INTRODUCTION
G protein-coupled receptors (GPCRs) form the largest class of transmembrane cell surface receptors and regulate diverse intracellular signaling pathways in response to a wide range of extracellular stimuli (Lefkowitz & Shenoy, 2005; Lefkowitz et al., 2023). Gonadotropin receptors such as lutropin/choriogonadotropin receptors (LH/CGR) belong to a large superfamily of GPCRs with seven transmembrane-spanning domains and large extracellular and intracellular regions (Choi et al., 2023).
LH/CGRs mediate intracellular effects through G protein activation (α, β, and γ) (Min et al, 2019, 2020). These Gα subunits are characterized into four classes: Gαs, Gαi, Gαq, and Gα12 (Syrovatkina et al., 2016). Gαs and Gαi stimulate and inhibit adenylate cyclase, respectively, thereby regulating cyclic AMP responsiveness. LH/CGR is involved in the receptor-mediated cyclic adenosine monophosphate (cAMP) response (Tao et al., 2000; Bhaskaran & Ascoli, 2005; Byambaragchaa et al., 2021a) and the phosphorylation and activation of extracellular-related kinase (pERK1/2) (Martinelle et al., 2004; Tao, 2006; Shiraishi & Ascoli, 2007).
Equine choriogonadotropin (eCG) is a glycoprotein hormone secreted by trophoblastic cells into the bloodstream of pregnant mares during the 2nd to 5th months of pregnancy, with a peak level at 70–80 days of gestation (Min et al., 1996, 2004; Rodríguez et al., 2023). eCG consists of a common α-subunit and a hormone specific β-subunit (Min et al., 2019, 2020; Lee et al., 2021). A single gene encodes both the CG β-subunit and LH β-subunit in horses (Sherman et al., 1992; Min et al., 1996, 2004), and results in follicle-stimulating hormone (FSH)-like and luteinizing hormone (LH)-like response in non-equid species (Lee et al., 2021).
In previous studies, LH/CGR mutations have been reported, where activating mutants could trigger precocious puberty in boys, but inactivating mutants could cause anovulation in women and blocked sexual maturation in men (Thermmen & Huhtaniemi, 2000). These mutants were shown to be constitutively active in the absence of agonist stimulation, with basal cAMP level elevated up to several fold compared to the wild-type receptor (Shenker et al., 1993; Zhang et al., 2005, 2007; Galet & Ascoli, 2006; Latronico & Segaloff, 2007). Recently, we reported the structural and functional properties of equine LH/CGR activating and inactivating mutants (Byambaragchaa et al., 2021a) and equine follicle stimulating hormone receptor (FSHR; Byambaragchaa et al., 2022a).
Most GPCRs have several sites in their C-terminal regions that undergo modification and activate downstream signaling in response to agonist-receptor binding. These C-terminal modifications include phosphorylation (serine and threonine), highly conserved palmitoylation (two cysteines), and tri-leucine residues.
We reported that the C-terminal phosphorylation sites play a pivotal role in the signal transduction of equine FSHR (Seong et al., 2020) and equine LH/CGR (Byambaragchaa et al., 2022b). In eel LH/CGR and FSHR, we reported that activating mutants exhibited a large increase in the basal cAMP response without agonist stimulation (Byambaragchaa et al., 2021b). A C-terminal deletion mutant of eel FSHR led to a decrease in the maximal cAMP response (Kim et al., 2018).
In the present study, we demonstrated that constitutively activating mutants in eel LH/CGR exhibited a high fold increase in basal cAMP response, but inactivating mutants showed a low cAMP response under high concentrations of the recombinant equine chorionic gonadotropin (rec-eCG) agonist.
MATERIALS AND METHODS
Oligonucleotides were synthesized, and mutated DNAs was sequenced by Genotech (Daejeon, Korea). The pGEM-T Easy Cloning Vector was purchased from Promega (Madison, WI, USA). DNA ligation reagents, endonucleases, PCR reagents, and restriction enzymes were purchased from Takara Bio (Shiga, Japan). Mammalian expression vector pCORON1000 SP VSV-G was purchased from Amersham Biosciences (Piscataway, NJ, USA). The pcDNA3 mammalian expression vector, CHO-S cells, FreeStyle MAX reagent, and FreeStyle CHO expression medium were purchased from Invitrogen (Carlsbad, CA, USA). CHO-K1 cells were obtained from the Korean Cell Line Bank (Seoul, Korea). Ham’s F-12 medium, Opti-MEM I, and serum-free CHO-S-SFM II were purchased from Gibco (Grand Island, NY, USA). The cAMP homogeneous time-resolved fluorescence (HTRF) assay kit was purchased from Cisbio (Codolet, France). The QIAprep-Spin plasmid kit was purchased from Qiagen (Hilden, Germany) and disposable flasks were purchased from Corning (Corning, NY, USA). CentriPlus centrifugal filters were purchased from Amicon Bio Separation (Billerica, MA, USA). The PMSG enzyme-linked immunosorbent assay (ELISA) kit was purchased from DRG International (Mountainside, NJ, USA). All other reagents were purchased from Sigma-Aldrich (St. Louis, MO, USA).
To construct point mutations in eel LH/CGR activating and inactivating mutants, we used cDNA encoding full-length eel LH/CGR-wt using an overlap extension PCR strategy to substitute specific amino acids, as described previously (Byambaragchaa et al., 2021b). The PCR products were ligated into the pGEMT-Easy vector and the sequence of each mutant was confirmed by DNA sequencing. Full-length fragments were cleaved using the XhoI and EcoRI restriction enzymes and ligated into the pCORON SP VSVG mammalian expression vector.
The signal sequence region was removed from eel LH/CGR and the fragments were inserted under the VSVG-tag signal sequence of the mammalian expression vector. Finally, the direction of insertion was confirmed by restriction enzyme digestion. We constructed three activating mutants (eel LH/CGR-M410T, L469R, and D590Y), two inactivating mutants (eel LH/CGR-D417N and Y558F) in the previous studies (Byambaragchaa et al., 2021b), and one deletion mutant (eel LH/CGR-t641) at the C-terminal cytoplasmic tail region. Fig. 1 shows a schematic diagram of eel LH/CGR-wt and the mutants, denoting the amino acids of the conserved activation and inactivation sites in eel LH/CGR.
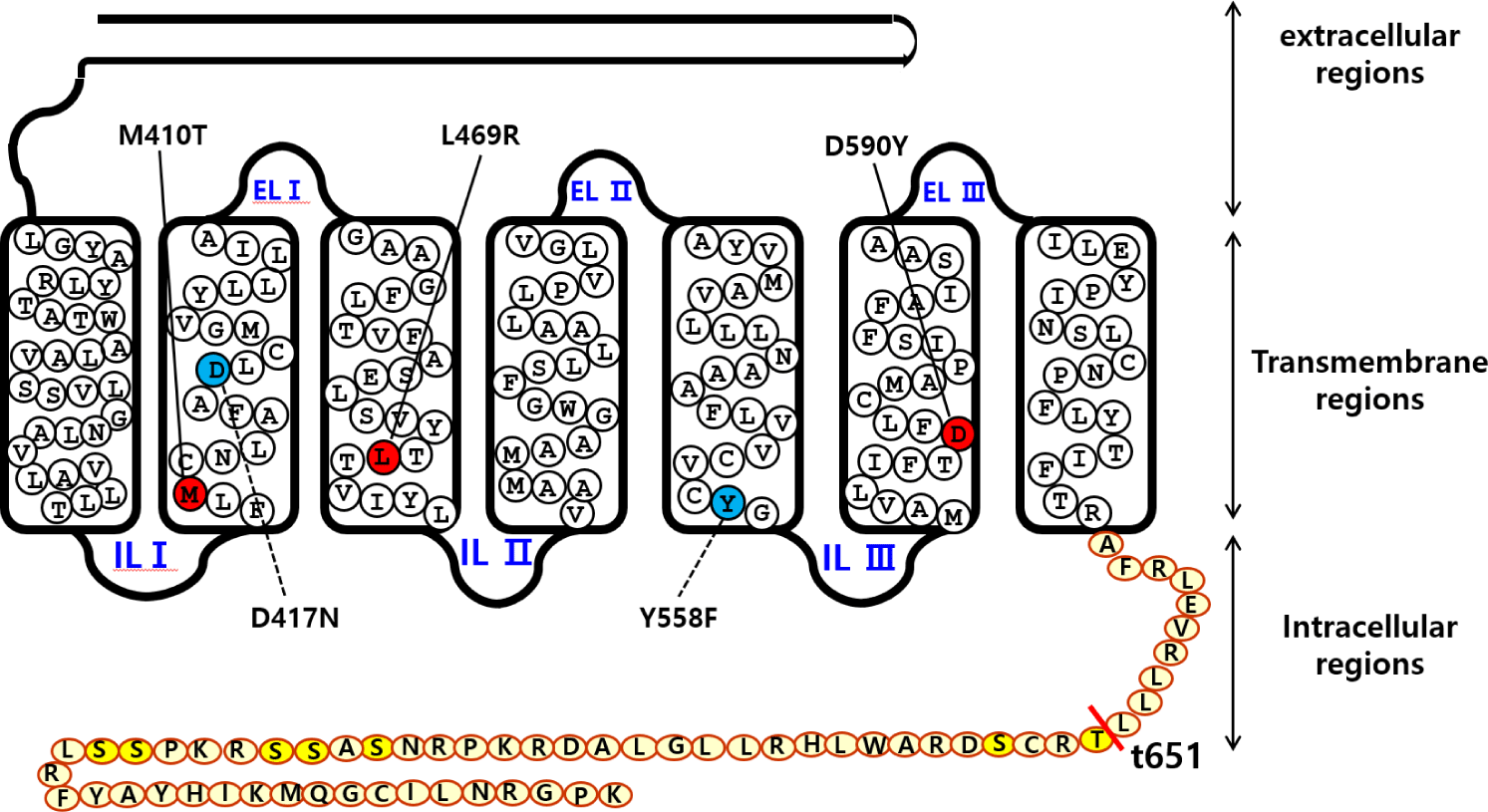
Plasmids cloned into the pCORON SP VSVG expression vector were transfected into CHO-K1 cells using liposome-mediated transfection, as previously reported (Byambaragchaa et al., 2024). The cells were grown to 80%–90% confluence in 6-well plates. Plasmid DNA and transfection reagent were mixed with Opti-MEM and incubated for 20 min at room temperature. The cells were washed with Opti-MEM and the DNA-Lipofectamine complex was added. Fresh growth medium containing 20% FBS was added to each well 5 h after transfection. The cells were subjected to cAMP analysis at 48 h post-transfection.
The pcDNA3 mammalian vectors were transfected into CHO suspension (CHO-S) cells using the FreeStyle MAX reagent, as reported in a previous study (Lee et al., 2021). Briefly, cells were cultured in 30 mL of CHO expression medium for 3 days. The cells were passaged at a 5–6×105 cells/mL in 125 mL CHO expression medium in a disposable spinner flask. The cell density was approximately 1.2–1.5 cells/mL on the day of transfection. Plasmid DNA (160 μg) and FreeStyle MAX reagent (160 μL) were mixed gently with 1.2 mL of OptiPRO serum-free medium and media samples were mixed and incubated for 5 min at RT. The mixed samples (2.4 mL) were gently added to the cell suspension flask. Finally, for the rec-eCG protein assay, the supernatant was collected on day nine post-transfection. The collected media were concentrated by CentriPlus centrifugal filters and quantified by PMSG ELISA with an anti-PMSG monoclonal antibody, horseradish peroxidase (HRP)-conjugated antibody, and TMB substrate, following the manufacturer’s instructions in the previous study (Lee et al., 2021).
cAMP accumulation in CHO-K1 cells expressing eel LH/CGR-wt and mutants was measured using a cAMP Dynamics 2 competitive immunoassay kit (Cisbio Bioassays, Codolet, France), as described in a previous study (Byambaragchaa et al., 2024). Briefly, transfected cells were diluted in 0.5 mM IBMX to inhibit cAMP degradation and seeded in 384-well plates (10,000 cells per well). Rec-eCG ligand (5 μL) was added to each well, and the cells were stimulated at RT for 30 min. The cAMP response was analyzed by measuring the decrease in HTRF energy transfer (665 nm/620 nm) using a TriStar2 S LB942 mi microplate reader (BERTHOLD Tech, Wildbad, Germany). The results were calculated from the ratio of fluorescence at 665 and 620 nm and expressed as Delta F% (cAMP inhibition) according to the following equation: Delta F% = (standard or sample ratio − mock transfection) 100 / mock transfection.
The cAMP concentration for Delta F% values was calculated using GraphPad Prism software (version 6.0; GraphPad Software, La Jolla, CA, USA).
The Multalin multiple sequence alignment tool was used for the sequence analysis. Dose-response curves were generated for experiments performed in duplicates. GraphPad Prism 6.0 (San Diego, CA, USA) was used to analyze the cAMP response, EC50 values, and stimulation curves. Curves fitted in a single experiment were normalized to background signals measured in mock-transfected cells. Each curve was plotted using the data from three independent experiments. Data were analyzed by one-way analysis of variance (ANOVA), followed by Tukey’s comparison test using GraphPad Prism v.6.0, as indicated in the figure captions. Statistical significance was determined at the following levels: * p<0.05 and ** p<0.01, indicating a significant difference between groups.
RESULTS
The in vitro biological activity was assessed using transfected CHO-K1 cells expressing eel LH/CGR-wt and activating mutants. The results of cAMP activation are shown in Fig. 2 and Table 1. The basal and Rmax cAMP responses in eLH/CGR-wt were 3.8 and 64.5 nM/104 cells, respectively (Table 1). cAMP responsiveness increased in a dose-dependent manner. The half-maximal effective concentration (EC50) value of rec-eCG-stimulated cAMP was approximately 58.66 ng/mL. The dose-response curve of the eLH/CGR-activating mutants was slightly shifted to the left compared with that of eLH/CGR-wt. Specifically, the basal cAMP response was very high compared to that observed in eLH/CGR-wt. The activating mutants (L469R and D590Y) showed 8.7- and 4.0-fold increases in the basal cAMP response, respectively, without rec-eCG agonist treatment. However, the M410T mutant did not show any difference in the basal cAMP response (Fig. 3).
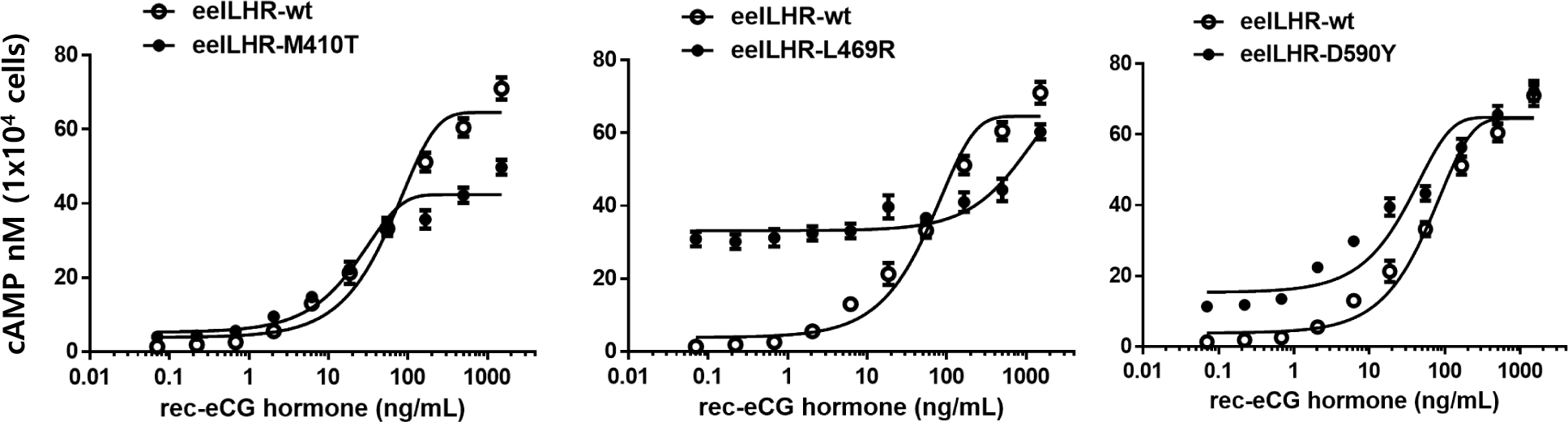
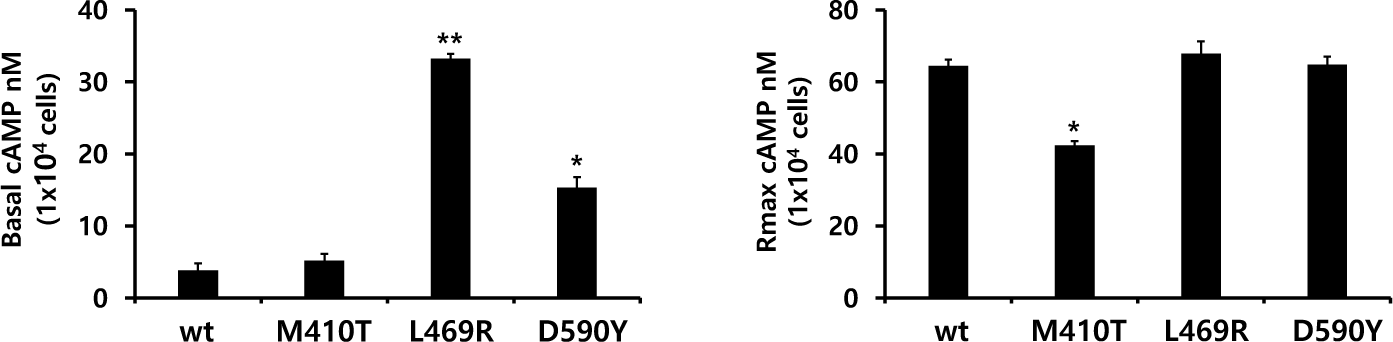
The L469R mutant showed the highest basal cAMP response, corresponding to approximately 50% of the maximal value observed in eLH/CGR-wt (Table 1). However, cAMP activity in the L468R mutant did not constitutively increase despite the high concentration of the rec-eCG agonist (Fig. 2). In the M410T mutant, the Rmax level was the lowest among all activating mutants. EC50 values in the M410T and D590Y mutants showed approximately 2-fold increase, but that in the L469R mutant could not be calculated exactly because of the high basal cAMP level priot to treatmemnt with rec-eCG agonist (100 ng/mL) (Table 1). Rmax values for L469R and D590Y mutants were almost similar to that of eel LH/CGR-wt, but the value for M410T mutant was significantly lower (42.4 nM/104 cells), which is approximately 65% of the Rmax value of the eel LH/CGR-wt (Table 1).
Eel LH/CGRs with inactivating mutations-D417N and Y558F were evaluated by quantifying cAMP accumulation in cells incubated with increasing concentrations of rec-eCG. The basal cAMP activity was not affected, as shown in Figs. 4 and 5 and Table 2. However, cAMP signaling was not completely impaired in these mutants, despite lower activation (Fig. 4). These Rmax values were approximately 50% of the corresponding values for eel LH/CGR-wt (Table 2). The EC50 value was approximately 108 ng/mL. However, the EC50 values of D417N and Y558F decreased 2.3- and 3.2-fold, respectively, demonstrating that detected approximately 253.9 ng/mL and 345 ng/mL, respectively.
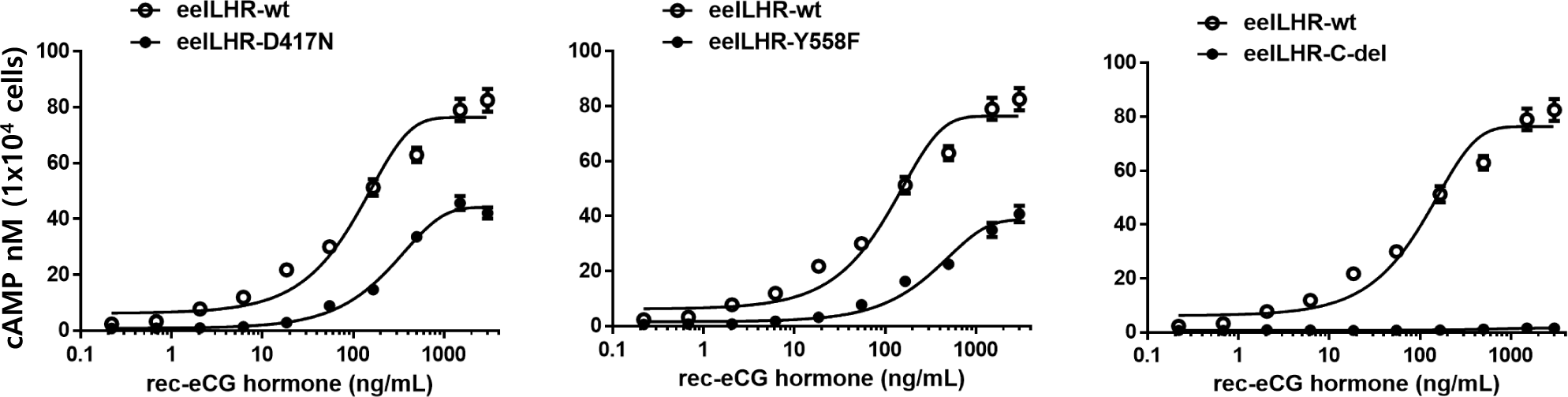
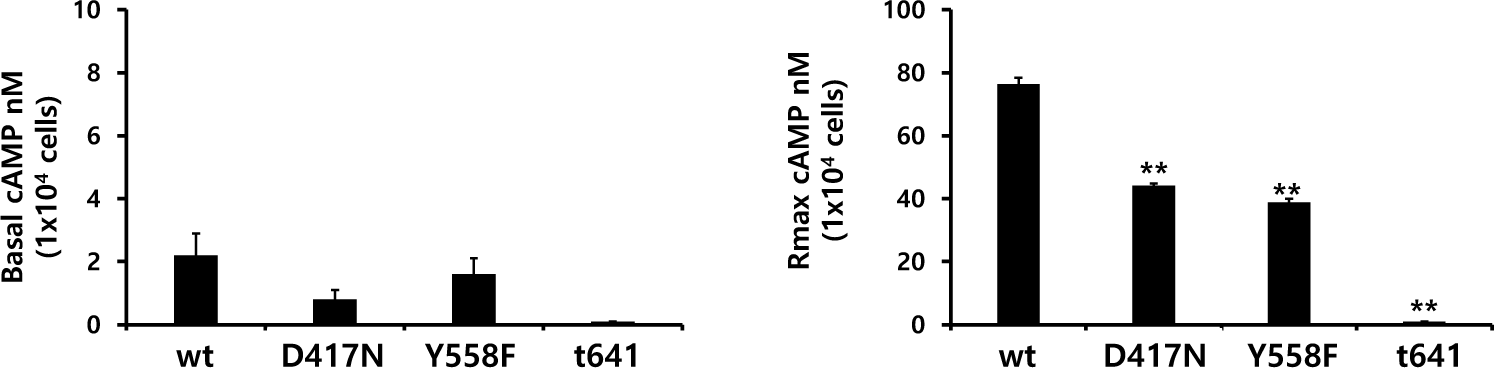
The eel LH/CGR also has a long C-terminal region containing approximately seven phosphorylation sites, as shown in Fig. 1. To assess the function of potential phosphorylation sites in cAMP signal transduction, eel LH/CGR-wt was truncated at amino acid 651 of the C-terminal region and the truncated mutant was referred to as eLH/CGR-t651. This mutation resulted in completely impaired cAMP responsiveness, as shown in Figs. 4 and 5 and Table 2. Thus, the Rmax value did not show any specific variation, indicating that the phosphorylation regions in the eel LH/CGR C-terminal region played a pivotal role in the PKA signal transduction pathway.
DISCUSSION
Based on the results of the present study, our findings clearly show that activating mutants exhibited constitutively active cAMP signaling (M410T, L460R, and D590Y), while inactivating (D417N and Y558F) mutants exhibited highly decreased and C-terminus deleted mutant (eel LH/CGR-t651) exhibited completely impaired cAMP responsiveness. However, all receptor mutants showed effective response to the rec-eCG agonist treatment. These highly conserved sites in the transmembrane region are important for downstream signal transduction. Therefore, we concluded that activating eel LH/CGR mutants exhibit continuous signaling without agonist stimulation. Phosphorylation sites in the C-terminal region of eel LH/CGR are essential for rec-eCG-induced phosphorylation.
Recently, we characterized eel LH/CGRs by rec-eel LH stimulation at the same sites, and found that activating mutants displayed 4–19-fold increase in basal cAMP responsiveness without agonist treatment (Byambaragchaa et al., 2021b). In equine LH/CGR, three constitutively activating mutants (L457R, D564G, and D578Y) exhibited 16.9-, 16.4-, and 11.2-fold increases in the basal cAMP response, respectively (Byambaragchaa et al., 2021a). Cells expressing eel LH/CGR-D576G mutant, which contains a highly conserved aspartic acid, exhibited a 5.8-fold increase following rec-eel LH and rec-eCG stimulation (Choi et al., 2023). Basal cAMP activity exhibited a 4.5-fold increase in COS-7 cells in hLH/CGR-D578G (Shenker et al., 1993) and hLH/CGR-L457R (Zhang et al., 2005, 2007; Galet & Ascoli, 2006; Latronico & Segaloff, 2007). In the present study, our data on the activation of eel LH/CGR-L469R and D590Y mutants were concordant with previous reports showing that the basal cAMP response resulted in a high increase without agonist stimulation.
In the inactivating mutants, many studies have reported in equine LH/CGR-D405N, -R464H, and Y546F (Byambaragchaa et al., 2021a), hLH/CGR-V144F (Richter-Unruh et al., 2005), hLH/CGR-F194V (Gromoll et al., 2002), rat LH/CGR-D383N, -R442H, and -Y524F (Dhanwada et al., 1996; Min et al., 1998), indicating that any mutants were completely impaired or not impaired. However, inactivating mutants did not affect the basal cAMP response.
Interestingly, our results in the presented study are no activity, in contrast to previously reported data for equine LH/CGR-t656 (Byambaragchaa et al., 2022b), equine FSHR-t641 (Seong et al., 2020), and eel FSHR-t614 (Kim et al., 2018), showing that agonist-induced receptors are not completely impaired in the cAMP response. In the present study, eel LH/CGR-t651 mutant were completely impaired. Thus, we suggest that the C-terminal regions of eel LH/CGR are necessary for agonist-induced uncoupling, with only seven phosphorylation sites. Thus, phosphorylation-dependent structure of the GPCR C-terminal region has been reported to modulate arrestin binding, and therefore, signaling is modulated in arrestin-dependent pathways (Guillien et al., 2023). Although this mechanism is not well understood, phosphorylation-and β-arrestin-dependent pathways may exist in truncated mutants for the internalization of eel LH/CGR. Thus, we suggest that conformational changes in the serine and threonine residues of the C-terminal domain of GPCR play an important role in the downstream signaling pathway.
In conclusion, our study showed that the activating mutants greatly increased the basal cAMP response, whereas the inactivating mutants decreased the Rmax cAMP response and EC50 value.
C-terminal deletion of potential phosphorylation sites completely impaired the cAMP response. The results of the present study suggest that there is a strong correlation between highly conserved activating and inactivating sites. Although not revealed in this study, the highly conserved regions in the C-terminus of eel LH/CGR represent specific signaling pathways such as internalization. Taken together, PKA signaling via LH/CGR-mediated signaling must be further studied to determine whether any pathway involving the phosphorylation of downstream effectors of the MAPK pathway exists.