INTRODUCTION
The mammalian testis is a highly heterogenous organ composed of functionally and developmentally multiple elements, including germ cells and somatic cells (Svingen & Koopman, 2013; Tremblay, 2015). This strongly suggests that more diverse genes are likely to be expressed in the testis compared to other relatively simple composition and consistent functioning organs in the body. In fact, numerous factors are expressed locally in the testis, including those are originally actively expressed in the hypothalamus or pituitary gland (Li et al., 2024). For examples, gonadotropin-releasing hormone (GnRH) and its receptor (Choi et al., 1994, Bull et al., 2000; Anjum et al., 2012), kisspeptin (product of KiSS1 gene, Salehi et al., 2015; Sharma et al., 2020) pituitary adenylate cyclase-activating polypeptide (PACAP, Arimura, 1998; Li et al., 2004), and LH subunit genes (Zhang et al., 1995a,b; Kim & Lee, 2017) are proven to be expressed locally in rats and mice testis. However, to date, little has been known on the precise role(s) and regulation of these local factors. To understand the function and regulation of local regulatory factors in detail, it would be useful to accumulate basic information about them, such as spatiotemporal expression and regulation patterns, changes in expression patterns in physiological or pathological situations, etc. In the present study, we investigate the temporal changes in expression pattern of reproductive hormone, that constitute the hypothalamic-pituitary hormone axis, in the testes between postnatal day 23 (PND 23) and PND83 to understand the possible function and regulation of the local regulators in the testis.
MATERIALS AND METHODS
Pregnant Sprague-Dawley rats were provided by DBL (Eumseong, Korea) and reared in Sangmyung University animal facility under photoperiods of 12 h light/dark with lights on at 07:00 and constant temperature of 21 °C -23 °C. Food and tap water were supplied ad libitum. The animal protocols were approved by the Animal Care and Use Committees at Sangmyung University (2018-03; approval number R-1801-1). All the animals received humane care in accordance with the guides for animal experiments of the Association for Assessment and Accreditation of Laboratory Animal Care (AAALAC) International.
Animals were sacrificed on PND23, PND53 and PND81; PND23 group represents immature stage, PND53 group represents pubertal stage and PND81 group represents young adult stage. Our preliminary trials revealed that the presence of sperm in dissected epididymal suspension confirmed at PND53. The testicles were cut out following excision of the skin of scrotal sac. Sacrifice and sample collection were done between 18:00-19:00. The tissues were immediately soaked in the RNA isolation solution, stored at -70°C until RT-PCRs.
Total RNAs were isolated from samples using the acid guanidinium thiocyanate-phenol-chloroform (AGPC) procedure. To five volumes of the RNA extraction solution containing the sample were added 1 volume of 3 M sodium acetate (pH 5.2, Bioneer, Daejeon, Korea) and 6 volumes of PCI (pH 4.3, 5:1 Phenol:Chloroform, Bioneer) in a 1.5 mL tube (Hankuk Bioscience, Seongnam, Korea). Mixed samples by inverting were centrifuged at 14,000×g for 25 min in cooling centrifuge (Hanil, Gimpo, Korea) at 4°C. The supernatant was carefully transferred to a new tube and the same volume of isopropanol (Duksan, Ansan, Korea) was added. The samples were mixed and placed over an hour at -20°C. The samples were centrifuged prior to the supernatant removed. The precipitated pellet was added to the RNA extraction solution and this process was repeated once more. Then the RNA pellet was washed with 1 mL of 70% ethanol (Merck, Darmstadt, Germany) twice by centrifugation. The final pellet was resuspended in diethylpyrocarbonate (DEPC, Sigma-Aldrich, St. Louis, MO, USA)-treated distilled water. The samples were stored at -70°C before using.
Total RNAs were used in RT-PCR reactions carried out with Maxime™ RT PreMix (InTron, Seongnam, Korea) and AccuPower PCR Premix (GeneAll, Seoul, Korea) according to the manufacturer’s instructions. Sequences of the primers and the specific PCR conditions used in this study were successfully worked in the previous study (Yoo & Lee, 2016), and were listed in Tables 1 and 2, respectively. The reactions were subjected to MultiGene™ OptiMax Thermal Cycler (Labnet, Edison, NJ, USA). The reaction products were analyzed by gel electrophoresis in 1.5% agarose gel (75 V, 65 min) and visualized by ethidium bromide staining (Dye All, GeneAll). The band intensities were measured using the image analysis system (Imager III-1D main software, Bioneer). Glyceraldehydes-3-phosphate dehydrogenase (GAPDH) was used as reference gene for normalization of quantitative RT-PCRs in the present study.
tLHβ, type luteinizing hormone β subunit; pLHβ, type luteinizing hormone beta subunit; Cgα, glycoprotein hormone common alpha subunit; LHCGR; luteinizing hormone/choriogonadotropin receptor; PACAP, pituitary adenylate cyclase-activating polypeptide; GnRH, gonadotropin-releasing hormone; GAPDH, glyceraldehydes-3-phosphate dehydrogenase.
tLHβ, type luteinizing hormone β subunit; pLHβ, type luteinizing hormone beta subunit; Cgα, glycoprotein hormone common alpha subunit; LHCGR; luteinizing hormone/choriogonadotropin receptor; PACAP, pituitary adenylate cyclase-activating polypeptide; GnRH, gonadotropin-releasing hormone; GAPDH, glyceraldehydes-3-phosphate dehydrogenase.
All experiments were performed at least three times. Values were expressed as mean±SE data were analyzed using student’s t-test as indicated. p<0.05 was considered statistically significant. Calculations were performed using Graphpad Software Prism version 5 (San Diego, CA, USA).
RESULTS AND DISCUSSION
In our RT-PCR study, GnRH mRNA level at PND 23 (1.00±0.06 AU) was significantly higher than those of PND 53 (0.47±0.03 AU) and PND81 (0.51±0.05 AU) (p<0.001, Fig. 1A). KiSS1 mRNA level at PND 23 (1.00±0.09 AU) was significantly higher than those of PND 53 (0.62±0.07 AU, p<0.05) and PND81 (0.34±0.06 AU, p<0.001), and KiSS1 mRNA level at PND 53 was significantly higher than that of PND 81 (p<0.05, Fig. 1B). PACAP mRNA level at PND 23 (1.00±0.25 AU) was significantly lower than those of PND 53 (5.11±0.21 AU) and PND81 (4.53±0.34 AU) (p<0.001, Fig 1C).
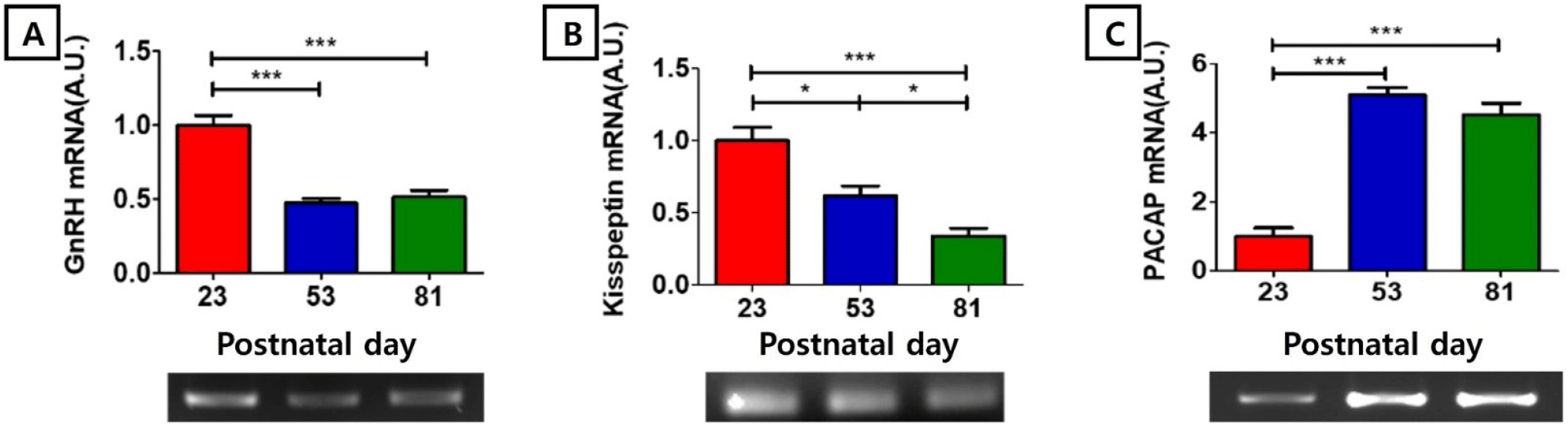
The mRNA level of testis type luteinizing hormone beta-subunit (tLHβ) at PND 23 (1.00±0.52 AU) was significantly lower than those of PND 53 (15.00±0.26 AU, p<0.001) and PND81 (12.37±0.54 AU, p<0.001), and the level at PND 53 was significantly higher than that of PND 81 (p<0.01) (Fig. 2A). The mRNA level of pituitary type luteinizing hormone beta subunit (pLHβ) showed nadir at PND 23 (1.00±0.45 AU), significantly lower than those of PND53 (127.38±4.38, p<0.001) and PND81 (125.91 AU, p<0.001) (Fig. 1B). The mRNA level of glycoprotein hormone common alpha subunit (Cgα) at PND23 (1.00±0.19 AU) was significantly lower than those of PND53 (4.11±0.31, p<0.001) and PND81 (3.05±0.32 AU, p<0.001), and the level of PND 53 was significantly higher than that of PND 81 (p<0.05) (Fig. 2C). The mRNA level of LH receptor (LHCGR) at PND 23 (1.00±0.04AU) was significantly lower than those of PND53 (1.47±0.07, p<0.001) and PND81 (1.23±0.08AU, p<0.05) (Fig. 2D).
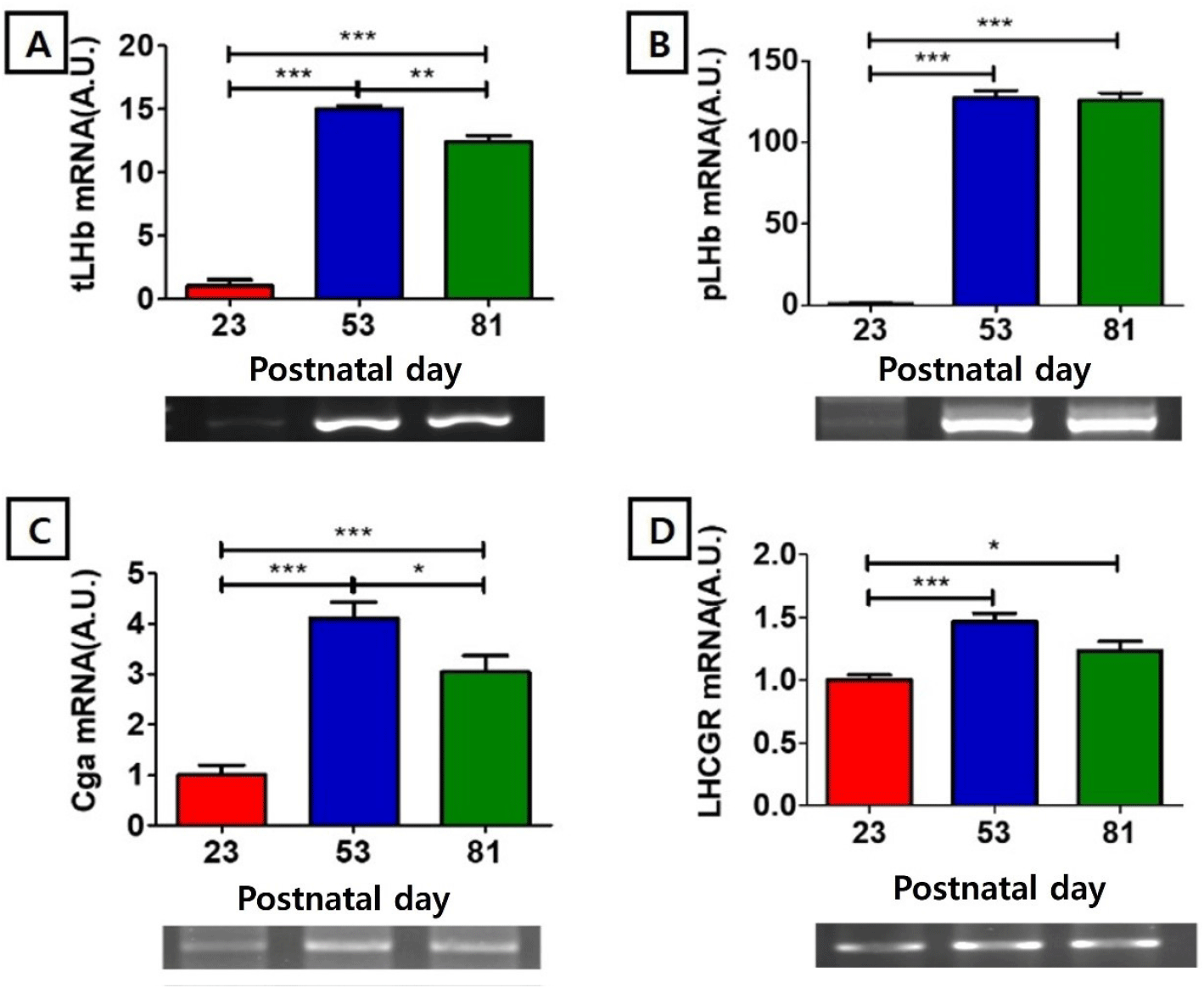
Among the hormonal factors whose expression and function were first reported in the hypothalamus of animals, especially in rodents, GnRH is the most well-known substance whose local expression in the testes was reported (Sharpe et al., 1982; Choi et al., 1994). GnRH mRNA was expressed within the seminiferous tubules in both mature rat and adult human testis. In rats, expression of GnRH mRNA was identified in the Sertoli cells and spermatogenic cells, and the receptors for GnRH were localized to cells in the interstitial tissues of the testis, probably Leydig cells (Bahk et al., 1995). In mice, immunoreactivity of GnRH increased in the interstitial cells during development; strong GnRH immunostaining was observed in the Leydig cell in the birth, pubertal, and reproductive active stages, whereas moderate immunostaining in germ cell of disorganized seminiferous tubules during senescence (Anjum et al., 2012). GnRH receptor mRNA is expressed in rat and mouse testicular germ cells, and these multiple GnRH-receptor encoding transcripts is also of interest and warrants further studies to evaluate their regulation and function (Bull et al., 2000).
In 2010s, the kisspeptin system has been included among the main central modulators of reproduction in rodent species due to its ability to regulate upstream activity of GnRH secreting neurons (Spaziani et al., 2021). Apart from the brain, kisspeptin system is widely distributed in peripheral tissues. Indeed, it is highly expressed in the testis (Meccariello et al., 2020; Sharma et al., 2020), raising the possibility that peripheral kisspeptin activity may sustain the formation and the physiology of spermatozoa (Mele et al., 2022). Interestingly, immunoreactive kisspeptins were observed mainly in Leydig cells, and the report that kisspeptin in the serum level decreased when gonads were removed suggests that Leydig cells could produce and secrete kisspeptin into the blood, enabling various functions in body (Salehi et al., 2015). Previously we reported that modest immunoreactive kisspeptins were detected in Leydig-interstitial cells, and the weak signals were detected in germ cells, mostly in round spermatids and residual bodies of elongated spermatids of the adult hamster (Park et al., 2022).
PACAP is an ancestral molecule that was isolated from sheep hypothalamic extracts based on its action to stimulate cAMP production by pituitary cell cultures, and this neuropeptide is one of the ligands that coordinate with GnRH to control reproduction (Winters & Moore, 2020; Li et al., 2024). PACAP is expressed in rat testis and is mediated intracrine signaling in the testicular germ cells (Arimura, 1998; Li et al., 2004). The testis-specific PACAP promoter sequence directs transcription in postmeiotic round spermatids during developmental stages I-VIII (Daniel & Habener, 2000). Mice PACAP and its receptor is expressed not only in testicular germ cells but also in Leydig cells, confirming its involvement in the control of steroidogenesis in mouse (Prisco et al., 2020).
It is currently unknown whether kisspeptin acts as an upstream regulator of GnRH in the testis as in the hypothalamus. Intriguingly, present study revealed the intratesticular expression of kisspeptin and GnRH from immaturity (PND23) to puberty (PND53) and emerging adults (PND83) showed a very similar trend, with expression peaking during immaturity and decreasing thereafter. On the contrary, the expression of PACAP in the testis showed a pattern of lowest expression during immaturity and then rising thereafter. Our finding suggest that the sequential mode of Kisspeptin-GnRH regulation might be present in rat testis with non-neuronal cells. Concerning the temporal changes in testicular PACAP expression, this type tended to be much more similar to that in expressions of testicular gonadotropin subunits, well-matched with the sequential mode of hypothalamic PACAP-pituitary gonadotropin regulation (Li et al., 2024).
In 1995, novel expression and its tissue-specific structure of luteinizing hormone subunit genes in the rat testis were published (Zhang et al., 1995a,b). The testicular LHβ mRNA has a 1.9kb 5’-extension, compared to the cognate pituitary mRNA. Their Northern hybridization study revealed the 2.7-kb mRNA transcript was found in the rat testis, but none in the pituitary (Zhang et al., 1995a). In situ hybridization revealed that LHβ expression was confined in seminiferous tubules to step 2–10 spermatids, and the LH-like protein was present in the cytoplasm of elongated spermatids as analyzed by immunohistochemistry. The authors suggested that the spermatogenic cells seem to produce LH-like substance during spermiogenesis, and the role of the peptides that originate from unique testicular transcripts of the cognate genes is remains unknown (Zhang et al., 1995b). We also found that the mouse testicular type LH-β products were produced by a primer set based on the rat sequences, with unexpected size of 800 bp (Kim & Lee, 2017). Sequencing revealed that the proximal and distal parts (2–82 and 661–773 bp, respectively) were homologous to rat testicular LHβ cDNA, and middle part (83–660 bp) was a unique mouse-specific region. Both Cgα and LHβ positive immunostaining signals were in the round and elongated spermatids and mature sperms. In the present study, we found that the expression of tLHβ and pLHβ was very low during immature stage but increased significantly during puberty and early adulthood. Expression of the cgα was lowest at the immature stage, but the relative expression was higher than that of the β subunits and increased at later stages. The expression pattern of LHCGR was similar to the pattern of cgα expression.
Mammalian reproductive function is a complex system of many players orchestrated by the hypothalamus-pituitary-gonadal (HPG) axis (Tsukamura, 2022). The HPG axis, also known as the hypothalamic–pituitary–ovarian/testicular (HPO/HPT) axis, mainly controls development, reproduction, and aging in mammals. This hormonal axis concept has been widely used to understand and explain the mammalian reproductive physiology conveniently and descriptively. Meanwhile, numerous factors other than GnRH-gonadotropins-sex steroids have been discovered in the organs of the axis (Tremblay, 2015; Spaziani et al., 2021). These are mostly present in traceable amount, so it was difficult to confirm using the existing Northern blot and immunoassay. With the introduction of PCR technology in late 1980s, research on the presence of local regulators was conducted like a trend (Huhtaniemi & Toppari, 1995; Huleihel & Lunenfeld, 2004). However, studies on the role of these local factors are still not easy to solve due to several limitations. As in the case of rat GHRH (Srivastava et al., 1995), if a promoter different from the originally discovered in hypothalamus is used in the testis, there might be a huge difference between the cognate hypothalamic expression and the local expression such as size and number of exon(s) and the resulting translated products. These differences in the transcript and the translated product between the organs, making it difficult to study the basic characteristics of biologically active tissuespecific protein or peptide products. Studies on the possibility of tissue-specific effect on the locally expressed receptor are also a prerequisite. Research on their local function will be hard to explain when the factor is considerably different in size with authentic hormone. Additionally, a strategy to develop testis-specific expression-silencing animals using gene editing or equivalent technology would be helpful.
Our attempts will provide clues to understanding the functions of local regulatory factors in the testis and further understanding various physiological and pathological phenomena of the testis.