INTRODUCTION
Lipopolysaccharide (LPS), also known as an endotoxin, is a large molecule consisting of a lipid and a polysaccharide. LPS is found in the outer membrane of Gram-negative bacteria, and elicits strong immune responses in mammals (Raetz & Whitfield, 2002). Several lines of evidence demonstrate that LPS challenge profoundly affects reproductive function. In male rats, intraperitoneal injection of LPS induced oxidative stress that leads to functional damages in the testicular mitochondria, and eventually, impairment of spermatogenesis (Aly et al., 2012). Also, direct injection of LPS into the caput region of the rat epididymis induced epididymitis, and this can decrease the expression of epididymal beta-defensins (SPAG11E), causing the impairment of sperm motility (Cao et al., 2010).
In female rats, LPS affects in vitro secretion of sex steroid hormones from both theca-interstital cells and granulosa cells (Taylor & Terranova, 1995; Taylor & Terranova, 1996). Systemic treatment with LPS and prolactin significantly increased the number of atretic follicles in proestrus ovaries and increased the apoptotic cells and macrophages (Besnard et al., 2001). In addition, prenatal or neonatal LPS exposure significantly delayed the day of vaginal opening (VO) in female rats (Izvolskaia et al., 2016; Cardoso et al., 2009), These studies suggest that LPS might affect the ovarian steroidogenesis and folliculogenesis. However, the exact mechanism underlying the adverse effect of LPS on the female reproduction is not clearly understood yet.
In the present study, we hypothesized that LPS treatment during adolescence can change the timing of puberty onset which is associated with altered ovarian steroidogenesis. To explore the hypothesis, we used prepubertal female rats and chronic LPS treatment model, and measured transcriptional activities of steroidogenesis related genes in ovary.
MATERIALS & METHODS
Timely pregnant Sprague-Dawley rats were obtained from Han-Lim Animal (Gyunggi-do, Korea) and reared in Sangmyung University animal facility under conditions of 12-h light/dark cycle (lights on at 07:00 h) and constant temperature of 22±1°C. During pregnancy and lactation, the mothers had free access to normal chow and tap water (ad libitum). All procedures used were approved by the Animal Care and Use Committee at Sangmyung University in accordance with guidelines established by the Korea Food and Drug Administration (KFDA).
The day after weaning, female dams were randomly assigned to control and LPS-treated group (n=6-8/group) and their body weights were checked from PND 25 until sacrifice. Rats were treated chronically (for 5 days, from PND 25 -29) with LPS (from Escherichia coli 0128:B12, Sigma-Aldrich, Korea) which was dissolved in sterile saline. LPS (50 μg/kg body weight; injection volume, 0.3 mL) were daily injected (ip) at 09:00 to treated animals and control animals with saline (0.9%). The date (age) at VO was evaluated through daily visual examination. At PND 39, animals were sacrificed and the tissues (ovary, uterus, oviduct, vagina, adrenal, spleen and thymus) were immediately removed and weighed at 18:00.
Seminal Vesicle tissue specimens were fixed 4% paraformaldehyde at 4°C for 24h. Fixed tissues were serially dehydrated in graded ethanol and xylene. Specimens were embedded in paraffin block. The tissues blocks were cut at 6 μm using microtome (HM350S, MICROM, Germany). Sections were stained with hematoxylin-eosin stain and examined under light microscope (BX51, Olympus, Japan).
Total RNAs were isolated from tisssue samples using the single-step, acid guanidinum thiocyanate-phenol-chloroform extraction method. Total RNAs were used in RT-PCR reactions carried out with Maxime™ RT PreMix (InTron, Korea) and Accupower PCR Premix (GeneAll, Korea) according to the manufacturer’s instructions. Sequences of the primers and the specific PCR conditions used in this study are listed in Tables 1 and 2, respectively. The reaction products were analysed by gel electrophoresis in 1.5% agarose gel, 75V 65 min and visualized by ethidium bromide staining. The band intensities were measured using the image analysis system (Imager III -1D main software, Bioneer, Korea).
All values are expressed as the means (±S.E.). Differences between control and treatment groups were analysed by Student’s t-test. P values less than 0.05 were considered significant. The IBM PC programs INSTAT and PRISM 3.0 (GraphPad, San Diego, CA, USA) were used to calculate and plot the results.
RESULTS
There was no difference in the body weights between the LPS-treated and their control animals from PND 25 until PND 38 (Fig. 1). On the other hand, immunological challenge by LPS during PND 25-29 delayed VO (Controls vs LPS-treated group = 37.50±0.83 vs 39.50±1.37, P<0.05), as clearly shown in Fig. 2. The day all the animals exerted VO was PND 41.
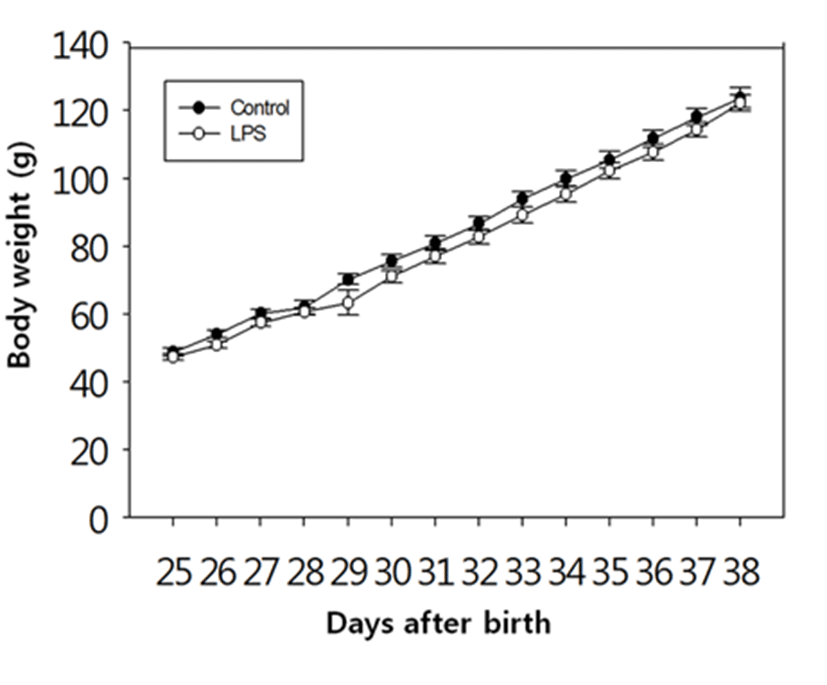
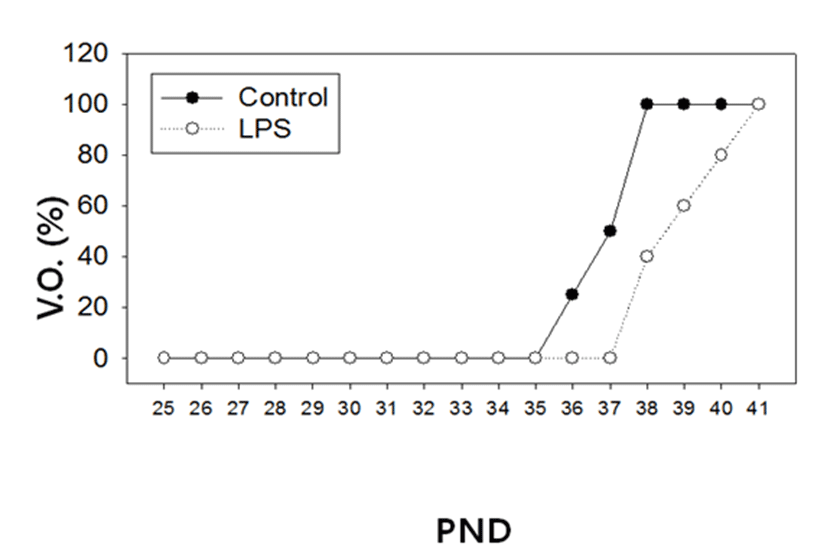
In order to evaluate a potential adverse effect of LPS on the reproductive organs, we measured the tissue weights of ovary, uterus, oviduct and vagina; Adrenal, spleen and thymus weights served as non-reproductive organs (Table 3). The animal sacrifice was scheduled at PND 39, since the majority of control animals failed to show VO while all LPS-treated animals exerted VO on the date (Fig. 2). Among the reproductive organs, the weights of the ovaries from LPS-treated animals were significantly lower than those of control animals (12.7±0.5 mg vs 19.5±1.4 mg, p<0.001). Likewise, the weights of the oviduct from LPS-treated animals were significantly lower than those of controls (4.8±0.3 mg vs 6.5±0.3 mg, p<0.001). However, there were no changes in the weights of uterus and vagina between the LPS-treated and their control animals. Non-reproductive tissues such as adrenal, spleen and thymus failed to show any weight difference between controls and LPS-treated animals.
To access the histological changes in LPS-exposured ovaries and uteri, standard paraffin section and hematoxylin eosin staining method were employed (Fig. 3). Multiple corpora lutea were found in the control ovaries, indicating ovulations were occurred in this animals. However, none of corpus luteum was present in LPS-treated ovary; instead, several degenerating (atretic) and cyst-like follicles were found. Uteri of LPS-treated animals shown the slightly thickened endometrial and myometrial layers and less-developed luminal structures compared to control uteri.
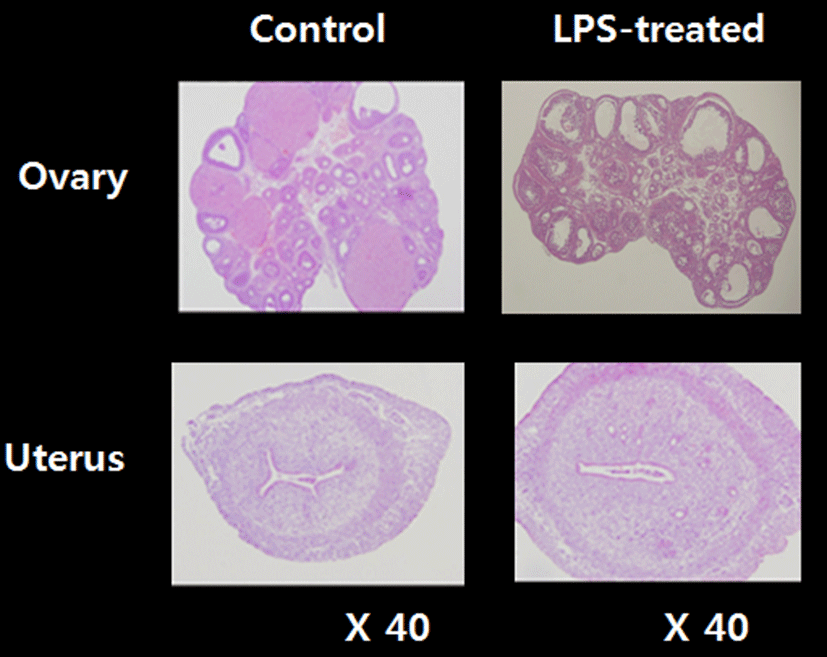
To address the relationship between the delayed puberty onset and the ovarian steroidogeneic activities in the LPS-exposured rats, we employed semi-quantitative RT-PCRs. Fig. 4A shows that the transcription level of steroidogenic acute regulatory protein (StAR) was significantly increased by LPS treatment (Control vs LPS-treated group = 1.0±0.3 A.U. vs 1.5±0.4 A.U., p<0.05). StAR is a transport protein that regulates cholesterol transfer within the mitochondria, which is the rate-limiting step in the production of steroid hormones. Likewise, the transcription levels of CYP11A1 (Fig. 4B, Control vs LPS-treated group = 1.0±0.4 A.U. vs 4.6±0.6 A.U., p<0.001). CYP17A1 (Fig. 4C, Control vs LPS-treated group = 1.0±0.2 A.U. vs 5.3±1.7 A.U., p<0.05), CYP19 (Fig. 4F, Control vs LPS-treated group = 1.0±0.2 A.U. vs 4.5±1.6 A.U., p<0.05) were significantly increased by LPS treatment. CYP11A1 is a cholesterol side-chain cleavage enzyme, commonly referred to as P450scc, and this is a mitochondrial enzyme that catalyzes conversion of cholesterol to pregnenolone. CYP17A1, also called cyto-chrome P450 17A1 or steroid 17α-hydroxylase, is a key enzyme in the steroidogenic pathway that produces progestins, mineralocorticoids, glucocorticoids, androgens, and estrogens. CYP19, also called aromatase, is responsible for the aromatization of androgens into estrogens.
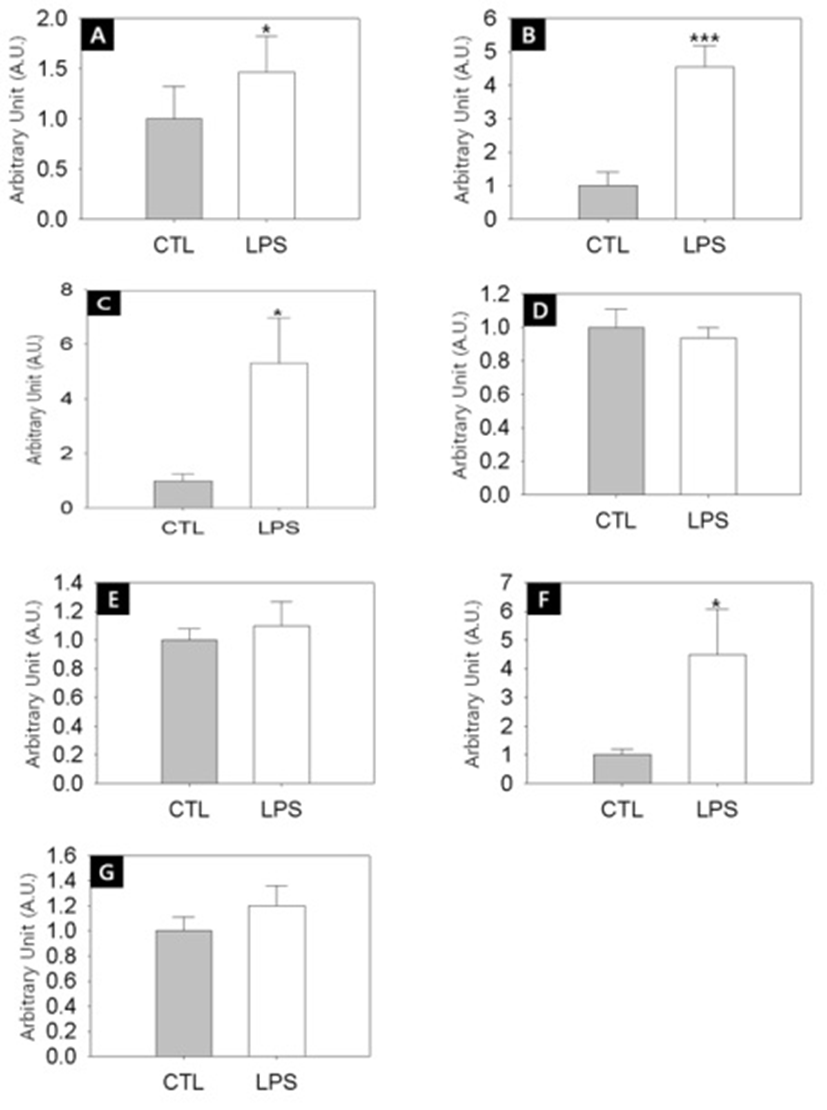
On the other hand, the transciption levels of 3β-HSD (Fig. 4D), 17β-HSD (Fig. 4E) and LH receptor (Fig. 4G) were not changed by LPS challenge. 3β-HSD (3β-Hydro-xysteroid dehydrogenase/Δ5-4 isomerase) is an enzyme that catalyzes the biosynthesis of progesterone from pregnenolone. 17β-HSD (17β-Hydroxysteroid dehydrogenases) is a key enzyme that catalyze the final steps of the steroid biosynthesis.
DISCUSSION
In the present study, we demonstrate that the immune challenge by prepubertal LPS injection resulted in delayed puberty onset and significant decreased transcriptional activities of the steroidogenesis-related genes in rat ovary. Previously published researches also shown that the delayed VO, a widely accepted vital sign of puberty onset, was observed in female rats with different LPS-exposure timimg; in utero (Izvolskaia et al., 2016), neonatal (Knox et al., 2009), neonatal and postwean (Ozgocer et al., 2015) and postwean (Cardoso et al., 2010). The methodological differences of LPS exposure between ours and the Cardoso et al. (2010) were number of dosage [PND 25-29 (daily) vs PND 25, 27 and 29], strains of animal (Sprague Dawley vs Wistar) and serotypes (Escherichia coli 0128:B12 vsSalmonella typhosa). Different exposure time and duration, dose, and origin of bacterial strains can elicit differential immunological response (Appelmelk et al., 1993). However, based on the studies including our own, it is reasonable that LPS might be a highly potent immune agent which can induce delayed puberty onset in female rats with a wide range of effectiveness.
It is well known that immune stress affects the reproductive system and could act at the different levels of the hypothalamic–pituitary–gonadal axis (HPG), most of all at the level of the brain (Herman et al., 2010). In the brain, LPS might play a suppressive role on the GnRH neuronal activity through the stimulation of the negative inputs such as opioid and GABA. Administration of LPS inhibited steroid-induced LH release and reduced the Fos expression in GnRH neurones, and pretreatment with LPS inhibited naloxone-induced LH release in adult female rats (He et al., 2003). In the following study, LPS significantly increased the content of glutamic acid decarboxylase 67 (GAD67), a GABA-synthesising enzyme, in the medial preoptic area (mPOA), and this LPS challenge completely eliminated the steroid-induced LH surge (Akema et al., 2005). The authors suggested that LPS stimulates GABA synthesis in preoptic neurones, which in turn inhibits the LH surge in female rats. This idea was supported by the facts that intraperitoneal LPS administrations during prepubertal period significantly increased GnRH and glutamate contents but decreased GABA content in the hypothalamus of peripubertal female rats (Cardoso et al., 2010). According to the authors, the tissue contents inversely correlated with the release of GnRH/glutamate/GABA. LPS can also acts on the hypothalamic kisspetin neurons, an upstream modulator of GnRH pulse generator. Neonatal LPS exposure resulted in the down-regulation of Kiss1 expression in the mPOA of prepubertal females rats, suggesting that the mPOA population of kisspeptin neurones play a pivotal role in controlling the onset of puberty, and that their function can be affected by neonatal stress such as immune challenge (Knox et al., 2009).
There are enough evidence to prove both of direct and indirect LPS actions on ovary. Systemic LPS treatment (over a period of 6 days) induced a state of constant dioestrus and decreased circulating concentrations of progesterone and estradiol, and resulted in significantly fewer large preovulatory follicles in adult rats (Shakil et al., 1994). In another systemic treatment study, LPS and prolactin significantly increased the number of atretic follicles and increased the mean number of apoptotic cells and macrophages in proestrus ovaries (Besnard et al., 2001). In the two classic in vitro studies, treatment of rat theca-interstital cells with LPS led to a dose-dependent decrease in LH-stimulated progesterone and androstenedione secretion (Taylor & Terranova, 1995), and treatment of rat granulosa cells with LPS led to a significant dose-dependent decrease in LH-stimulated estradiol accumulation in culture media (Taylor & Terranova, 1996). The authors also demonstrated that the LPS had no significant effect on LH receptor level, and suggested that the effect appears to be restricted to the LH-stimulated aromatization of androgens to estrogen. LPS, therefore, appears to have a direct suppressive effect in ovarian functions, possibly via an inhibitory action on steroidogenesis (Shakil et al., 1994). Our findings that chronic LPS exposure elicits the changes in the expressions of steroidogenesis-related genes in rat ovary support this hypothesis.
It is intriguing that the expression of the steroidogenesis- related genes are differentially regulated in brain, testis and adrenal with tissue-specific manner. LPS administration induced similar oxidative damages and altered several gene expressions in the brain and testis of adult male rats; the mRNA expression of 3β-HSD and 17β-HSD was increased in the brain with significant decrease in the testis in LPS treated animals (Sadasivam et al., 2014). The authors also demonstrated that the LPS treatment completely represses androgen receptor co-repressor (ARR19) in the brain while not in the testis, and suggested that the opposite expression patterns in the brain and testis are attributed to the tissue-specific expression of ARR19. Similar to this, our results show that the expression levels of ovarian 3β-HSD and 17β-HSD remained unchanged in LPS-exposured rats. In mice study, no change in StAR mRNA was detected in the testis while increments of StAR protein and mRNA in the adrenal (Hales et al., 2000). Our finding that increase of ovarian StAR expression by LPS treatment is close to adrenal pattern rather than testis one.
In conclusion, the present study demonstrated that the repeated LPS exposure during the prepubertal period induced multiple alterations in the steroidogenic machinery in ovary, and resulted in delayed puberty onset. Delayed puberty is tightly connected with reduced level of sex steroids at an appropriate age, short stature and decreased self-esteem. Although malnutrition is probably the most important mechanism responsible for delayed puberty, chronic illness is accompanied by a delay in growth and the pubertal growth spurt, and therefore, early diagnosis is essential and appropriate and specific therapy fundamental (Pozo & Argente, 2002). In that sense, prepubertal LPS challenge model is useful to understand the consequences of chronic or frequent bacterial infection in early life and the reciprocal regulation of immune (stress) - reproductive function.