INTRODUCTION
The epididymal fat pad is located between the testis and caput epididymis and is a part of gonadal white adipose tissue in male (Niemalä et al., 2008). The appearance of the epididymal fat-like structure is detectable at the birth, even though the mature adipocyte in epididymal fat during postnatal development is observed at 14 days of postnatal age (Han et al., 2011). Postnatal development of the epididymal fat is associated with gene expression of adipogenic genes, such as perilipin, peroxisome proliferator activated receptor 𝛾, and adipocyte protein 2 (Han et al., 2011). During the postnatal perioid, the mass and size of the epididymal fat become continuously increased, and cellular composition of the epididymal fat is changed (Cleary Margot et al., 1977; Björntorp et al., 1979; Frühbeck 2008). Based on histochemical aspects and/or relative to the location of spermatic blood vessels, the epididymal fat is divided into two or three different parts (Tirard et al., 2007; Altintas et al., 2011), and differential expression patterns of various adipocytokines, including leptin, adiponectin, and resistin, among different epididymal fat parts has been reported during postnatal period (Altintas et al., 2011; Lee 2019; Lee & Kim, 2019).
Adipose tissue is a well-known endorine organ which produces a number of adipocytokines and enzymatic factors influencing on the development and function of target tissues (Kershaw & Flier, 2004). The functional role of epididymal fat has not been examined in detail yet. When the part of epididymal fat is surgically removed, the spermatogenesis in the young adult hamster is disrupted along with decreases of testis mass and sperm number, even though the serum level of testosterone has not been affected (Chu et al., 2010). Our previous research has shown the possible role of epididymal fat on expressional regulation of testicular steroidogenic enzymes (Lee & Lee, 2023). The surgical lipectomy of epididymal fat (EPFX) at different postnatal age has given an effect on expression of a number of steroidogenic enzymes in differential manners (Lee & Lee, 2023). For example, The EPFX at 2 months of postnatal age results in significant increases of expression levels of steroidogenic enzymes, while expression of these testicular enzymes with the EPFX at 5 months of postnatal age are decreased (Lee & Lee, 2023). Interestingly, the EPFX at 8 months of postnatal age has not affected expression of testicular steroidogenic enzymes (Lee & Lee, 2023). In addtion to the effect of EPFX on the testis, differential expression of various adipocytokines in the epididymal fat during postnatal period suggest that the epididymal fat-derived factors would influence on the expression of testicular steroidogenic enzymes in complicated and differential manners at different postnatal ages.
A functional role of the testis is the production of testosterone which is required for normal spermatogenesis and masculinity. The testosterone is an androgenic component which exerts its biological function through the binding with androgen receptor (AR). In addition, the testosterone is further metabolized into two potent steroid hormones, dihydrotestone (DHT) or estrogen, by the action of 5α-reductases or cytochrome P450 aromatase, respectively (Luu-The, 2013). The DHT or estrogen usually binds to its corresponding intracellular receptor, AR or estrogen receptors (ERs, ERα and ERβ), present within cells and modulates expression of target genes (Luu-The, 2013). The presence and localization of 5α-reductase type 1, cytochrome P450 aromatase, AR, and ERs within the testis have been extensively determined (Goyal et al., 1997; Luu-The, 2013). The expressional regulation of these genes by adipocytokines has been reported from other researches. Masarwi et al (2018) have shown stimulatory effect of leptin on expression of P450 aromatase and ER in the growth plate. The deficiency of leptin in the mouse has resulted in down-expression of ERs in the testis (Martins et al., 2017). However, expressional regulation of these genes in the testis by the epididymal fat-derived molecules has not been determined.
In the present research, expressional changes of testicular 5α-reductase 1, P450 aromatase, AR, and two ERs α and β genes by partial EPFX were examined at several postnatal ages. A quantitative real-time PCR analysis was employed to evaluate expression levels of these genes in the mouse testis at 2, 5, 8, and 12 months of postnatal ages.
MATERIALS AND METHODS
The C57BL/6 male mice at 7 weeks of postnatal age were acquired from Samtako (Osan, Korea) and individually housed at the arrival and for the entire experimental period. The food and water were supplied ad libitum, and a total of 29 mice were used for the present research.
The animals at 2 months of postnatal age were randomly divided into four age groups, 2, 5, 8, and 12 months of postnatal ages. The each age group was divided into two subgroups, a sham (no lipectomy performed) and a epididymal fat lipectomy (EPFX) group. Thus, there were a total of 8 experimental groups for the current study, including a sham (n=4) and an EPFX (n=4) group at 2 months of postnatal age, a sham (n=3) and an EPFX (n=3) group at 5 months of postnatal age, a sham (n=3) and an EPFX (n=4) group at 8 months of postnatal age, and a sham (n=4) and an EPFX (n=4) group at 12 months of postnatal age.
For the EPFX, the animal was first anesthetized with ether and hold on a warm heating pad. The testis and epididymial fat were exposed through an incision on each scrotum, and the epididymal fat was removed with a pair of fine scissors, as much as possible, but a careful handling was required to minimize the disruption of the blood system supplied to the testis. Most of the proximal epididymal fat part was removed sugically by the EPFX, and the scrotum incision was sealed with instant glue (Aron Alpha Instant glue, Toagosei, Tokyo, Japan) after the testis and rest of epididymal fat were pulled back into the scrotum. The same surgical procedure, except the lipectomy step, was performed for a sham animal. With a confirmation of full recovery from the surgery, the mouse was placed to own cage and carefully monitored for any abnormal behaviors and casualty for 2 weeks before the isolation of testis. All procedures were performed in accordance with protocols approved by the Eulji University Animal Care and Use Committee (EUIACUC 20-04).
At the 14th day after the EPEX, the animal was euthanized by cervical dislocation, and the testis and epididymal fat were taken from the animal. The tissues were quickly placed in cold PBS solution, and the testis was separated from the rest and quickly frozen in liquid nitrogen. The testis was stored in –80°C freezer until used for total RNA isolation.
The piece of testis was homogenized in Trizol reagent (Molecular Research Center, Cincinnati, OH, USA). Sequantial reactions with chloroform and isoproanol were carried out to isolate and obtain total RNA pellet. After washing in 70% EtOH once, the pellet was air-dried in room temperature and then resuspended in DEPC-dH2O. The quantitative measurement and qualitative examination of isolated total RNA was accomplished by NanoDrop Lite spectrophotometer (Thermo Scientific, Waltham, MA, USA) and 1.2% agarose gel electrophoresis, respectively.
One microgram of total RNA was utilized to construct the first-stranded complementary DNA (cDNA) by iScripTM Reverse transcription Supermix for reverse transcription (RT)-qPCR (Bio-Rad Laboratories, Hercules, CA, USA). The RT reaction was carried out in the final volume of 20 μL in a following condition, 5 min at 25°C, 20 min at 46°C, and 1 min for 95°C. Qantitative real-time PCR was directly performed with the generated cDNA.
To perform quantitative real-time PCR, a 25 μL mixture of PCR was prepared with 1 μL of cDNA, 10 pmol of each oligonucleotide primer, 7 μL of iQTM SYBR® Green Supermix (Bio-Rad Laboratories), and nuclease-free dH2O to adjust to a total reaction volume. Expression levels of 3-oxo-5α-steroid 4-dehydrogenases type 1 (Srd5a1), a.k.a. cytochrome P450 5α-reductase I, cytochrome P450 aromatase (Cyp19a1), androgen receptor (Ar), estrogen receptor α (Esr1), and estrogen receptor β (Esr2) were examined. The information of primers used for quantitative real-time PCR is presente in Table 1. As an internal control for PCR analysis, glyceraldehyde-3-phosphate dehydrogenase (Gapdh) was utilized. The PCR was carried out a pre-denaturation stage at 95°C for 5 min, cycles of a denaturation stage at 95°C for 30 sec, an annealing stage at Tm for 30 sec, and an extension stage at 72°C for 30 sec, and an additional extension stage at 72°C for 10 min in PTC-200 Chromo 4 real-time system (Bio-Rad Laboratories). The PCR products were checked by 1.2% agarose gel electrophoresis in size.
The RT reactions and real-time PCRs were independently triplicated to acquire a mean value for an experimental animal. The expression levels of molecules examined were normalized with that of Gapdh to get a mean and standard error of a given experimental group. The data are presented in the relative ratios of transcript levels between tested molecule and Gapdh. Statistical comparison between sham and EPFX groups at each postnatal age was determined by the Student t-test, as considered significant if p<0.05.
RESULTS
The EPFX at 2 months of postnatal age resulted in a significant increase of Srd51 transcript level in the testis (Fig. 1A). But, expression level of Srd5a1 was significantly decreased by the EPFX at 5 months of postnatal age (Fig. 1A). There was no significant change of Srd5a1 mRNA level in the testis by the EPFX at 8 months and 12 months of postnatal ages (Fig. 1A).
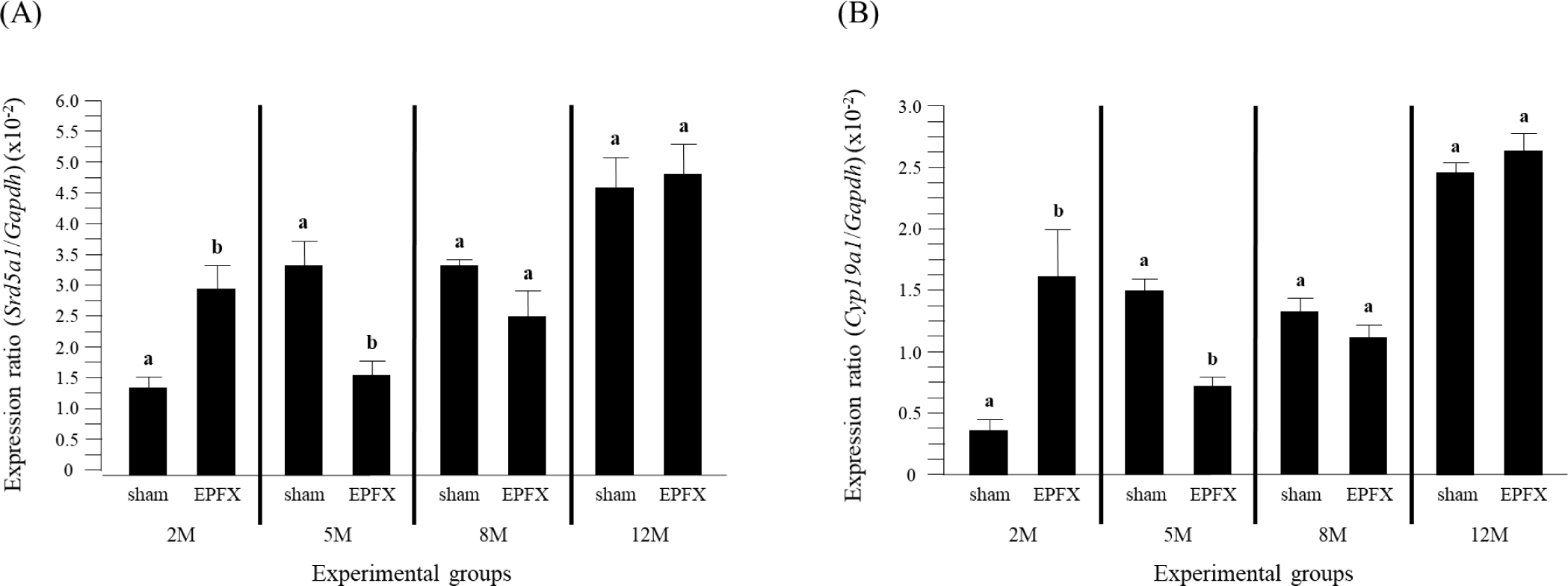
Fig. 1B shows the effects of the EPFX at different postnatal ages on the levels of testicular Cyp19 transcript. A drastic increase of Cyp19 expression was found in the EPFX group at 2 months of postnatal age, while the transcript level of Cyp19 was significantly decrease in the EPFX group at same postnatal age (Fig. 1B). The EPFX at 8 months and 12 months of postnatal ages didn’t influence expression of Cyp19 in the testis (Fig. 1B).
The expression level of testicular Ar was significantly increased by the EPFX at 2 months of postnatal age, even though a notable decrease of Ar transcript level was found in the testis treated with the EPFX at 5 months of postnatal age (Fig. 2A). The EPFX at 8 months of postnatal age didn’t give an effect on expression level of Ar in the testis (Fig. 2A). A similar finding was observed at 12 months of postnatal age (Fig. 2A).
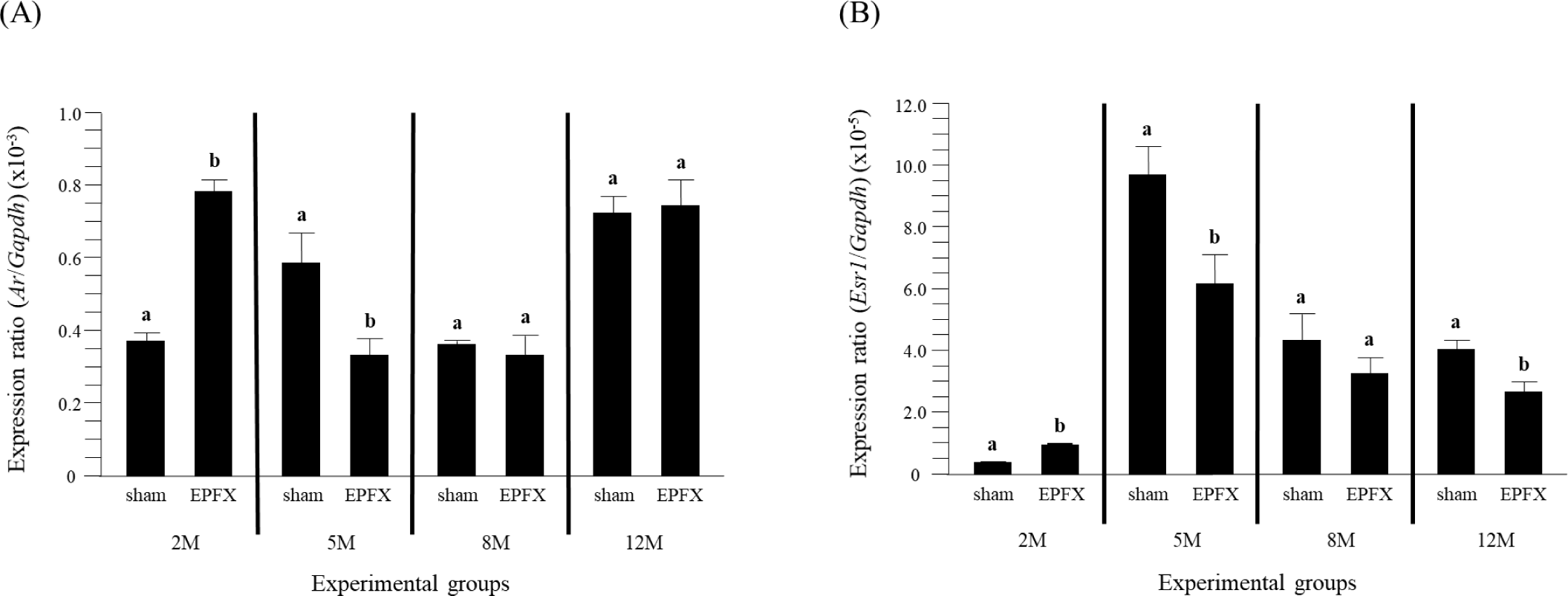
The abundance of Esr1 transcript was significantly increased in the testis treated with the EPFX at 2 months of postnatal age (Fig. 2B). However, a decrease of Esr1 mRNA level was detected in the testis of EPFX group at 5 months of postnatal age (Fig. 2B). Like other molecules examined, there was no significant change of testicular Esr1 transcript level in EPFX group at 8 months of postnatal age (Fig. 2B). Interestingly, expression level of testicular Esr1 in the EPFX group at 12 months of postnatal age was significantly lower that that in sham group at same postnatal age (Fig. 2B).
The level of Esr2 transcript in the testis was significantly increased by the EPFX at 2 months of postnatal age (Fig. 3). However, unlike other molecules, the abundance of testicular Esr2 at 5 months of postnatal age was not affected by the EPFX (Fig. 3). In addition, the EPFX did not cause any change of expression level of Esr2 in the testis at 8 months and 12 months of postnatal ages (Fig. 3).
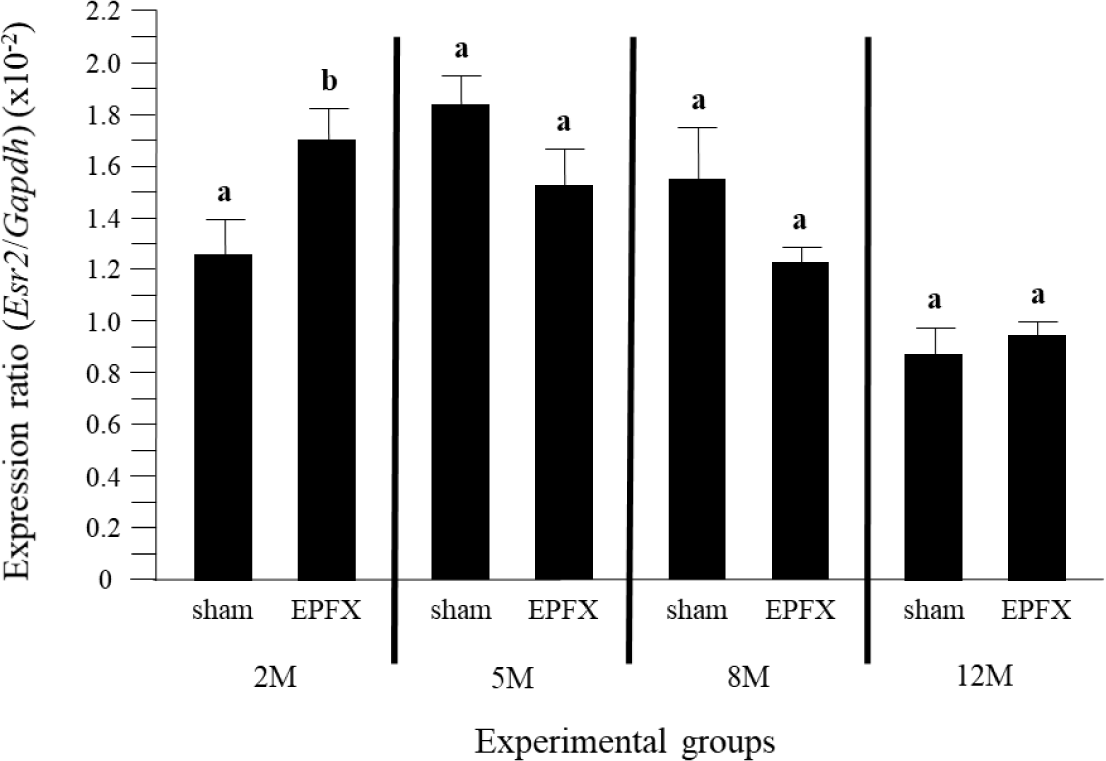
DISCUSSION
The current study has attempted to examine the effect of EPFX at different postnatal age on expression of testicular Srd5a1, Cyp19, Ar, Esr1 and Esr2. The EPFX at 2 months of postnatal age results in significant increases of transcript levels of all examined molecules. However, expression of testicular Srd5a1, Cyp19, Ar, and Esr1, except Esr2, is decreased by the EPFX at 5 months of postnatal age. The EPFX at 8 and 12 months of postnatal age does not influence on expression of any tested genes in the testis, except a significant decrease of Esr1 by the EPFX at 12 months of postnatal age.
Expression of a variety of adipocytokine in the epididymal fat has been examined by other and our previous studies. These adipocytokines present in the mouse epididymal fat include leptin, adiponectin, resistin, and tumor necrosis factor (TNF)-α (Altintas et al., 2011; Ye et al., 2013; Lee, 2019; Lee & Kim, 2019). In addition, our earlier reports have shown differential expression patterns of some adipocytokines in the epididymal fat, depending on postnatal age and/or distal or proximal part of the epididymal fat (Lee, 2019; Lee & Kim, 2019). For example, transcript level of leptin is gradually increased in the distal epididymal fat from 2 months to 12 months of postnatal age (Lee & Kim, 2019). But expression of leptin in the proximal epididymal fat is increased from 2 months to 8 months of postnatal age, followed by a significant decrease at 12 months of postnatal age (Lee, 2019). Expression of resistin in both distal and proximal epididymal fat is significantly increased at 8 months of postnatal age, even though the decrease of resistin mRNA level at 12 months of postnatal age is detected only in proximal epididymal fat (Lee, 2019; Lee & Kim, 2019). In addition, the deficiency of leptin gene induces the impaired steroidogenesis along with significantly decreased expressions of Ar, Esr1, and other steroidogenic enzymes (Martins et al., 2017). These findings suggest that expression of adipocytokines in the whole epididymal fat is likely controlled in more complicated manner than expected. Not only the expression but also actual secretion of leptin and resistin from the epididymal fat has been demonstrated by other researches (Nazian, 2013; Ye et al., 2013). Even though a growing body of evidence suggests that the epididymal fat could serve as an endocrine tissue, a biological role of the epididymal fact has not been caught an special attention.
Considering the proximity of the epididymal fat to the testis, it is logic to consider that epididymal fat-derived factors could give an influence on the function of the testis. The direct influence of the epididymal fat on the function of the testis has not been examined much. Chu et al. (2010) have demonstrated that the EPFX results in the abolition of sperm production and the cease of spermatogenesis, even though serum testosterone concentration is not affected by the EPFX. The effect of the EPFX at different postnatal age on expression of testicular steroidogenic enzymes has been examined in our previous research (Lee & Lee, 2023). Expression of steroidogenic enzymes in the testis, including steroidogenic acute regulatory protein, cytochrome P450 side chain cleavage, cytochrome P450 17α-hydroxylase, hydroxysteroid dehydrogenase 3, and hydroxysteroid dehydrogenase 17β type 3, is increased by the EPFX at 2 months of postnatal age, while the EPFX at 5 months of postnatal age results in decreases of transcript levels of steroidogenic enzymes in the testis (Lee & Lee, 2023). The data from the current study are quite similar with those of steroidogenic enzymes in the testis by the EPFX. The EPFX at 2 months of postnatal age causes increases of testicular Srd5a1, Cyp19, Ar, Esr1 and Esr2 transcript levels, but expression of these genes, except Esr2, is significantly reduced by the EPFX at 5 months of postnatal age. Together, the findings from these EPFX researches clearly suggest that the epididymal fat influences testis functions, most likely through modulation of expression of testicular molecules by the action of epididymal fat-derived factor(s). Further researches are required to define the epididymal fat-originated elements giving an impact on the testis functions.
The effects of adipocytokines on expression of Cyp19, Esr1, and Esr2 have been studied. The stimulatory effect of leptin on Cyp19 has been reported in the growth plate (Masarwi et al., 2018) and breast cancer cell (Kim et al., 2017). In addition, expression of Esr1 and Esr2 is up-regulated by leptin in the growth plate (Wang et al., 2012). Martins et al. (2017) show decreases of testicular Esr1 and Esr2 expression by the deficiency of leptin in the mouse. Expression of adipocytokines in the epididymal fat at different postnatal ages has been examined by our previous researches, and differential expression patterns of adipocytokines depend on the distal or proximal part of epididymal fat (Lee, 2019; Lee & Kim, 2019). For example, expression of leptin in the distal epididymal fat is continuously increased during postnatal period (Lee & Kim, 2019), while transcript level of leptin in the distal epididymal fat is increased until 8 months of postnatal age, with a transit decrease of leptin mRNA level at 12 months of postnatal age (Lee, 2019). Because others have found that leptin induces expression of Cyp19, Esr1, and Esr2, it is speculated that the EPFX at 2 months of postnatal age would result in a decrease of leptin amount produced from the epididymis fat and thus decreases of Cyp19, Esr1, and Esr2 transcript levels in the testis. However, the current study has shown unexpected observations, significant increases of Cyp19, Esr1, and Esr2 transcript levels in the testis by the EPFX. Interestingly, expression levels of Cyp19 and Esr1, but not Esr2, were decreased by the EPFX at 5 months of postnatal age. Based on these findings, it is difficult to define the regulatory effect of epididymal fat-derived leptin on expression of testicular Cyp19, Esr1, and Esr2. Moreover, even though the current research provides potential evidence of the function of epididymal fat on expressional regulation of various testicular genes, any epididymal fat-derived factor(s) directly acting on regulation of gene expression in the testis could not be specified from the present findings. Selective inhibition of expression of specific adipocytokine gene in the epididymal fat would inform the regulatory role of such adipocytokine on expressional regulation of target genes in the testis.