INTRODUCTION
A chronic hyperglycemic condition has widespread adverse effects on various tissues, including the heart, kidney, retina, muscle, liver, and vasculature (Rask-Madsen et al., 2013; Bissel, 2015). Emerging evidence indicates that the functions and structure of the lung are also affected by diabetes mellitus (DM). Decline in total lung capacity, forced vital capacity, and diffusion capacity has been reported in patients with DM and may contribute to increased risk for pulmonary diseases (Ehrlich et al., 2010; Pitocco et al., 2012; Kolahian et al., 2019). Recent studies of DM patients and diabetic animal models demonstrated that DM induces fibrotic changes that accompany an epithelial-to-mesenchymal transition in the lung, which is mediated through the activation of transforming growth factor (TGF)-β signaling pathways (Talakatta et al., 2018). Gene expression profiling in the lung of diabetic rats revealed altered expression of specific sets of genes related to apoptosis, the stress response, and collagen (Lunteren et al., 2014). However, the molecular mechanism underlying these physiological and gene expression changes in diabetic lung tissues, and the therapeutic strategies that may be derived from them, remain to be elucidated.
Administration of mesenchymal stem cells (MSCs) is one promising strategy for the treatment of diabetic complications (Rasaei et al., 2020). Increasing evidence demonstrates that perivascular stem cells (PVCs), known as a precursor of MSCs, exhibit regenerative potential in various disease models, including traumatic brain injury, uterine injury, Achilles tendon rupture, muscle atrophy, and spinal cord injury (Cao et al., 2017; Park et al., 2020). More recently, we demonstrated that PVCs regulate hematopoietic differentiation in a development-specific manner and suppress inflammasome activation during inflammatory responses in murine and human macrophages (Kim et al., 2019; Jeong et al., 2020). These findings suggest that PVCs could be a therapeutic option for treating diabetic complications. In fact, PVCs were found to accelerate wound healing in a diabetic rat and partially rescued hyperglycemia-induced alterations in bone marrow hematopoietic composition (Kim et al., 2018). However, the therapeutic efficacy of PVCs on physiological and gene expression changes in the diabetic lung has not been reported. In the present study, we aimed to determine gene expression changes in the mouse lung due to streptozotocin (STZ)-induced DM. Furthermore, we evaluated the effects of human PVCs on gene expression changes in the diabetic lung.
MATERIALS AND METHODS
C57BL/6J mice were purchased from Dooyeol Biotech (Seoul, Korea) and housed in a specific pathogen-free facility. All animal experiments were approved by the Institutional Animal Care and Use Commitment of Kangwon National University (KW-180809-2). Male C57BL/6J mice (20–22 g, 8 to 10 weeks) were intraperitoneally injected with 50 mg/kg STZ (S0130, Sigma-Aldrich, St. Louis, MO, USA) daily for 5 consecutive days to induce type 1 DM. After induction of diabetes, mice were intravenously administered with PVC-CM (40 μg/100 μL) daily for 6 weeks.
Immunofluorescence staining was performed as previously described (Kim et al., 2019). For immunofluorescence staining, 4-μm thick lung sections were dewaxed with xylene and rehydrated with a gradient of ethanol. The sections were subjected to antigen retrieval with a citrate buffer bath (pH 6) at boiling temperature and then blocked for endogenous peroxidase activity. After rinsing with phosphate buffered saline (PBS), the sections were incubated with primary antibodies against α-SMA (Santa Cruz Biotechnology, SC-53015), Zbtb16 (LS Bio, LS-c334349), Adrb2 (Abcam, ab182136), and Amigo2 (LS Bio, LS-c404504) overnight at 4°C. The sections were washed with PBS and incubated with secondary GFP- or RFP-labelled antibodies for 30 min. Nuclei were counterstained with DAPI (Abcam, ab104139). Immunofluorescence images were captured by fluorescence microscopy (IX-51, Olympus, Tokyo, Japan).
Total RNA was extracted from lung tissues using the RNeasy Mini Kit (Qiagen, 74106, Valencia, CA, USA). RNA purity and integrity were evaluated using the Agilent 2100 Bioanalyzer (Agilent Technologies, Santa Clara, CA, USA). Microarray analysis has been performed as previously reported (Kim et al., 2017).
Total RNA was extracted from lung tissues using an RNeasy Mini kit (Qiagen, Duesseldorf, Germany) and cDNA was synthesized using the TOPscrip™ RT DryMIX (Enzynomics, Daejeon, Korea). PCR amplification was performed using a Step One Plus real-time PCR system (Applied Biosystems, Warrington, UK) with TOPreal™ qPCR 2X PreMIX (Enzynomics). Relative expression was normalized against GAPDH expression by the ΔΔCt method. Sequences of primers used in this study are listed in Table 1.
Human PVCs were obtained from human umbilical cords as previously described. This study was approved by the institutional review board of Kangwon National University Hospital (2014-06-003-010). All participants provided written informed consent. PVC-CM was prepared as previously described ( Jeong et al., 2020). Briefly, PVCs were incubated with serum-free α-MEM for 24 hrs and filtered through a 0.22 μm filter. Filtered medium was concentrated using an Amicon Ultra-15 Centrifugal Filter Units (Millipore) and stored at −80°C until use.
RESUTLS
To investigate the histological and gene expression changes in the lungs following long-term exposure to hyperglycemic conditions, a diabetic mouse model was established in which STZ (50 mg/kg) was administered for 5 consecutive days. Fig. 1A). Histological analysis showed that hyperglycemia resulted in alveolar wall thickening and a reduction in alveolar air space (Data not shown). We next investigated global gene expression in the lungs of STZ-induced diabetic mice with or without treatment of PVC-conditioned medium (CM). Unsupervised hierarchical clustering showed that the global expression patterns of lung tissue were significantly altered by long-term hyperglycemic condition and partially reversed by PVC-CM treatment (Fig. 1B). A total of 172 genes (72 upregulated and 100 downregulated) were significantly altered in the STZ group compared with the control. There were also 39 differentially expressed genes (DEGs) (23 upregulated and 16 downregulated) found in the lungs of the STZ group compared with the STZ+PVC-CM group (Table 2). To compare the biological relevance of these DEGs, gene ontology (GO) analyses were performed using total expression data from control, STZ, and STZ+PVC lungs. The main GO categories that included DEGs between the control vs STZ groups were response to cellular process, response to stimulus, and programmed cell death. In addition, a number of general GO terms were identified, including 39 that were associated with differential expression between the STZ and STZ+PVC-CM groups. Of these GO terms, two were characterized by genes related to immune processes and regulation of responses to stimulus (Fig. 1C). These results indicate that long-term hyperglycemia induces gene expression changes in lung tissues that can be partially reversed by PVC treatment.
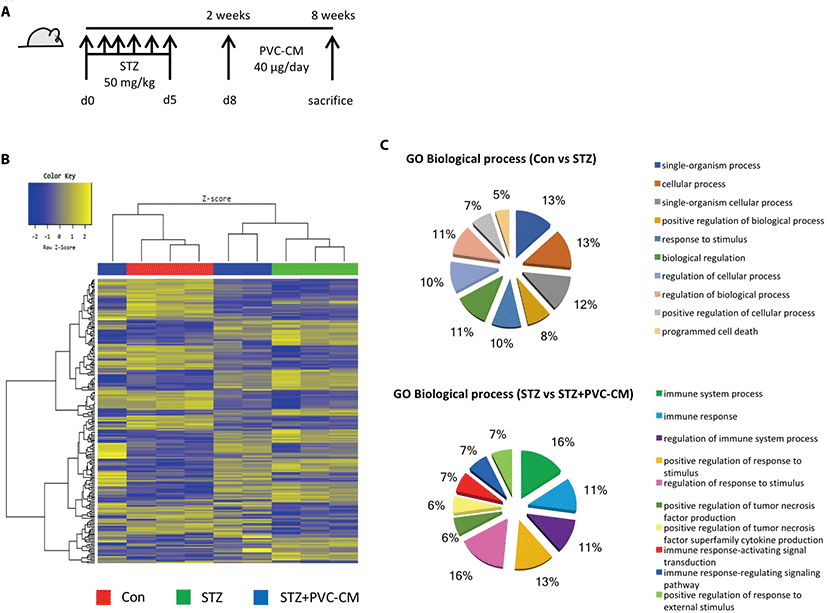
PVC, perivascular stem cell; STZ, streptozotocin; fc, fold change.
To identify potential target genes associated with hyperglycemia-induced changes in lung tissues, we first selected 19 up- and downregulated genes from the STZ group where expression could be reversed by PVC-CM treatment (Fig. 2A). Among the group of upregulated genes, qPCR analysis confirmed that the expression pattern of adhesion molecule with IgG-like domain 2 (Amigo2), beta 2-adrenergic receptor (Adrb2), and zinc finger and BTB domain containing 16 (Zbtb16) were identical between the cDNA microarray data (Fig. 2B). Moreover, the Klk1b22 gene was upregulated significanty in the STZ group compared to the control but not reversed by PVC-CM treatment (Fig. 2B). Immunostaining of lung tissues demonstrated that Adrb2 and Ztbt16 were localized mainly to the apical membrane of bronchioles, while Amigo2 was detected primarily in endothelial cells of pulmonary vessels (Fig. 3). Consistent with the PCR results, hyperglycemic conditions stimulate enhanced expression of these proteins. These findings suggest that hyperglycemia mediates cell type-specific regulation of Adrb2, Amigo2, and Ztbt16, which could be useful indicators of diabetic injury in lung tissues.
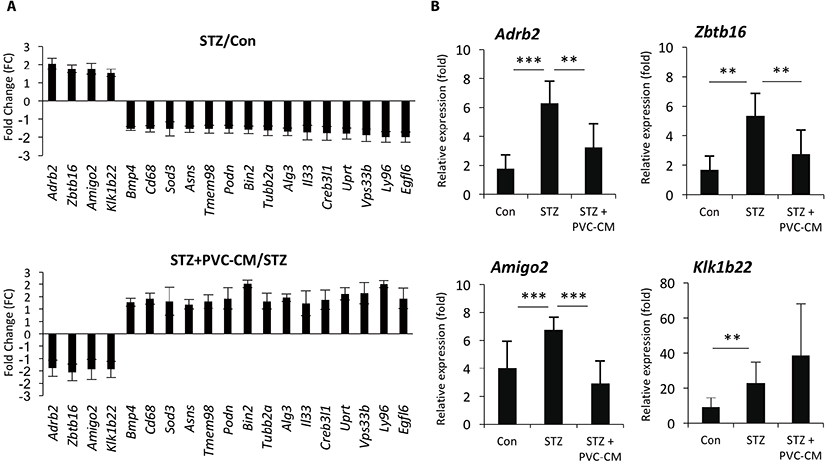
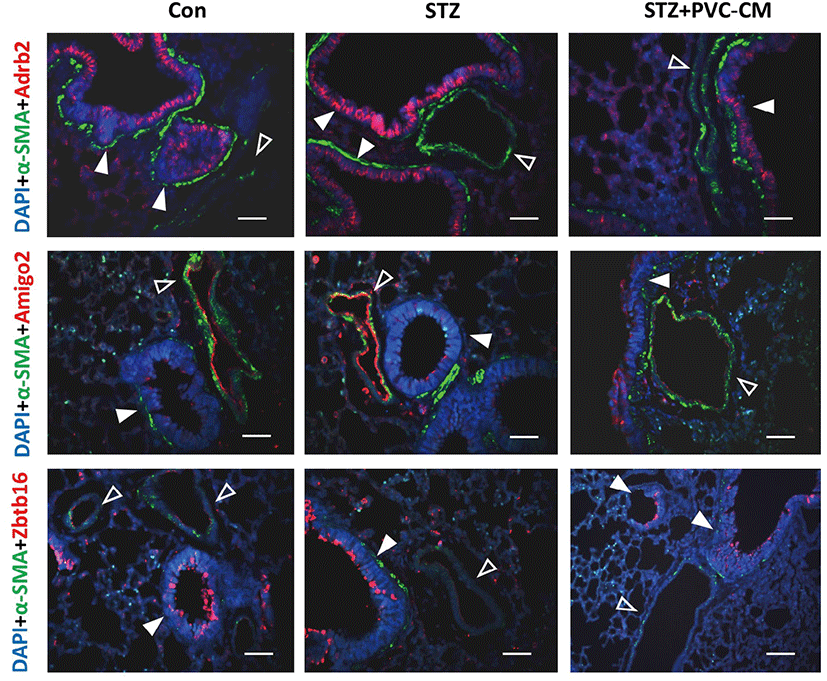
DISCUSSION
In the present study, we found that long-term hyperglycemia induced alterations in gene expression, as well as histological changes, in the lung. The number and extent of gene expression changes in diabetic lung tissue were moderate compared with findings in other diabetic tissues, including in the kidney, skeletal muscle, and lens, suggesting that the lung is more resistant to hyperglycemic stress than other organ tissues. Importantly, we found that PVCs could partially reverse hyperglycemia-induced gene expression changes in lung gene expression, thereby demonstrating that they can be an alternative for treating diabetic complications in various tissues, including the lung.
This study demonstrated that Adrb2, Amigo2, and Ztbt16 are hyperglycemia-regulated genes in the lung. Although a few studies have reported whether the function and expression of these genes are associated with histological and physiological changes in diabetic lung tissue, evidence suggests that these genes are potentially useful as prognostic markers to predict lung injury in a diabetic state (Prior et al., 2011; Al-Goblan et al., 2014; Park et al., 2015). Accumulating evidence has demonstrated that Adrb2 is expressed in many cell types in the lung, and it has been considered a critical pharmacologic target in the management of asthma and chronic obstructive pulmonary disease (COPD) due to a correlation between Adrb2 polymorphisms and reduction of lung function (Kim et al., 2009). In addition, significantly higher levels of ADRB2 mRNA were observed in the bronchial mucosa of patients with severe COPD and asthma compared with those displaying mild disease. Given the strong relationship between DM and lung diseases, investigation of downstream signaling activation of Adrb2 and its polymorphisms in diabetic lung tissue may provide a better understanding of the role of this gene in terms of its physiological and gene expression changes in diabetic lung tissue. Long-term hyperglycemia leads to reduced lung function by increasing vascular permeability, suggesting that DM could elevate the risk of tumor metastasis. DM also increased immune cell infiltration in multiple organs and promoted inflammation, mediated by altered expression of several adhesion molecules, including intercellular adhesion molecule 1, vascular cell adhesion molecule 1, and P-selectin, on endothelial cells to augment leukocyte binding (Gu et al., 2013; Pawelczyk et al., 2017; Rasaei et al., 2020). Amigo2, an anti-apoptotic adhesion molecule, is known to be expressed in endothelial cells and involved in angiogenesis and vascular remodeling in pathological situations. Thus, upregulation of Amigo2 in pulmonary vessel endothelial cells of diabetic lung tissue could be involved in promoting influx of immune cells and angiogenesis. These findings suggest that targeting Adrb2 and Amigo2 may provide opportunities for the development of novel strategies to prevent or ameliorate hyperglycemia-induced lung injury.
In this study, we identified hyperglycemia-regulated genes in the diabetic mouse lung. However, it remains to be explored whether these genes play a crucial role in developing pulmonary complications and are regulated in the lungs of DM and pulmonary fibrosis patients.