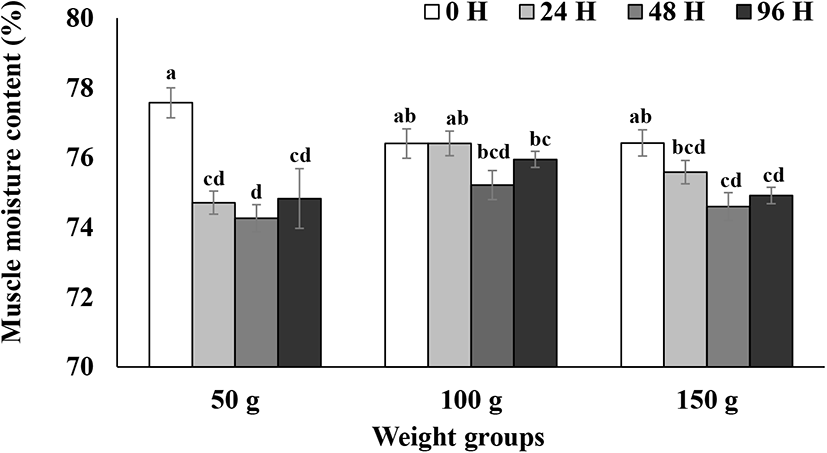
Gill NKA activity during the 96 h seawater challenge is presented in Fig. 3. Gill NKA activity in the 100 g size group started to significantly increase from 48 h after seawater transfer until the end of test (Fig. 3). Gill NKA activity in the 50 and 150 g size groups did not show significant increases until 96 h after the transfer (Fig. 3). Gill NKA activity in the 100 g size group was significantly higher than that of the 50 and 150 g size groups 24, 48, and 96 h after seawater transfer (p<0.05, Fig. 3). Seawater adaptation capacity increased with the increase in the size of juvenile O. mykiss (Conte & Wagner, 1965; Wagner, 1974; Johnsson & Clarke, 1988). O. mykiss that develop high hypoosmoregulatory capacity are also known to possess the ability to upregulate NKA allowing them to pump out excess Na+ and Cl− in response to increment of environmental salinity (Bystriansky et al., 2006; Kennedy et al., 2007). In the current study, 100 g size steelhead trout showed high seawater adaptability by increasing NKA levels in response to the direct transfer to seawater, whereas 50 g size steelhead did not show any upregulation of NKA levels, which in turn suggest an underdeveloped seawater adaptability of 50 g size steelhead trout (Bystriansky et al., 2006; Kennedy et al., 2007). On the other side, steelhead trout of the 150 g size showed the overall lower NKA levels than those of the other size groups and did not show any increase of NKA level in response to seawater transfer (Fig. 3), which can be attributed to the previous finding that the development of capacity to upregulate gill NKA to distinct high levels for hypoosmoregulation is related to growth rate (Ewing et al., 1980). Ewing et al. (1980) reported that a growth rate of less than 0.027 cm/day can prohibit a peak of gill NKA activity in Chinook salmon. In the current study, three seawater tests for each size group were separately conducted in chronological order as described in the material and method section. Steelhead trout in the 150 g size showed a much lower grow rate compared to the other two size groups, which may be why the gill NKA activity was much lower compared to the other groups and did not respond to the abrupt salinity change in the present study.
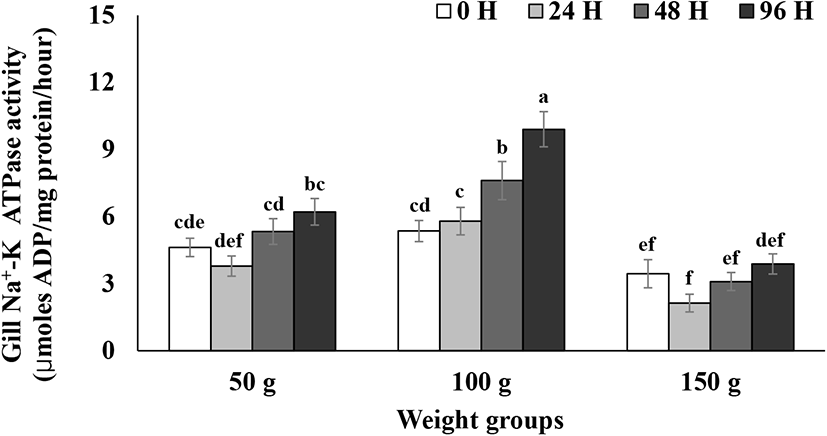
In conclusion, in the consideration of plasma Na+ and Cl− levels, muscle moisture content, gill Na+/K+ ATPase activity, and mortality, steelhead trout of 50 g size were found to have not yet developed a high level of hypoosmoregulatory capacity, whereas fish of 100 and 150 g sizes showed a high level of hypoosmoregulatory capacity after direct transfer to seawater (32 ppt). Therefore, steelhead trout larger than a 100 g size is recommended for transfer to seawater culture. In the future, study on optimization of acclimation method (regime) warrant further research.