INTRODUCTION
Gonadotropin-releasing hormone (GnRH) is a critical hormone in the regulation of reproductive processes in the body. Produced in the hypothalamus as a neurohormone, GnRH primarily regulates the release of LH and FSH from the pituitary gland (Schally et al., 1971). These hormones are essential for the functioning of the ovaries in women and the testes in men. In women, LH and FSH stimulate the ovaries to produce estrogen and progesterone, which are essential for the menstrual cycle, ovulation, and pregnancy. In men, LH stimulates testosterone production in the testes, while FSH is critical for sperm production and maturation (Nieschlag et al., 1999; Smith, 2009). The release of GnRH from the hypothalamus is influenced by several factors, including stress, exercise, and levels of circulating sex hormones, and plays an important role in the feedback loop that maintains the hormonal balance necessary for reproductive health (Elias & Wilson, 1993; Dong et al., 1998; Ciechanowska et al., 2010).
Although GnRH is primarily known for its role in the hypothalamic-pituitary axis, recent studies suggest that it has local effects in the ovaries and uterus (Michael Conn & Crowley, 1994; Raga et al., 1998; Choi et al., 2006). Research has demonstrated the presence of GnRH and its receptors (GnRHRs) in ovarian and uterine tissues (Knecht et al., 1985; Minaretzis et al., 1995; Schäfer-Somi et al., 2015). This suggests a potential autocrine or paracrine role for GnRH within these organs, where it may act directly on the cells that produce it or on neighboring cells, as opposed to its systemic role, where GnRH acts as a hormone released into the bloodstream (Dong et al., 1998; Choi et al., 2006; Schäfer-Somi et al., 2015).
In the ovaries, GnRH is known to regulate several functions, including follicular maturation, ovulation, and the production of ovarian steroids such as estrogen and progesterone (Hillensjö & LeMaire, 1980; Parborell et al., 2005; Singh & Krishna, 2010). In the uterus, GnRH has been reported to act as a local regulator involved in the regulation of various uterine functions, including cell proliferation and apoptosis in the uterine endometrial cells, which are critical for menstrual health and successful implantation during pregnancy (Imai et al., 1998; Meresman et al., 2003; Guo et al., 2018). The function of GnRH in the ovary involves interactions with other local and systemic hormones, specifically it is a modulator of the actions of LH and FSH (Gong et al., 1995; Sonntag et al., 2005; Nyuji et al., 2019). In addition, it has been reported that GnRH in the uterus is intertwined with other hormonal pathways, such as those involving estrogen and progesterone (Metallinou et al., 2007).
In vitro fertilization-embryo transfer (IVF-ET) is a series of procedures in which fertilization, which typically occurs in the woman’s body, is artificially induced outside the human body. Subsequently, an embryo is transferred to the woman to facilitate pregnancy. In the IVF-ET process, the first essential step is the induction of superovulation to retrieve mature oocytes from the woman. Artificial superovulation is induced by gonadotropin hormone injections, a process known as controlled ovarian hyperstimulation (COH). Common superovulation agents include FSH, human menopausal gonadotropin (hMG), and human chorionic gonadotropin (hCG) (Li et al., 2015). In order to increase the chances of successful pregnancy, infertile couples often repeat the COH process several times. However, repeated administration of high doses of exogenous gonadotropins has been reported to cause ovarian dysfunction. Such repeated ovarian stimulation can lead to ovarian hyperstimulation syndrome, a condition that occurs when the ovaries are over-stimulated by gonadotropin hormones during IVF-ET (Jacobs & Agrawal, 1998). In contrast, other studies have shown that COH procedure does not adversely affect oocyte retrieval, embryo implantation rates, or pregnancy success rates (Caligara et al., 2001; Acevedo et al., 2006).
However, it remains unclear whether the administration of high doses of exogenous gonadotropins affects ovarian and uterine function. Therefore, we investigated the expression of GnRHR, recognized as local regulators in the ovary and uterus, in both the ovary and uterus of mice following injection of high doses of pregnant mare serum gonadotropin (PMSG), which has follicle stimulating (FSH-like) and luteinizing (LH-like) activities. In addition, we examined the expression of GnRH receptor in the ovary and uterus of young and aged mice to investigate the possibility that GnRH produced in these organs may be involved in age-related reproductive decline in female mice.
MATERIALS AND METHODS
Six-week-old and 12-month-old female ICR mice were purchased from Raonbio (Yongin, Korea) and housed in groups of five per cage under controlled illumination (12:12 h light/dark cycle, lights on/off: 6 h/18 h) and temperature (22±2°C). Animals were fed a standard rodent diet and tap water ad libitum. Animal care and experimental procedures were approved by the Institutional Animal Care and Use Committee at the Seoul Women’s University in accordance with guidelines established by the Korea Food and Drug Administration.
We used 6-week-old mice for the young group and 12-month-old mice for the aged group of three animals each (n=3). The mice were euthanized by CO2 anesthesia, and the cerebrum, pituitary gland, thymus, heart, lung, stomach, pancreas, kidney, spleen, large intestine, small intestine, muscle, ovary and uterus were harvested and immediately stored until use. We also used 6-week-old young mice divided into three groups of three animals each (n=3). They received intraperitoneal injections of either 10 or 30 IU of PMSG (Sigma-Aldrich, St. Louis, MO, USA) and saline as controls. Sixteen hours later, the mice were euthanized by CO2 anesthesia, and the ovary and uterus were harvested and immediately stored until use.
Total RNA was isolated by using the TRIzol (Invitrogen, Carlsbad, CA, USA). The final pellet was air dried and dissolved into RNase-free DEPC solution (Invitrogen). The RNA concentration was measured with the Nano-drop (ThermoFisher Scientific, Waltham, MA, USA). The extracted RNA was used for cDNA synthesis with oligo dT primers, dNTPs (Promega, Madison, WI, USA), and reverse transcriptase (Invitrogen) in RT buffer (Invitrogen). In the process of synthesizing cDNA, a Gene Pro thermal cycler (Bioer, Hangzhou, China) was used. First strand cDNA synthesis was performed in RNase-free DEPC solution containing 2 μg total RNA and 10 pmol oligo dT at 70°C for 5 min, followed by double-strand synthesis in 5X RT buffer and 0.1 M DTT (Invitrogen) with 10 mM dNTP (Promega), 200 unit/μL RTase at 37°C for 60 min and at 72°C for 15 min.
Conventional RT-PCR was performed in buffer solution containing template cDNA, Taq polymerase (Enzynomics, Daejeon, Korea) and each primer. The following pairs of primers were used: GnRHR forward 5’-ACCCACGCAGTACGGTATTC-3’; reverse 5’-GTGGCTACACCACTGTCGAA-3’ and β-actin forward 5’-AGCCATGTACGTAGCCATC-3’; reverse 5’-ATCTTCATGGTGCTAGGAGC-3’ primers (Bioneer, Daejeon, Korea). The optimum temperature cycling protocol was used as 95°C for 30 s, 60°C for 1 min and 72°C for 1 min, using the Gene Pro thermal cycler (Bioer). The PCR products were run on a 2% agarose gel. The bands were visualized using Dye Loading STAR(6X) (DYNE BIO, Seongnam, Korea) and detected using Biospectrum Imaging System (Analytik Jena, Jena, Germany).
qRT-PCR was performed in a buffer solution containing template cDNA, SYBR Green (Enzynomics), and each primer. The primer pairs were as follows: 18S forward 5’-GTCTGTGATGCCCTTAGATG-3’; reverse 5’-AGCTTATGACCCGCACTTAC-3’ and GnRH1 forward 5’-TCACATCCAAACAGATGGGCAA-3’; reverse 5’-CAGATCCCTGAGGGGTGAAC-3’ and GnRHR forward 5’-TCCGTGAGTTCACCAACCAA-3’; reverse 5’-TGAGTGGGGGTTCCCTGTTA-3’ (Bioneer). qRT-PCR was performed using the Light Cycler® 480 Real-time PCR System (Roche, Manheim, Germany).
The tissues were fixed in 4% paraformaldehyde for 30 min. The tissues were rinsed in ethanol series to remove fixative residues, embedded in paraffin block. The tissues blocks were cut 10 μm sections using a microtome, deparaffinized, and rehydrated with graded xylene-alcohol series, and then washed with PBS before immunostaining. The sections were blocked with peroxidase blocking reagent (Dako, Produktionsvej, Denmark) for 10 min and 2.5% horse serum for 20 min to prevent nonspecific binding. After rinsed with washing buffer, the sections were incubated with anti-rabbit GnRHR polyclonal antibody (1:200, Abcam, Cambridge, UK) at 4°C for overnight. The sections were washed with PBS, rinsed and incubated with Goat anti-rabbit IgG 594 conjugated (1:400, Bethyl Laboratoies, Montgomery, TX, USA) at 26°C for 1 h. All of the stained sections were counter-stained with DAPI and mounted with mounting medium (Vector Laboratories, Newark, CA, USA), and then observed under fluorescence microscopy (Axioskop2, Carl Zeiss, Oberkochen, Germany).
RESULTS
To compare GnRHR mRNA expression in various organs between young and aged mice, organs from 6-week-old and 12-month-old female mice were dissected. GnRHR mRNA in each organ was detected by conventional RT-PCR. The PCR product had a size of 310 bp, confirming that GnRHR is highly expressed in the pituitary gland, the primary target organ of GnRH. In addition to the pituitary gland, GnRHR mRNA expression was observed in all other organs examined, although expression levels were much lower than in the pituitary gland. In particular, high levels of GnRHR mRNA expression were observed in the heart and liver of young mice (Fig. 1A), while increased expression was observed in the thymus, lung, and small intestine of aged mice (Fig. 1B). In addition, GnRHR expression was higher in the kidney and spleen than in other organs of both young and aged mice (Fig. 2).
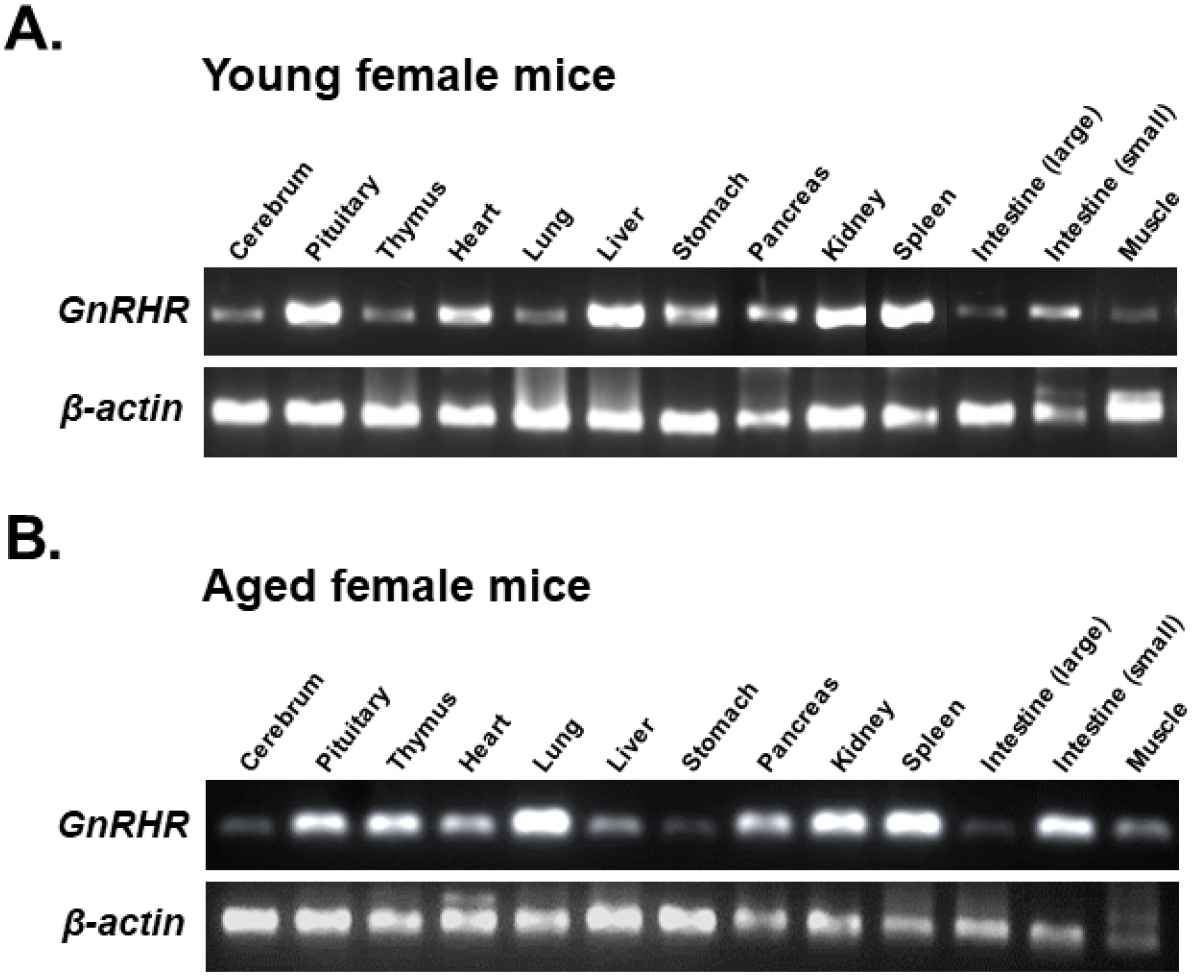
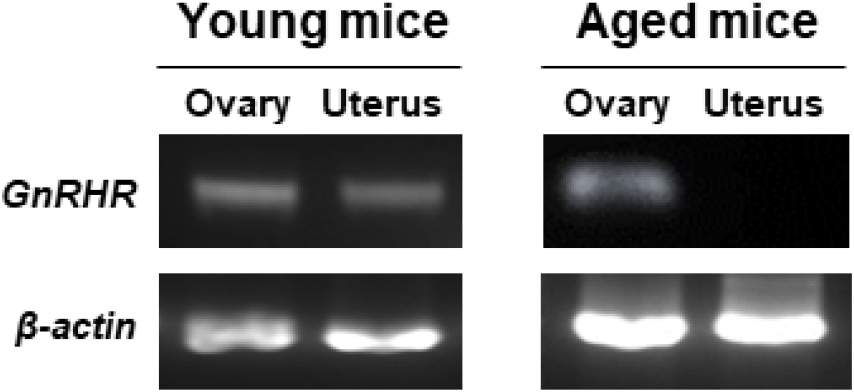
qRT-PCR was then used to compare the levels of GnRHR mRNA in organs that express GnRHR. As expected, the pituitary gland showed the highest expression of GnRHR, which was nearly 20-fold higher than the next most highly expressed organs, such as the liver, spleen, ovary, and uterus in young mice (Fig. 3A). In contrast, in aged mice, while GnRHR expression in the pituitary gland was lower compared to young mice, it was still nearly 5-fold higher than in organs like the thymus and kidney, which had the next highest expression levels (Fig. 3B). Notably, GnRHR expression was low in the thymus of young mice but high in aged mice. Conversely, its expression was high in the liver of young mice but low in aged mice (Fig. 3).
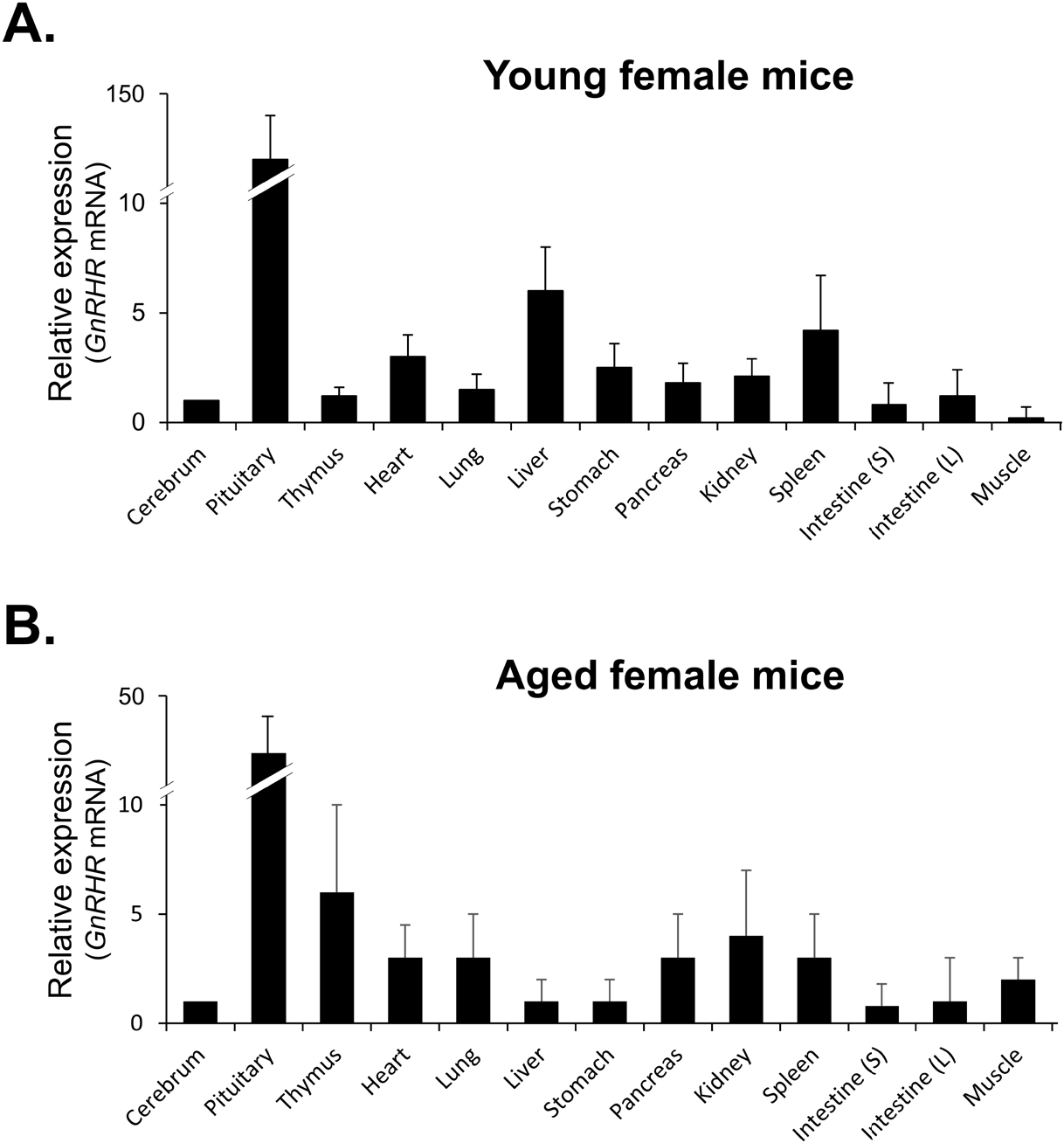
GnRHR mRNA was also detected in the ovaries of both young and aged mice by conventional RT-PCR. However, it was almost undetectable in the uterus of aged mice (Fig. 2), whereas its mRNA expression was observed in the uterus of young mice. To further compare the levels of GnRHR mRNA in the ovary and uterus of young and aged mice, we performed qRT-PCR analysis. GnRHR expression was lower in both the ovary and uterus of aged mice compared to young mice. Notably, in aged mice, GnRHR expression in the uterus was dramatically reduced compared to that in young mice (Fig. 4A). GnRH receptors in the ovary were localization by immunohistochemical staining. GnRH receptors were presented in the granulosa cells of an ovarian follicle (Fig. 4B).
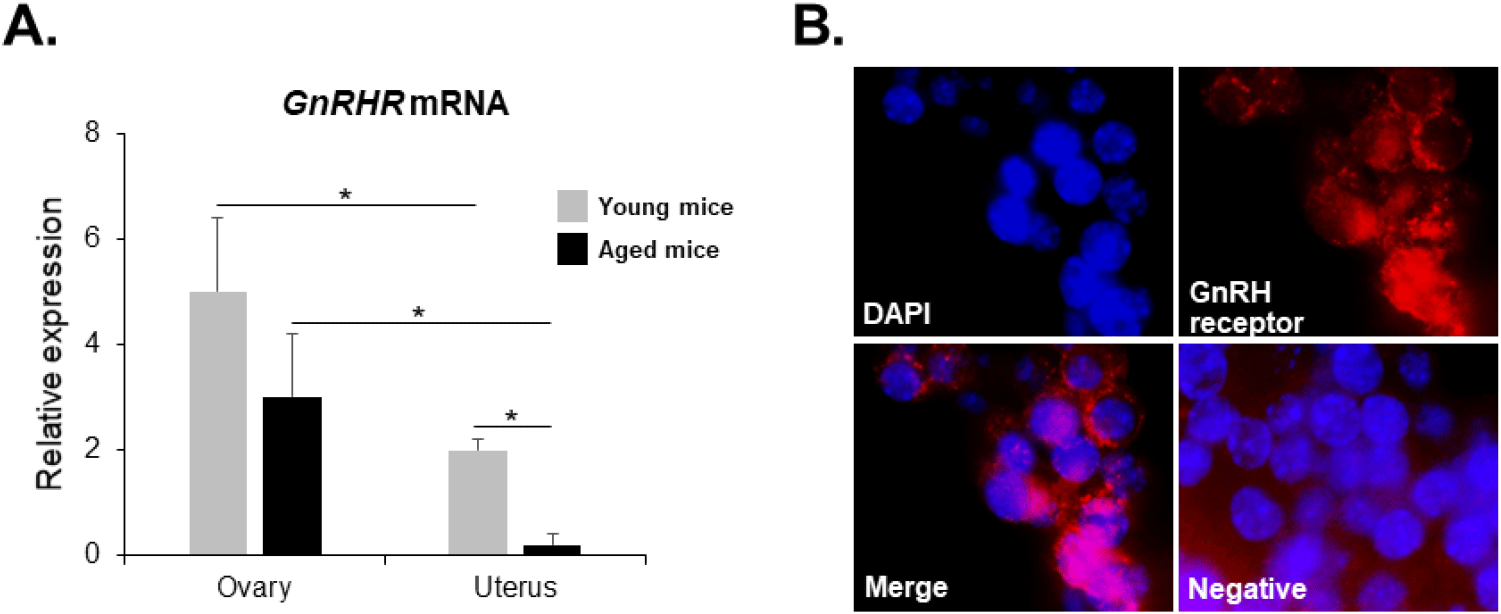
To investigate whether high-dose gonadotropins regulate the expression of GnRHR genes in the ovary and uterus, mice were injected with high doses of PMSG. GnRH and GnRH mRNA expressions were then analyzed by qRT-PCR. The results indicated a significant decrease in GnRH mRNA levels in the ovary, while a significant increase was observed in the uterus following administration of both 10 and 30 IU of PMSG (Fig. 5A and B). In addition, GnRH mRNA expression levels were examined in the ovary and uterus after PMSG administration. Interestingly, GnRH mRNA expression in both organs showed opposite trends to GnRHR expression. Specifically, GnRH mRNA levels were significantly increased in the ovary, whereas a significant decrease was observed in the uterus after administration of both 10 and 30 IU of PMSG (Fig. 6A and B).
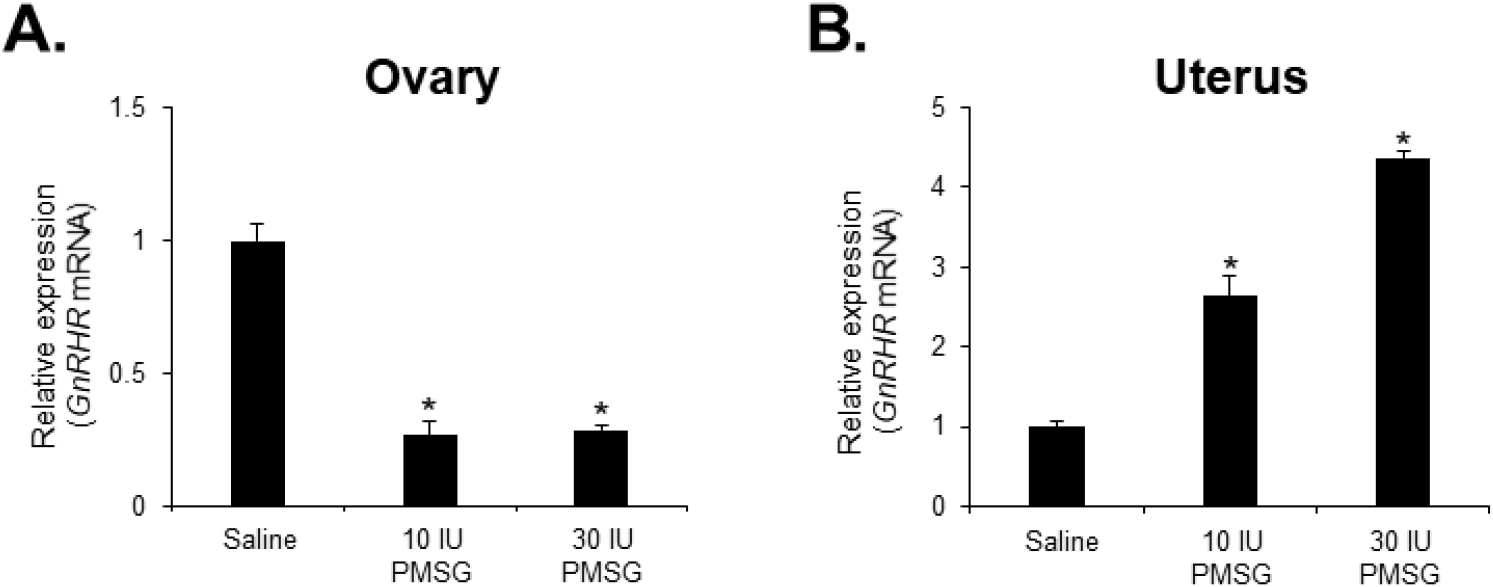
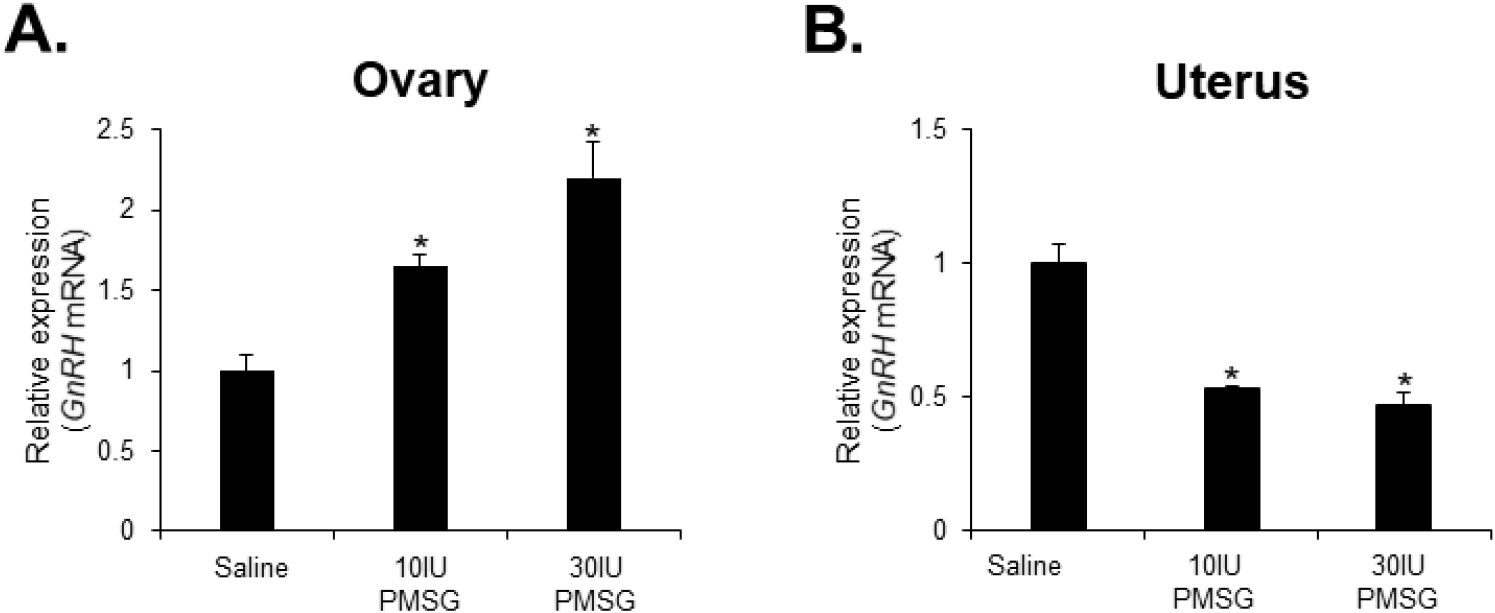
DISCUSSION
COH is a critical first step in the IVF process, aimed at obtaining a large number of high-quality oocytes. This requires that patients receive repeated high doses of gonadotropins during COH. However, such repeated high-dose ovarian stimulation could potentially interfere with ovarian follicle development and embryo implantation (Whang et al., 2021; Clark et al., 2022). Postulating that these problems may be locally induced by GnRH, which is expressed in both the ovary and uterus, we conducted a study to investigate whether high doses of gonadotropins modulate the expression of GnRHR in these organs. In addition, we examined the expression of GnRH receptor in the ovary and uterus of young and aged mice to elucidate whether GnRH expressed in these organs may be one of the factors contributing to the age-related decline in reproductive function in female mice.
We first confirmed the previously reported GnRHR mRNA expression in various organs, including the ovaries and uterus of young and aged mice (Pieper et al., 1981; Kakar & Jennes, 1995; Cheung & Wong, 2008). As expected, GnRHR mRNA expression was much higher in the pituitary glands of both young and aged mice. This is consistent with the understanding that the pituitary gland is the primary target organ for GnRH, a peptide hormone produced in the hypothalamus (Torrealday et al., 2013). The interaction of GnRH with its receptor in the pituitary gland is fundamental to the regulation of reproductive processes (Catt et al., 1985). In addition, our results showed that GnRHR mRNA was also highly expressed in the heart, liver, thymus, lung, kidney, and spleen of both young and old mice, although the levels were much lower than those found in the pituitary gland. In particular, liver expression of GnRHR was higher in young mice, whereas thymus expression was higher in older mice. Previous studies showed that the mRNA and protein levels of GnRH and GnRHR were altered in a tissue-specific manner in the maternal thymus, lymph node, spleen and liver during early pregnancy in sheep, suggesting that their levels may be altered with age (Cao et al., 2021). Other research has provided insight into the important role of GnRH in thymic development, particularly during the fetal and early postnatal stages, demonstrating the role of GnRH in regulating thymic development in rats (Melnikova et al., 2019). These findings support our results showing that GnRHR mRNA is expressed in extrapituitary organs, including the liver and thymus, suggesting a broader physiological role for this receptor beyond its primary function in the reproductive system (Cheung & Wong, 2008; Aguilar-Rojas & Huerta-Reyes, 2009).
Ovarian aging is an important aspect of reproductive biology with implications for female fertility, overall health, and disease risk. It refers to the natural decline in the quantity and quality of a woman’s ovarian follicles and oocytes with age (Moghadam et al., 2022; Wang et al., 2023). Similarly, uterine aging is also an important aspect of women’s reproductive and overall health, involving changes in the structure, function, and regenerative capacity of the uterus over time. Although not as extensively discussed as ovarian aging, uterine aging also plays a critical role in fertility, pregnancy outcomes, and overall health (Deryabin & Borodkina, 2023; Wu et al., 2023). Both processes are complex and influenced by various factors, including the accumulation of cellular senescence, mitochondrial dysfunction, stem cell exhaustion, and altered intercellular communication (Nelson et al., 2013; Shirasuna & Iwata, 2017). Given the changes in intercellular communication observed in the ovary and uterus with aging, we hypothesized that GnRHR expressed in these organs may undergo alterations as part of the aging process. Therefore, we examined its expression in these organs in both young and aged mice, to elucidate the relationship between GnRHR mRNA expression in the ovary and uterus and the age-related decline in reproductive function in female mice. Our results showed that GnRHR expression was lower in both the ovary and uterus of aged mice compared to young mice. In particular, GnRHR expression in the uterus was dramatically reduced in aged mice compared to young mice. These results were supported by previous studies showing the expression of GnRHR mRNA in the mouse ovary and uterus with aging (Peng et al., 1994; Fraser et al., 1996).
Next, we examined the expression levels of GnRH and GnRHR mRNA after injection with high doses of PMSG to elucidate whether high-dose gonadotropins regulate the expression of GnRHR genes in the ovary and uterus. Our results showed a significant decrease in GnRHR mRNA expression levels in the ovary, while a significant increase was observed in the uterus after PMSG administration. Interestingly, GnRH mRNA expression levels were increased in the ovary, but decreased in the uterus after PMSG administration, in contrast to the GnRHR expression results. Many previous studies support our findings that gonadotropins may be involved in GnRH expression and function in the ovary and uterus. It has been reported that GnRHR system are expressed in female reproductive tissues like the endometrium and ovary, indicating their roles in processes such as embryo implantation and follicular development (Maggi et al., 2016). Moreover, gonadotropins are known to act directly or indirectly on the ovary and uterus to stimulate the expression of GnRHRs in these organs of mice (Torrealday et al., 2013). The regulation of the GnRH/GnRHR system gene expression in the human ovary is influenced by gonadal steroids, gonadotropins, and GnRH itself. In ovarian cells, these components act in a paracrine/autocrine manner, influencing processes such as steroidogenesis, cell proliferation, and apoptosis (Metallinou et al., 2007). The expression patterns of GnRH and GnRH receptor mRNA in the ovary and uterus of the female rat vary throughout the estrous cycle, suggesting a dynamic regulation of these components in relation to the reproductive cycle (Schirman-Hildesheim et al., 2005).
In summary, GnRHR mRNA was detected in various organs, with the highest levels in the pituitary gland. Young mice showed significant GnRHR expression in the heart and liver, while aged mice showed increased expression in the thymus, lung, and small intestine. Kidney and spleen also exhibited notable GnRHR expression in both young and aged mice. Quantitative RT-PCR revealed that the pituitary gland had the highest GnRHR expression, but with notable differences between young and aged mice, including that liver expression was higher in young mice, whereas thymus expression was higher in aged mice. Moreover, GnRHR mRNA was present in the ovaries of both young and aged mice but was almost undetectable in the uterus of aged mice. In addition, a significant decrease in GnRH mRNA levels was observed in the ovary, while an increase was seen in the uterus after PMSG administration. The expression of GnRH mRNA in these organs showed opposite trends to that of GnRHR expression. These results suggest the involvement of GnRH in age-related reproductive decline and the potential effects of high-dose gonadotropin treatments on reproductive organ function. The study contributes to understanding the impact of aging and hormonal treatments on GnRH signaling pathways, which are crucial for reproductive function and health.