INTRODUCTION
Mainstream of research on the endocrine disrupting chemicals (EDCs) has been reproductive toxicity, which is about the adverse effects of sex steroid-like substances on the hypothalamic-pituitary-gonadal (HPG) hormone axis and its target organs (Czarnywojtek et al., 2023). Since mammalian adrenal glands have steroid receptors, and share a high similarity with gonads in synthesize and secrete steroid hormones from cholesterol, the glands could be suitable for research on EDCs (Hinson & Raven, 2006; Lagunas et al., 2022). In fact, EDC studies on the adrenal gland have been gradually accumulating, although in much smaller amounts than on the gonads; these are including adrenal steroidogenesis disruption by diethylstilbestrol (DES) (Haeno et al., 2015), genistein-induced histomorphometric and hormone secreting changes in the adrenal cortex (Ajdžanović et al., 2009), corticosterone secretion changes by low-dose bisphenol-A (Lan et al., 2015), reduced pubertal development of cortex by triphenyltin chloride (Li et al., 2020), and disruption of cortical function by dipentyl phthalate (Chen et al., 2023). Previously, we also demonstrated that a chronic low-dose effect of Di (2-ethylhexyl) phthalate altered the histological characteristics and steroidogenic gene expression of adrenal gland in female mice (Lee et al., 2021), and nonylphenol (NP) exposure resulted in histological changes with sexually dimorphic pattern in rat adrenal glands (Kim & Lee, 2022). In the present study, we hypothesized that the structural changes are closely linked to functional changes, especially secretory function in rats, so the hormonal secretory patterns of NP treated adrenals might be changed. To test this hypothesis, we developed the short-term adrenal incubation method, and measured the catecholamines and cortical steroids in NP-treated media using the high-performance liquid chromatography (HPLC) coupled with electrochemical detector (ECD) and specific enzyme-linked immunosorbent assay (ELISA), respectively.
MATERIALS AND METHODS
Sprague-Dawley rats were provided by DBL (Eumseong, Korea) and reared in Sangmyung University animal facility under photoperiods of 12h light/dark with lights on at 7 AM and constant temperature of 21°C–23°C. Food and tap water were supplied ad libitum. The animal protocols were approved by the Animal Care and Use Committees at Sangmyung University (2018-03; approval number R-1801-1). All the animals received human care in accordance with the guides for animal experiments of the Association for Assessment and Accreditation of Laboratory Animal Care (AAALAC) International.
The short-term organ culture was based on previous report (Mandon et al., 1987) with minor modification. Briefly, the adrenal glands of 8 weeks-old female and male rats were pre-incubated with a Krebs-Ringer Bicarbonate (KRB; Sigma-Aldrich, St. Louis, MO, USA) medium for 1 hour at 37°C in incubator (BINDER, Tuttlingen, Germany) aerated with 95% air and 5% CO2. Then the glands were shifted in fresh media and treated with NP (100 pM, 1 nM, 10 nM, 100 nM or 1 μM) for 1 hour at 37°C. After incubation, the media were centrifuged (10,000×g for 30 min at 4°C, Hanil, Gimpo, Korea) and the supernatants were stored at –80°C until analysis.
The levels of corticosterone and aldosterone were measured by specific ELISA following supplier’s protocols (MyBioSource, San Diego, CA, USA). Briefly, standard and samples (50 μL) were added to each well and then add the detection reagent A solution (50 μL) immediately. The plate was incubated for 1 hour at 37°C, then the liquid was aspirated and washed 3 times with the wash solution (300 μL). After last washing, the remaining liquid was removed completely by aspirating or decanting and the detection reagent B solution (50 μL) was added to each well. The plate was incubated for 1 hour at 37°C. After incubation, the plate was washed 5 times as conducted previous washing step. The substrate solution (90 μL) was added to each well and the plate was incubated for 25 minutes at 37°C. When the liquid turned blue with the addition of the substrate solution, the stop solution (90 μL) was added to each well and mixed by tapping the side of the plate. The plate was measured at 450 nM using microplate reader immediately. The detection range of the corticosterone ELISA kit was 7.812–500 ng/mL and the aldosterone kit was 0.39–25 ng/mL, respectively.
Measurements of catecholamine, including norepinephrine (NE) and epinephrine, were conducted with reverse-phase high-performance liquid chromatography with electrochemical detection (RP-HPLC-ECD) using methods modified from Wood & Hall (2000). The concentration range of NP treatment was determined through our preliminary experiments to reflect realistic exposure in daily life. The samples were centrifuged at 10,000×g for 10 min at 4°C to remove precipitated proteins. The supernatants were diluted by mobile phase buffer [0.01 N Formic acid (Shinyo Pure Chemicals, Osaka, Japan), 0.05 mM 1-Octanesulfonic acid sodium salt (Sigma-Aldrich), 10% Methanol (Honeywell, Morristown, NJ, USA)] before determining catecholamine content. The mobile phases were prepared as needed and degassed by sonicator (KODO Technical Research, Hwaseong, Korea) prior to use. Standards and samples were analyzed at room temperature using 5 μm Waters Xbridge C18 (150×4.6 mm I.D.) column (Waters, Milford, MA, USA). Injection volumes of samples and standard were 10 μL (2707 Auto sampler; Waters). The flowrate was 1 mL/minute over 20 minutes with an isocratic elution (1525 Binary HPLC Pump; Waters). Retention times of NE, epinephrine and dopamine were about 7, 9, and 11 minutes, respectively. Electrochemical detection was performed using model 2464 ECD [Waters; in situ Ag/AgCl (ISAAC; [Cl−]=0.1 M)].
RESULTS AND DISCUSSION
The NE and epinephrine levels of media were measured by HPLC. The NE levels of NP-treated media, except 100 pM NP, in which a female adrenal gland was cultured were significantly increased [Fig. 1A; control (CTL) vs 1 nM NP, 64.5±0.6 vs 68.5±0.2 ng/μL, p<0.001; vs 10 nM NP, vs 68.3±1.0 ng/μL, p<0.05; vs 100 nM NP, vs 69.8±0.2 ng/μL, p<0.001; vs 1 μM NP, vs 71.2±1.1 ng/μL, p<0.01]. The NE in male adrenal glands was more released when treated with 100 nM and 1 μM NP (Fig. 1C; CTL vs 100 nM NP, 49.8±3.0 vs 68.7±7.8 ng/μL, p<0.05; vs 1 μM NP, vs 59.3±0.8, p<0.05). However, epinephrine levels in both male and female adrenal media were not significantly changed (Fig. 1B and D).
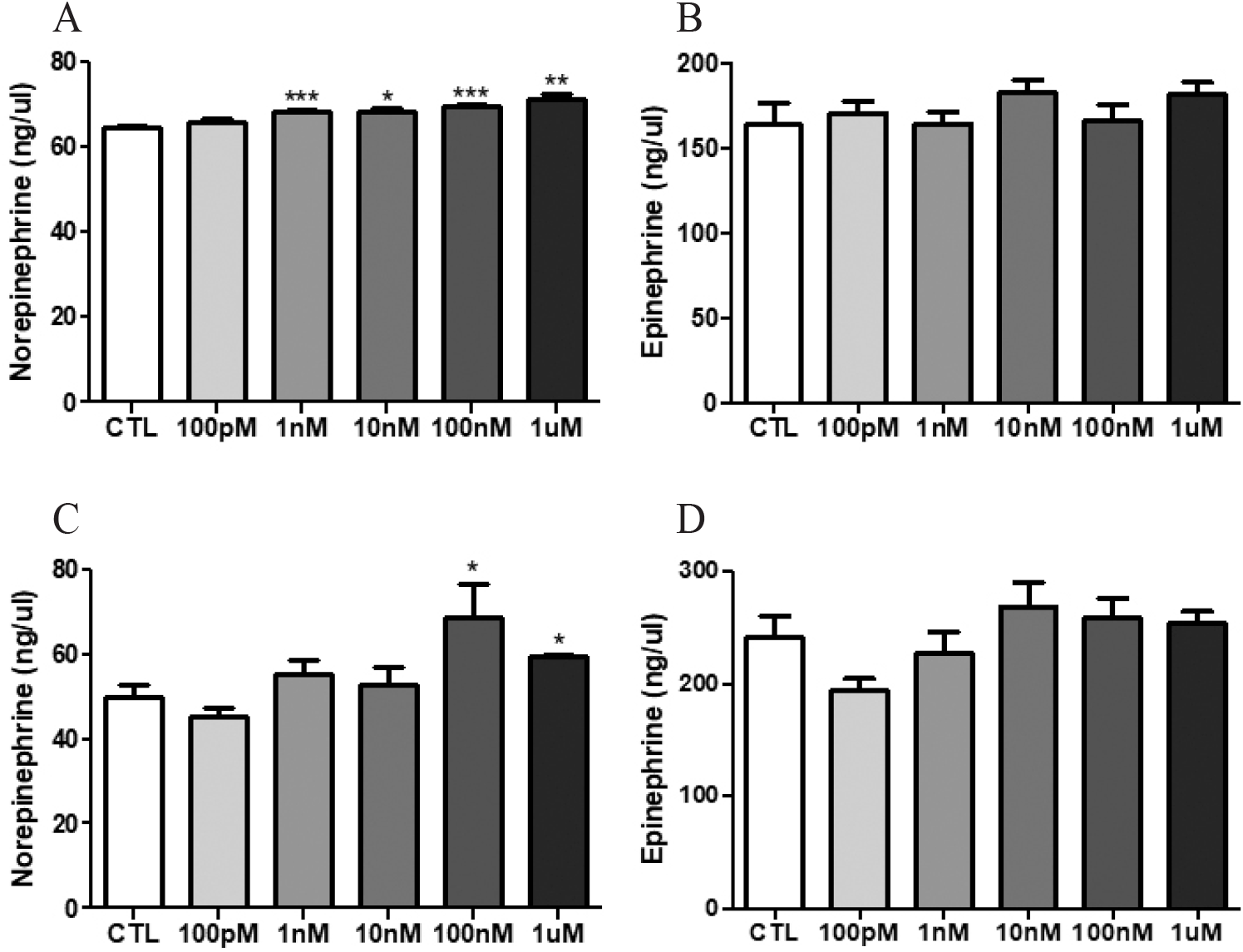
The levels corticosterone and aldosterone in media were analyzed by ELISA. Significant changes of corticosterone levels in media from cultured female adrenal glands were not found (Fig. 2A). The corticosterone level of male adrenal media only treated with 100 pM NP was significantly lowered (Fig. 2C; CTL vs 100 pM NP, 139.4±1.6 vs 134.6±1.6 ng/mL, p<0.05). The aldosterone level in the female adrenal media treated with 100 pM NP was significantly decreased, while the level of media treated with 10 nM NP was significantly increased, showing an inverse manner (Fig. 2B; CTL vs 100 pM NP, 3.3±0.4 vs 2.6±0.03 ng/mL, p<0.05; vs 10 nM NP, vs 5.6±0.2 ng/mL, p<0.01). In male adrenal study, the aldosterone levels of 10 nM, 100 nM and 1 μM NP-treated media were significantly declined (Fig. 2D; CTL vs 10 nM NP, 4.7±0.3 vs 2.4±0.3 ng/mL, p<0.001; vs 100 nM NP, vs 2.2±0.2 ng/mL, p<0.001; vs 1 μM NP, vs 2.3±0.2 ng/mL, p<0.001).
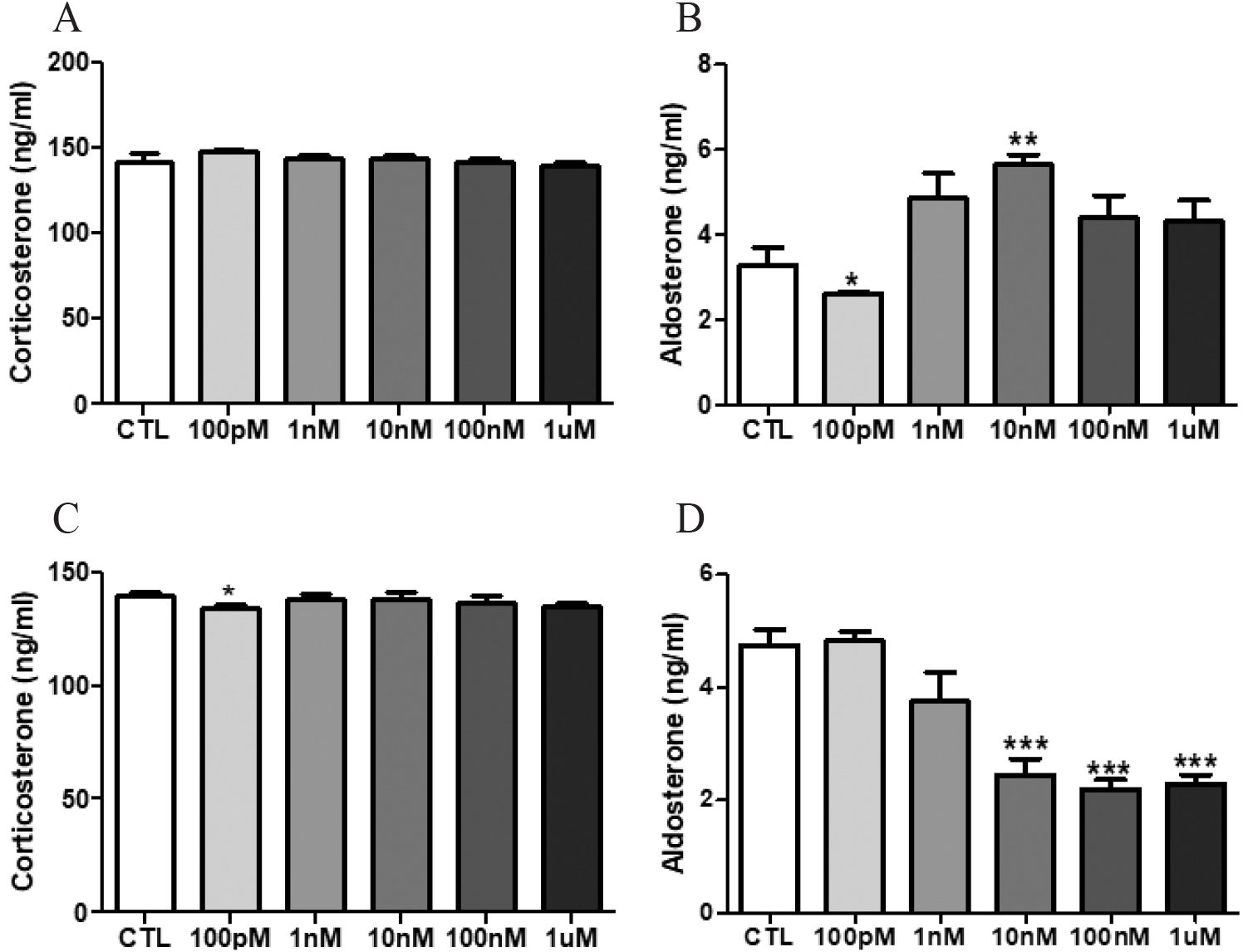
In the present study, we developed rat adrenal organ culture system with simple serum-free KRB media for short-term period, and found this medium was suitable for detection of catecholamines and steroids, so EDC activity of NP could be easily verified. Treatment with NP altered secretory pattern of aldosterone from adrenals of both sexes. Intriguingly, NP treatment had a stimulatory effect on the female adrenal gland while had an inhibitory effect on the male adrenal gland, showing sexual dimorphism. In general, EDC has been known to have a negative effect on both males and females. Interestingly, these results suggest the possibility that the pathological effects of NP on rat adrenal glands are likely to occur only in one sex. In addition, NP treatment shown stimulatory effects on the NE secretions from adrenal medulla of both sexes, particularly resulted in more sensitive response at lower concentration in female adrenal gland, suggesting possible relationship between NP exposure and autonomic nervous system disorders.
It is now clear that hypothalamus-pituitary-thyroid, HPG, hypothalamus-pituitary-adrenal glands represent the main targets of EDCs (Gore et al., 2015; Kabir et al., 2015). Among these organs on the hormone axes, adrenal glands present favorable structural and biochemical features that make them ideal targets for EDCs (Lauretta et al., 2019). Adrenal glands, like gonads, are solid organ with a mechanism to synthesize and secrete steroid hormones and catecholamines. However, compared to the studies on gonads, only a small number of research have assessed the effects of EDC on the adrenal gland, although maintaining proper function of adrenal glands is crucial for maintaining life (Harvey, 2016). Nevertheless, data is gradually accumulating that major endocrine disruptors with reproductive toxicity also have adverse effects on the adrenal glands (Egalini et al., 2022). Adrenal glands possess estrogen receptors (ERalpha and ERbeta) and androgen receptor (AR), so estrogen-like or anti-androgenic substances can act as or disrupt the original ligands (Trejter et al., 2015). For example, the strong synthetic estrogen DES, which is known to bind ERs, substantially decreased the blood and adrenal corticosterone levels without any histological alteration (Haeno et al., 2015). Genistein, one of soybean phytoestrogen, can cause the shunting of metabolic pathways in the adrenals of orchidectomized middle-aged rats, stimulating dehydroepiandrosterone secretion and inhibiting corticosterone and aldosterone secretion (Ajdzanović et al., 2009). Treatment of mouse Y1 adrenal cortex cells with low doses of bisphenol-A (10–1,000 nM) increased promoter activity and protein expression of Cyp11a1, induced c-Jun phosphorylation, and increased corticosterone production (Lan et al., 2015). Oral administration of triphenyltin (0–2 mg/kg/day, for 18 days) inhibits production of corticosterone via blocking the expression of cholesterol uptake transporters and cholesterol biosynthesis in male pubertal rats (Li et al., 2020). In utero exposure to Di-n-pentyl phthalate (DPeP, 0–500 mg/kg/day) significantly reduced serum aldosterone and corticosterone levels, markedly reduced the thickness of zona glomerulosa, and significantly downregulated the expression of steroidogenesis- and apoptosis-related genes (Chen et al., 2023). Previously we also demonstrated that low-dose NP exposure induced early beginning of puberty and pathohistological abnormalities in ovary and uterus of F1 mice (Kim et al., 2020), and adrenal histology could be altered in F1 rats (Kim & Lee, 2022).
Among EDCs, NP has been relatively well studied using both in vivo and in vitro model systems. For example, NP exposure in developmental periods results in hyperadrenalism and increasing 11β-hydroxysteroid dehydrogenase I (11β-HSD1) activity in an adult rat model (Chang et al., 2010, 2018). In rat adrenal zona glomerulosa cell culture, treatment with NP increased aldosterone release, and this stimulatory effect seemed to be mediated through steroidogenic acute regulatory protein and aldosterone synthase activity (Chang et al., 2012). Another in vitro study (Yanagihara et al., 2005) revealed that NP significantly stimulated the catecholamine biosynthesis from tyrosine even at 0.1 nM and maximally at 10 nM, and increased tyrosine hydroxylase activity in the cultured bovine adrenal medulla cells. The in vitro cell/organ culture system including our own might provide easier evaluation of EDC activities and render much clear interpretation on biosynthesis/secretion of cortical steroids and medullary catecholamines.
The reproductive organs such as ovaries and testes are representative examples of sexual dimorphism. On the other hand, it is very difficult to distinguish between male and female adrenal glands in morphological aspect. However, from a functional perspective, adrenal glands are a good example of a non-reproductive sexually dimorphic organ. Indeed, most adrenal lesions occur in females rather than males such as Cushing’s syndrome (Levasseur et al., 2019). In particular, the adrenal cortex is a dynamic portion in which senescent cells are continuously replaced by newly differentiated ones, and this constant renewal facilitates organ remodeling in response to physiological demand for different cortical steroids (Pihlajoki et al., 2015; Kim & Lee, 2022). Therefore, 3 times higher in adrenocortical cell renewal in female rodents appears to be a key mechanism for sexual dimorphism in the adrenocortical morphology and hormone secretion patterns (Lyraki & Schedl, 2021). Previously we demonstrated that low-dose NP treatment induced sexually dimorphic pattern of adrenocortical shrinkage in F1 rats, suggesting the dimorphic pattern of the area-related cortical steroids biosynthesis and/or secretion in the animals (Kim & Lee, 2022). Further, focusing on the dimorphic nature of adrenocortical steroid and/or catecholamines will be a great help not only in gathering basic knowledge but also in understanding the clinical applications. For example, one could try to find the sex-specific nature and cure of adrenal disorders such as Conn syndrome (a hyperaldosteronism occurs when the adrenal glands release too much aldosterone) and Addison’s disease (also called adrenal insufficiency, a condition with too little cortisol or aldosterone).