INTRODUCTION
The male reproductive tract is consisted of the testis, excurrent ductules, and accessory glands, including prostate and seminal vesicle. The epididymis is a part of excurrent ductules, which the testis is connected via efferent ductules (Robaire et al., 2006). The epididymis has a tubular structure, which the lumen is located inside and is surrounded by a layer of epithelial cells (Robaire et al., 2006). The epididymis is anatomically divided into four parts, including initial segment, caput (head region) epididymis, corpus (body region) epididymis, and cauda (tail region) epididymis, which are characterized by the presence and different frequency of specific cell types and different histochemical existence of enzymatic molecules (Robaire et al., 2006). The epididymal cell types present within the epithelial layer are principal, apical, narrow, clear, basal, and halo cells (Robaire et al., 2006). Even though the principal cell is found along the entire epididymal ductules, the restricted localization of other cell types is commonly observed (Robaire et al., 2006). For example, the apical and narrow cells are mainly found in the initial segment (Sun & Flickinger, 1980), while the clear cells only exist in the caput, corpus, and cauda epididymis (Abou-Haïla & Fain-Maurel, 1984). In addition, a number of researches have demonstrated that these cell types have distinctive morphologies, functions, and localization relative to other cell types (Robaire et al., 2006). Thus, it is generally considered that each epididymal region plays different roles on control and regulation of epididymal functions.
The epididymis is the site in which immature spermatozoa produced from the testis become mature and acquire motility and fertilizing capacity (Robaire et al., 2006). The physiological functions of epididymis include transport and maturation of spermatozoa and storage and protection of spermatozoa (Robaire et al., 2006). The regulation of water movement into and out of the epididymal lumen is a way to form the adequate luminal microenvironment for sperm maturation (Robaire et al., 2006). Even though most of testicular fluid is reabsorbed in the efferent ductules (Clulow et al., 1994), luminal water reabsorption at significant level occurs in the epididymis, especially in the caput epididymis (Tao et al., 2000). Other scientific evidences have also shown that not only fluid reabsorption from the epididymal lumen but also water secretion into the lumen are equally important to regulate the luminal composition of the epididymis and the fluidity of the epididymal luminal content (Wong, 1998). Thus, the controlled transepithelial water movement is important to regulate sperm maturation of epididymal function.
Aquaporins (AQPs) are water channel molecules that are chiefly localized at plasma membrane in various cell types, including testis, efferent ductules, and epididymis in the male reproductive tract (Verkman & Mitra, 2000; Da Silva et al., 2006). AQPs have 6 membrane-spanning domains in structure and contribute to water movement across plasma membrane (Verkman & Mitra, 2000; Ribeiro et al., 2021). The AQPs have at least 13 isoforms in mammalian cells, and expression and localization of different types of AQPs in the male reproductive tract have been extensively examined (Da Silva et al., 2006; Ribeiro et al., 2021). AQPs are also capable of transporting ammonia or hydrogen peroxide, depending on types of AQPs (Da Silva et al., 2006; Ribeiro et al., 2021). There are at least 8 types of AQPs expressing in the epididymis, and specific expression of different AQP types has been detected along the epididymis (Ribeiro et al., 2021). For examples, expression of AQP1 is found in smooth muscle and endothelial cells adjacent to epididymal epithelial cells (Brown et al., 1993), and AQP9 is exclusively localized at the apical microvilli of principal cells throughout the entire epididymal region (Pastor-Soler et al., 2001). The expression of AQPs in the male reproductive tract is regulated by various factors, including androgen and estrogen (Badran & Hermo, 2002; Pastor-Soler et al., 2002; Oliveira et al., 2005). Because expressional alterations of AQPs in male reproductive tract are associated with the reduction of reproductive capability (Huang et al., 2006), it is relatively reasonable to consider that exogenous compound affecting male fertility could influence expression of AQPs in the male reproductive tract.
Nandrolone decanoate (ND) is an androgenic-anabolic steroidal compound which is frequently administrated to treat several clinical symptoms, such as muscle wasting accompanied with human immunodeficiency virus infection and anemia involved in chronic renal failure (Busardò et al., 2015). Uncontrolled and unregulated usage of ND is strongly associated with the reduction of male fertility, probably due to expressional alteration of steroidogenic enzymes in the testis in part (Min & Lee, 2018). In addition, chronic administration of ND results in a decrease of epididymal weight (Mirkhani et al., 2005), even though the effect of ND on the epididymal has not determined in detail. Non-clinical use of ND among non-professional people and bodybuilders appears to be increased (de Souza & Hallak, 2011). But, despite deleterious undesired outcomes on male fertility by the misuse of ND, the effect of ND on the epididymis has been rarely studied.
The present research was focused to determine the effect of ND treatment at different doses for short or long period on expression of Aqp1 and Aqp9 in the rat epididymis. The segmental expressional modulation of Aqp1 and Aqp9 by ND treatment was examined by using a quantitative real-time polymerase chain reaction (PCR) analysis.
MATERIALS AND METHODS
A total of 34 male Sprague Dawley rats at 40 days of age were purchased from Samtako (Osan, Korea) and individually caged during an entire experimental period. The animals were allowed ad libitum access to food and water and kept in controlled conditions. The ND, 50 mg/mL concentration in a stock solution, was obtained from Organon Korea (Seoul, Korea), and diluted into adequate concentrations with peanut oil for ND treatment.
The equal number of animals at 50 days of age were divided into two different treatment groups, 2 week-ND treatment and 12 week-ND treatment groups. The animals in each experimental group were further randomly assigned into one of three subgroups, including control (peanut oil treatment, n=5), low dose-ND treatment (2 mg of ND/kg body weight/week, n=6), or high dose-ND treatment (10 mg of ND/kg body weight/week, n=6). The first subcutaneous ND injection was started at 50 days of age, and the injection amount of peanut oil or ND was adjusted every week by measuring body weight of animal.
After the last injection was allowed at the day of 2nd or 12th week, the animal was euthanized by over-exposure to CO2 in a closed chamber. The reproductive tract, including testis and epididymis, was pulled out through an incision made on the scrotum. The epididymis was rapidly separated from the testis and efferent ductules in cold phosphate-buffered saline (PBS) solution. The epididymis transferred into a fresh PBS solution was further dissected out into initial segment, caput epididymis, corpus epididymis, and caudal epididymis. The epididymal segments were quickly frozen in liquid nitrogen and stored in −80°C until further use.
Total RNA from the frozen tissue was extracted with Trizol reagent (Molecular Research Center, Cincinnati, OH, USA). Shortly, the tissue was rapidly homogenized in Trizol solution, and chloroform and isopropanol were sequentially supplied to precipitate total RNA. The concentration of total RNA re-suspended in DEPC-dH2O was measured by NanoDrop Lite spectrophotometer (Thermo Scientific, Massachusetts, MA, USA). The quality of total RNA was determined by 1.2% agarose gel electrophoresis.
One microgram of total RNA was used to generate the first strand complementary DNA (cDNA) strand by utilizing iScripTM Reverse transcription Supermix for reverse transcription (RT)-qPCR (Bio-Rad Laboratories, Hercules, CA, USA). A total volume of the mixture with total RNA was adjusted with nuclease-free dH2O to 20 μL. The RT reaction was carried out at 25°C for 5 min, 46°C for 20 min, and 95°C for 1 min as instructed in the protocol.
The quantitative real-time PCR was performed with a mixture of 1 μL of cDNA, 7 μL of iQTM SYBR® Green Supermix (Bio-Rad Laboratories), 10 pmol of each oligonucleotide primer, and nuclease-free dH2O to make a total volume of 25 μL. The information of oligonucleotide primer used for real-time PCR is shown in Table 1. As an internal control for PCR analysis, cyclophilin A (Ppia) was used. The PCR was executed in a pre-denaturation step at 95°C for 5 min, cycles of a denaturation step at 95°C for 30 sec, an annealing step at Tm for 30 sec, and an extension step at 72°C for 30 sec in PTC-200 Chromo 4 real-time system (Bio-Rad Laboratories). At the end of each PCR, an extra extension step at 72°C for 10 min was added. The PCR product was fractured in 1.2% agarose gel electrophoresis to check the size.
PCR, polymerase chain reaction; Aqp1, aquaporin 1; Aqp9, aquaporin 9; and Ppia: cyclophilin A.
The levels of Aqp1 and Aqp9 transcripts in each experimental groups were adjusted to that of Ppia transcript level. The relative ratios of Aqp1 and Aqp9 expression levels among experimental groups were obtained from 2-ΔΔCt method (Livak & Schmittgen, 2001). Independently triplicated or quadruplicated RT reactions and PCRs were performed to acquire a mean and standard error of an experimental groups, and the data were presented in the relative ratio of Aqp1 or Aqp9 transcript level against that of control group. The one-way ANOVA was employed to determined the existence of statistical differences at transcript level of Aqp1 or Aqp9 among different experimental groups. If a significance was detected, a post-hoc test, Duncan’s test, was followed. Less than 0.05 level of probability was considered to be statistically different.
RESULTS
The transcript level of Aqp1 in the initial segment was not significantly changed by ND treatment for 2 weeks (Fig. 1A). The similar finding was observed from Aqp9 transcript level treated with ND for 2 weeks (Fig. 1B). However, a significant decrease of Aqp1 expression level was detected in the initial segment by ND treatment at the low dose for 12 weeks (Fig. 1C). The level of Aqp1 transcript at the high dose-treated group of ND for 12 weeks was also significantly lower than that of control group, even though there was no statistical difference between the low and high dose-treated groups of ND (Fig. 1C). The level of Aqp9 transcript was not significantly changed by ND treatment at the low dose for 12 weeks, while the high dose treatment of ND for 12 weeks resulted in a decrease of Aqp9 expression in the initial segment (Fig. 1D).
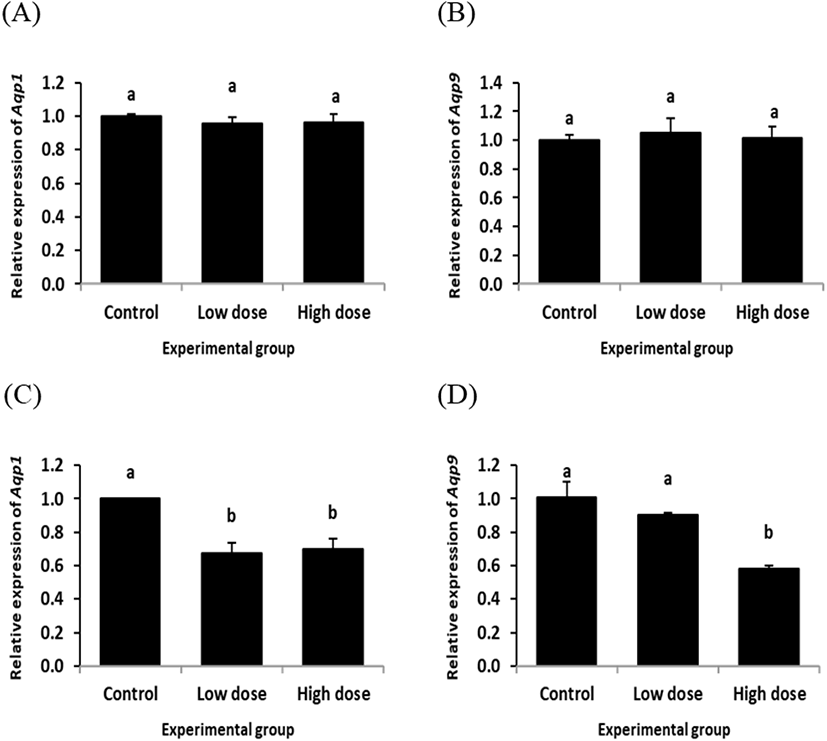
The expression of Aqp1 in the caput epididymis with ND treatment for 2 week was not significantly changed, regardless the dose of treatment (Fig. 2A). However, a significant decrease of Aqp9 transcript level was detected in the caput epididymis with ND treatment at the low dose for 2 weeks, while there was no significant change of Aqp9 transcript level at the high dose ND treated-group for 2 weeks (Fig. 2B).
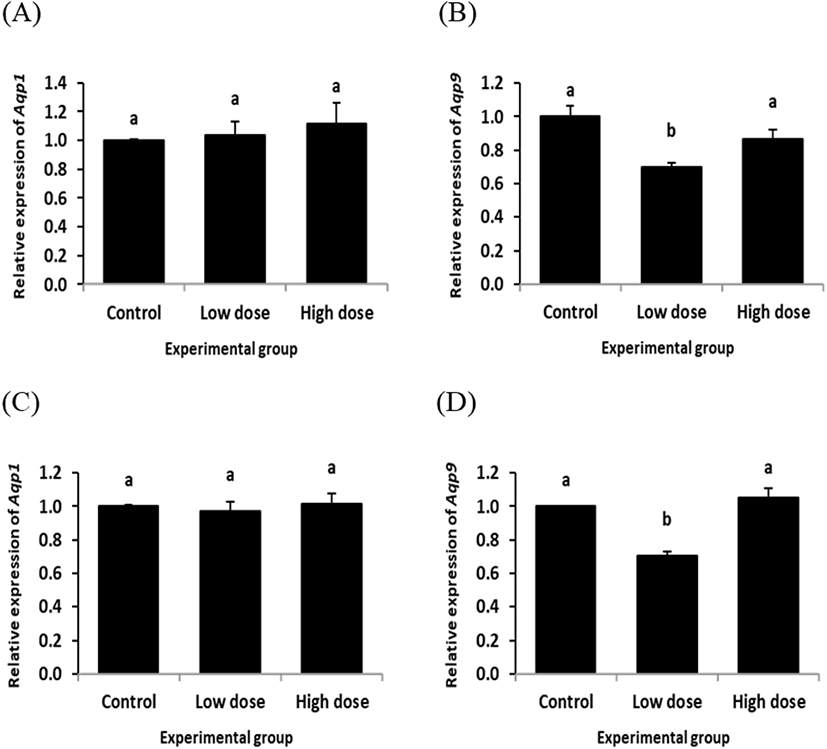
The expression patterns of Aqp1 and Aqp9 in the caput epididymis by ND treatment for 12 weeks (Fig. 2C and D) were quietly similar with those of ND treatment for 2 weeks (Fig. 2A and B). That is, the ND treatment for 2 weeks didn’t give an influence on the level of Aqp1 transcript at any dose (Fig. 2C). But, the low dose treatment of ND for 12 weeks induced a reduction of Aqp9 expression in the caput epididymis, even though there was no significant expression change of Aqp9 observed with the high dose ND treatment for 12 weeks (Fig. 2D).
Fig. 3 shows the changes of Aqp1 and Aqp9 transcript levels in the rat corpus epididymis treated with ND for 2 or 12 weeks. The ND treatment at the low dose for 2 weeks resulted in a significant increase of Aqp1 transcript level in the corpus epididymis (Fig. 3A). A further increase of Aqp1 transcript amount was observed in the corpus epididymis exposed to the high dose of ND for 2 weeks (Fig. 3A). However, expression of Aqp9 was significantly decreased by ND treatment at the low dose for 2 weeks (Fig. 3B). The ND treatment at the high dose for 2 weeks caused an additional reduction of Aqp9 transcript level in the corpus epididymis (Fig. 3B).
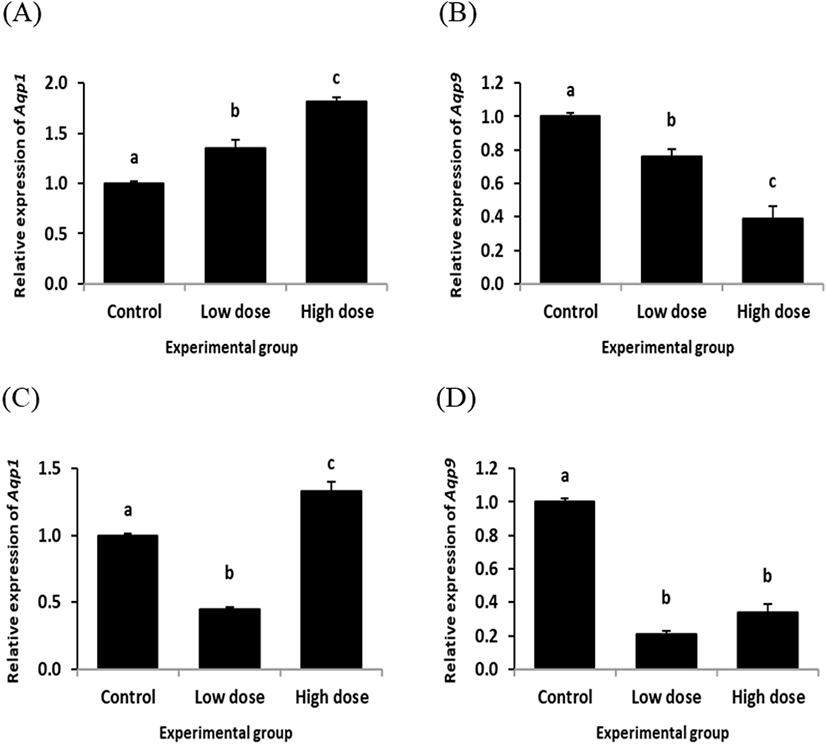
The level of Aqp1 in the corpus epididymis was significantly decreased by the low dose treatment of ND for 12 weeks (Fig. 3C). But, the Aqp1 transcript level in the corpus epididymis of the high dose ND treatment groups for 12 weeks was statistically higher than those of control and low dose ND treatment groups (Fig. 3C). Expression of Aqp9 in the corpus epididymis by ND treatment for 12 weeks was significantly decreased at both doses (Fig. 3D).
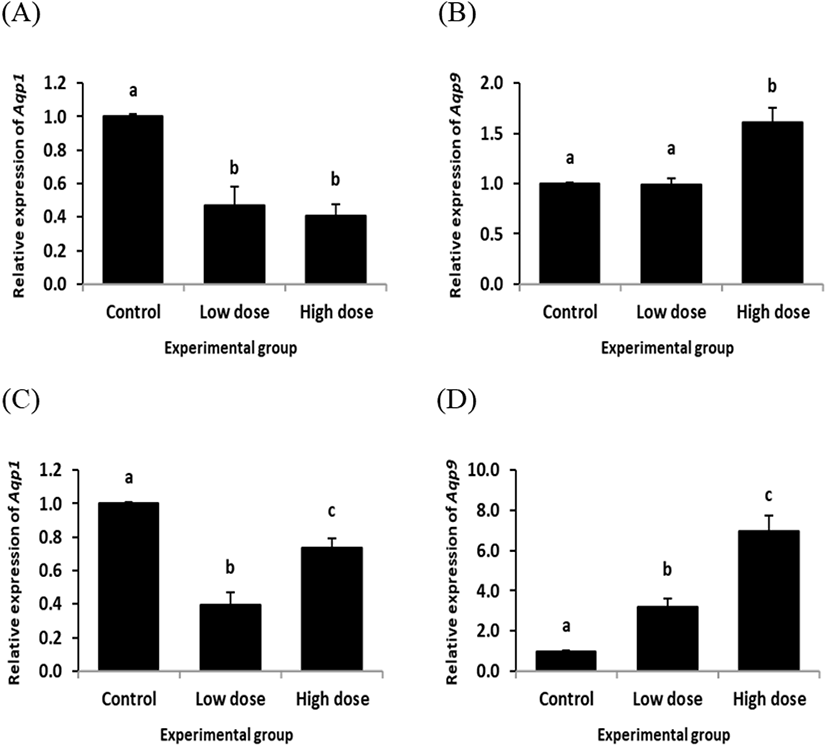
In the cauda epididymis, ND treatment for 2 weeks resulted in significant decreases of Aqp1 transcript levels at both doses (Fig. 4A). However, ND treatment at the low dose for 2 weeks didn’t influence on the level of Aqp9 transcript, even though the high dose treatment of ND for 2 weeks caused a significant increase of Aqp9 expression in the cauda epididymis (Fig. 4B).
Expression of Aqp1 in the cauda epididymis was significantly decreased by the low dose treatment of ND for 12 weeks (Fig. 4C). The level of Aqp1 transcript in the caudal epididymis treated with the high dose of ND for 12 weeks was significantly lower than that of control group, but higher than that of the low dose ND treatment group (Fig. 4C). The low dose ND treatment for 12 weeks resulted in a significant increase of Aqp9 transcript level in the cauda epididymis (Fig. 4D). An additional increase of Aqp9 expression in the cauda epididymis was observed with the high dose ND treatment for 12 weeks (Fig. 4D).
DISCUSSION
The present research examined expression changes of Aqp1 and Aqp9 in each epididymal segment by ND treatment at two doses for 2 or 12 weeks. The current findings are summarized as follows: 1) expression of Aqp1 and Aqp9 is differentially modulated among different epididymal segments by ND treatment, even at same dose for same period; 2) ND treatments at different doses usually result in similar outcomes on expression of Aqp1 or Aqp9 within a given epididymal segment; and 3) change patterns of Aqp1 and Aqp9 expression by ND treatment in an epididymal segment are not always same, even at same doses and for same period.
Hormonal regulation of Aqps expression in the excurrent duct of male reproductive tract has been demonstrated from other researches. Expression of Aqp9 is modulated by estrogen and 5α-dihydrotestosterone in the efferent ductules, but only by 5α-dihydrotestosterone in the initial segment (Oliveira et al., 2005). The nonsteroidal antiandrogen flutamide results in a decrease of Aqp9 expression in the epididymis (Pastor-Soler et al., 2002). The exposure to a steroidal estrogen receptor antagonist, ICI182,780, leads to a decrease of Aqp1 expression in the monkey caput epididymis (Shayu et al., 2005). In addition, 3-beta-diol, an androgenic metabolite, could influence on the regulation of Aqp9 expression in the efferent ductules (Picciarelli-Lima et al., 2006). Moreover, Badran & Hermo (2002) have shown that expression of Aqp9 in the initial segment and cauda epididymis appears to be regulated by testicular factor(s), not by androgen, suggesting differential regulation of specific Aqps at different epididymal segments. Thus, these observations indicate that expressional regulation of Aqps in the efferent ductules and epididymis is under control of various testicular and/or nontesticular factors. Also, expression of specific Aqp type in the epididymis could be regulated by segment-specific manner(s). Indeed, the present research have also shown differential expression patterns of Aqp1 and Aqp9 in different epididymal regions by same dose and/or duration treatment of ND. For example, the ND treatment for 2 weeks has not affected expression of Aqp1 and Aqp9 in the initial segment, while an increased level of Aqp1 transcript and a decreased level of Aqp9 transcript have been observed with same treatment in the corpus epididymis. Also, in the caput epididymis, even though the low dose treatments of ND for 2 and 12 weeks result in decreased levels of Aqp9 transcript, the high-dose ND treatments for same periods have not influenced on Aqp9 expression level. Together with the other’s findings, the current research data suggest that expression of Aqp1 and Aqp9 along the epididymis by the exposure to ND is differentially regulated in segment-specific and/or dose and treatment duration-dependent manners.
Such differential regulation of Aqp1 and Aqp9 by ND could be due to different distribution of androgen receptor (AR) concentration along the epididymis. Pujol & Bayard (1979) have shown that the caput epididymis has the highest AR concentration and the corpus epididymis has the lowest AR amount. Because ND has a similar affinity to AR comparable with testosterone (Bergink et al., 1985), ND could exert its biological effect via AR in a target tissue. Thus, it was expected that the caput epididymis would be more responsive than the corpus epididymis to ND exposure. However, more dramatic changes of Aqp1 and Aqp9 expression by ND treatment have been observed in the corpus epididymis, not in the caput epididymis. In addition, significant changes of transcript levels in the caput epididymis have been just detected in Aqp9, not Aqp1, exposed to the low dose of ND. A clear answer to such ambiguous data could not be provided at this moment. An additional investigation of the regulatory effect of ND on Aqp1 and Aqp9 expression in the epididymis is suggested.
It is also suggested that expressional change of Aqp1 and Aqp9 by ND treatment in the epididymis could be not direct action of ND but indirect effect accompanied with systemic response to ND. The exposure to ND frequently accomplishes aberrant expression of steroidogenic enzymes and abnormal histology in the testis (Svechnikov et al., 2010; Min & Lee, 2018). In fact, a previous research has demonstrated that ND administration in a same experimental condition with the present study results in significant reduction of several testicular steroidogenic enzymes, including steroidogenic acute regulatory protein, cytochrome P450 side chain cleavage, and cytochrome P450 17α-hydroxylase, accompanied with a drastic decrease of serum testosterone concentration (Min & Lee, 2018). Because functions of the epididymis are largely influenced by testosterone (Robaire et al., 2006), a decrease of testicular testosterone synthesis by ND exposure could give an impact on gene expression in the epididymis to result in functional abnormality. However, even though this suggestion seems to be supported by dose-related decreases of Aqp9 transcript levels in the corpus epididymis by 2 week-ND treatment, other data don’t seem to fit to the presumption.
The present research shows that expressional regulation of Aqp1 and Aqp9 in the epididymis by ND is quite complicate. The existence of complex regulatory mechanism of Aqp1 and Aqp9 in the epididymis by hormonal factor has also been proposed from others (Badran & Hermo, 2002; Oliveira et al., 2005; Squillacioti et al., 2021). Considering the main function of the epididymis and physiological role of AQPs in the epididymis, it is suggested that the aberrant expression of Aqp1 and Aqp9 in the epididymis by ND treatment would cause inappropriate development of mature spermatozoa, thus leading into ND-induced infertility. In addition, a possible segment-specific regulation of Aqp1 and Aqp9 expression in the epididymis by ND administration is suggested from the current study, even though detailed molecular mechanism(s) would be determined from further researches.