INTRODUCTION
Equine chorionic gonadotropin (eCG), also known as pregnant mare serum gonadotropin (PMSG), has the unique characteristic of exhibiting both luteinizing hormone (LH)- and follicle-stimulating hormone (FSH)-like activities in non-equid species (Chopineau et al., 1993; Apparailly & Combarnous, 1994; Min et al., 1996, 2019; Legardinier et al., 2008; Galet et al., 2009). However, in horses, eCG exhibits only LH activity (Galet et al., 2009).
eCG belongs to the glycoprotein hormone family, which includes LH, FSH, and thyroid stimulating hormone (TSH) (Min et al., 1996, 2004, 2019, 2020). Of all known glycoprotein hormones, eCG also has the highest carbohydrate content (>40% of molecular weight).
CG has been reported to be expressed in primate and Equidae species during early pregnancy. In particular, eCG is secreted from endometrial cups between days 37 and 120 of pregnancy (Boeta & Zarco, 2012; Flores-Flores et al., 2014; Conley, 2016). The glycoprotein hormones from the pituitary and placenta comprise two non-covalently linked α-subunits and β-subunits. Glycoproteins have the same α-subunits but different β-subunits. In primates, the CGβ and LHβ genes are different (Murphy & Martinuk, 1991); however, in horses, eCGβ and eLHβ encode a single gene (Sherman et al., 1992; Min et al., 1994, 1996). There is a specific difference between eCG and eLH in the structure of their glycoresidues, which are both sialylated and sulfated in eLH and sialylated in eCG.
The eCG α-subunit has two N-linked glycosylation sites at Asn56 and Asn82, and its β-subunit has one glycosylation site at Asn13. In addition to these N-glycosylation sites, the eCG β-subunit has a heavily glycosylated carboxyl-terminal peptide (CTP) region, extending the lengths of the β-subunits from 121 to 149 amino acids (Talmadge et al., 1984; Crawford et al., 1986). Analysis of a purified preparation of eCG revealed that the eCG β-subunit contains 149 amino acids (Sugino et al., 1987). There are least 11 O-glycosylation sites on the extended CTP of the eCG β-subunit (Bousfield et al., 1985; 1992; Hokke et al., 1994). hCG, secreted from the human placenta during early pregnancy, has four O-glycosylation sites and is located on amino acids 121 to 145 of the extended CTP region. Therefore, eCG is a distinct molecule for biological activity and the structure of its glycoresidues. We previously reported several studies on eCG, including cDNA cloning of the eCG α-subunit and β-subunit, biological dual activities of LH-like and FSH-like in cells expressing rat LHR and rat FSHR (rLHR and rFSHR), and ovulation rate in vivo (Min et al., 1994, 1996, 2004; 2019, 2020; Park et al., 2017; Kim et al., 2018; Byambaragchaa et al., 2021a,b,c; Lee et al., 2021).
Gonadotropin receptors, like eLH/CGR, belong to a large superfamily of G protein-coupled receptors (GPCRs) characterized functionally by their interaction with guanine nucleotide-binding proteins and structurally by their seven transmembrane spanning domains, extracellular amino-terminus, and intracellular carboxy-terminus. Like with other GPCRs, the agonist-induced activation and phosphorylation of eLH/CGR are important steps for its agonist-induced internalization (Byambaragchaa et al., 2021c). Most internalized receptor-hormone complexes are routed to the lysosome where the hormone and receptor are degraded (Kishi et al., 2001). The complex accumulates in endosomes and is then recycled back to the cell surface where it is bound (Krishnamurthy et al., 2003). The recycling or degradation of the internalized gonadotropin receptors results in the maintenance or loss of cell surface receptors, respectively (Galet et al., 2003, 2004; Hirakawa & Ascoli, 2003). This receptor recycling promotes the maintenance of cell surface receptors and preserves hormonal responsiveness (Bhaskaran & Ascoli, 2005).
The receptors of glycoprotein hormones have two specific pathways: the protein kinase A pathway, which produces cAMP via adenylate cyclase, and the protein kinase C pathway, with the Ca2+ pathway by phospholipase C. This study aimed to delineate the roles of glycosylation sites in the LH-like activity of eCG using cells expressing eLH/CGR. To better define the involvement in signal transduction of rec-eCGβ/α-stimulated cAMP synthesis, we constructed mammalian expression vectors of eCG β/α wild-type, mutant (eCGβ/αΔ56), the glycosylation sites at Asn56 of the eCG α-subunit, mutant (eCGβ-D/α) deleted O-glycosylation sites of the eCG β-subunit, and double mutant (eCGβ-D/αΔ56).
The biological activity of these proteins was investigated by signal transduction in cells expressing eLH/CGR in vitro. The findings of this study revealed the role of glycosylation sites in the LH-like activity of eCG.
MATERIALS AND METHODS
The pcDNA3 mammalian expressing vector, Chinese hamster ovary suspension (CHO-S) cells, FreeStyle MAX reagent, and FreeStyle CHO expression medium, were purchased from Invitrogen (San Diego, CA, USA). CHO-K1 cells were obtained from the Korean Cell Bank (KCB) (Seoul, Korea). The polymerase chain reaction (PCR) reagents, restriction enzymes, and DNA ligation kits were obtained from Takara (Shiga, Japan). Ham’s F-12 medium, Opti-MEM I, serum-free CHO-S-SFM II, and Lipofectamine 2000 were purchased from Gibco BRL (Grand Island, NY, USA). Fetal bovine serum (FBS) was obtained from Hyclone Laboratories (Logan, UT, USA). Disposable spinner flasks and glass flasks were obtained from Corning (Corning, NY, USA). The cyclic adenosine monophosphate (cAMP) Dynamic 2 immunoassay kit was purchased from Cisbio Bioassay (Codolet, France). PSMG enzyme-linked immunosorbent assay (ELISA) kit was purchased from DRG International (Mountainside, NJ, USA). The QIAprep-Spin plasmid kit was purchased from Qiagen (Hilden, Germany). The oligonucleotides were synthesized by Genotech (Daejeon, Korea). All other reagents were obtained from Sigma-Aldrich (St. Louis, MO, USA).
To construct eCG mutants, the cDNA encoding the full-length eCG β-subunit was fused with the mature protein portion of the α-subunit using overlapping PCR mutagenesis, as previously reported (Min et al., 2000; Lee et al., 2021). To determine the importance of oligosaccharides in the LH-like activity of eCG, site-directed mutagenesis was performed to change the sequence of the glycosylation sites. The glycosylation sites of the eCG α-subunit and β-subunit are shown in Fig. 1. After each fragment of the eCG α-subunit and β-subunit was amplified, PCR was performed to construct the single-chain eCGβ/α. The same method was used to add a myc-tag between the first and second amino acids of the mature protein in the β-subunit. A schematic diagram of the single-chain eCGβ/α/mutants is shown in Fig. 1.
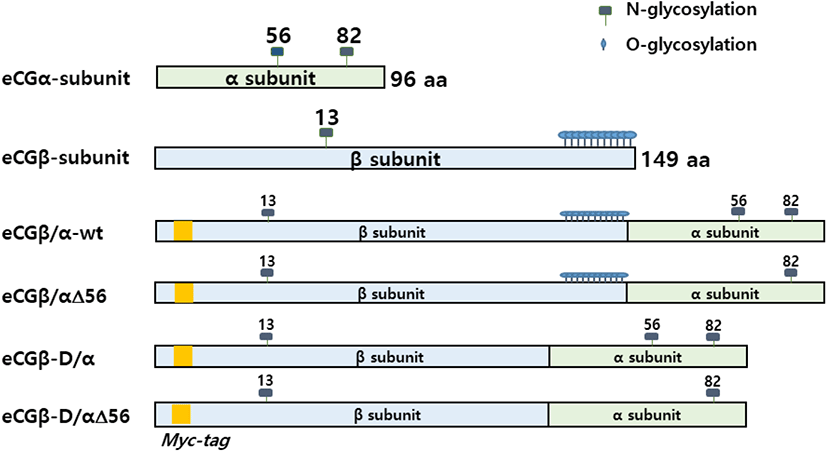
The rec-eCGβ/α proteins were expressed by transfecting the vectors into CHO-S cells using the FreeSytle MAX reagent, following the supplier’s instructions. Briefly, CHO-S cells were cultured with FreeStyle CHO expression medium at 1×107 cells/30–50 mL for 3 days. The cells were passaged at 5–6×105 cells/mL with 50–125 mL CHO expression medium in disposable spinner flasks before one day of transfection. For transfection, the cell density was approximately 1.2–1.5×106 cells/mL. DNA (1.2 mL of OptiPRO serum-free medium) and transfection MAX reagent (1.2 mL of OptiPRO serum-free medium) were mixed and incubated for 5–10 min at room temperature and then added to each cell suspension flask. For rec-protein production, the culture media were collected on day 7 after transfection and centrifuged at 100,000×g at 4°C for 10 min. The samples were then concentrated by freeze-drying. Rec-proteins were analyzed using enzyme-linked immunosorbent.
The rec-eCG proteins were quantified by PMSG ELISA with anti-PMSG antibody and horseradish peroxidase (HRP)-conjugated secondary antibody, and 3,3’,5,5’-tetramethylbenzidine (TMB) substrate as previously reported (Min et al., 2019). After freeze-drying, the concentrated sample was diluted 10 times with phosphate buffer saline (PBS). The samples were added to the wells of 96-well microplates coated with specific antibody eCG molecules. Then, the plate was incubated for 1 h and washed three times with distilled water, followed by incubation with 100 μL of HRP-conjugated secondary antibody for 1 h. Next, the plate was washed five times with 300 μL of distilled water and incubated with TMB (100 μL) for 30 min. Finally, 50 μL of stop solution was added and the absorbance of the reaction at 450 nm was measured using a plate reader within 30 min. I IU was assumed to be 100 ng, according to the conversion factor of the suggested assay protocol.
eLH/CGR cDNA was cloned using cDNA from equine testes and ovaries, as previously reported (Lee et al., 2017). The amplified PCR fragments were cut by the EcoRI and XhoI enzymes, and then the purified samples were ligated into the pcDNA3 mammalian expression vector by the EcoRI and XhoI enzyme sites (designated as pcDNA3-eLH/CGR). The orientation of the insert was confirmed using restriction mapping. The identity of this construct was verified by sequencing the entire open reading frame. Transfection of CHO-K1 cells was performed using the liposome transfection method as previously described (Lee et al., 2021). CHO K1 cells were cultured in growth medium (Ham’s F-12 media containing penicillin (50 U/mL), streptomycin (50 μg/mL), glutamine (2 mM), and 10% FBS). 80%–90% of CHO K1 cells were transfected in 6 well plates. The DNA and lipofectamine reagent were combined and incubated for approximately 20 min. The cells were washed with Opti-MEM and the complexes were added to the cells. CHO growth medium (20% FBS) was added to each well after 5 h. The next day, the culture medium was changed by CHO growth medium. The transfected cells were adjusted for cAMP analysis 48–72 h after transfection.
AMP accumulation in CHO cells was measured using cAMP Dynamics 2 competitive immunoassay kits (Cisbio Bioassays) as described previously (Byambaragchaa et al., 2021c). Briefly, the cAMP assay used a cryptate-conjugated anti-cAMP monoclonal antibody and d2-labeled cAMP. Standard samples were prepared to cover an average range of 0.17–712 nM (final concentration of cAMP per well). At 48 h post-transfection, the cells (10,000 cells per well) were seeded into 384-well plates. To prevent cAMP degradation, MIX was added to the cell dilution buffer. Next, 5 μL of the compounds were added to each well and the plate was incubated for 30 min to stimulate the cells. Then, cAMP-d2 (5 μL) and anti-cAMP-cryptate (5 μL) were added to each well. The wells were then sealed and the plates were incubated for 1 h. cAMP was detected by measuring the decrease in HTRF energy transfer (665/620 nm) using a TriStar2 S LB942 microplate reader (BERTHOLD Tech., Wildbad, Germany). The results were calculated from the 665/620 nm ratio and expressed as Delta F % (cAMP inhibition), according to the following equation: [Delta F% = (Standard or sample ratio – Sample negative) × 100 / ratio negative]. The cAMP concentration for Delta F% values was calculated using GraphPad Prism software (version 6.0; GraphPad, La Jolla, CA, USA).
The Multalin multiple sequence alignment software was used for sequence analysis. GraphPad Prism 6.0 (San Diego, CA, USA) was used for analyzing cAMP responsiveness, EC50 values, and the stimulation curve. Dose-response curves were fitted with a nonlinear regression. Curves fitted in single experiments were normalized to the background signal measured for mock-transfected cells (0%). One-way analysis of variance was used to compare the results between samples. A p-value of <0.05 indicated a significant difference between groups.
RESULTS
Site-directed mutagenesis was performed to examine the biological activity of the glycosylation sites in cells expressing eLH/CGR. eCG consists of an α-subunit and a β-subunit, displaying two non-covalently linked subunits, as shown in Fig. 1. The recognition signal sites for N-linked glycosylation of proteins are the tripeptide sequence Asn-Xaa-Thr/Ser. As shown in Fig. 1, the eCG α-subunit contains two N-linked glycosylation sites at the amino acid residues Asn56 and Asn82 while the β-subunit contains one N-linked glycosylation site at Asn13 and has approximately 11 O-linked glycosylation sites in the CTP region. In the eCGβ/α wild-type, a cDNA encoding full-length eCG β-subunit (20 amino acid residue signal sequence and 149 amino residues of the mature protein) was fused with the 5’-end of eCG α-subunit cDNA lacking the signal sequence, as previously reported (Lee et al., 2021). In the subunit mutants, we mutated the specific glycosylation sites (Asn56 N-linked glycosylation site in the α-subunit and CTP-region O-glycosylation sites in the β-subunit) to demonstrate that the glycosylation sites play a pivotal role in the biological activities of cells expressing other mammalian LHR and FSHR genes (Min et al., 2019, 2020; Lee et al., 2021).
We constructed three mutants using the same method: the first mutant was constructed by substitution the Asn (AAC) of α-subunit56 at the glycosylation site with Gln (CAG); the second mutant was constructed by deleting the C-terminal region the β-subunit (CTP: 115-149aa), comprising approximately 11 O-linked glycosylation sites; and the third was constructed by deleting eCGβ-D/α and eCGβ-D/αΔ56 mutants in the CTP region of the eCG β-subunit. Thus, these two mutants showed lower DNA bands than those of eCGβ/α and eCGβ/αΔ56, as shown in Fig. 2.
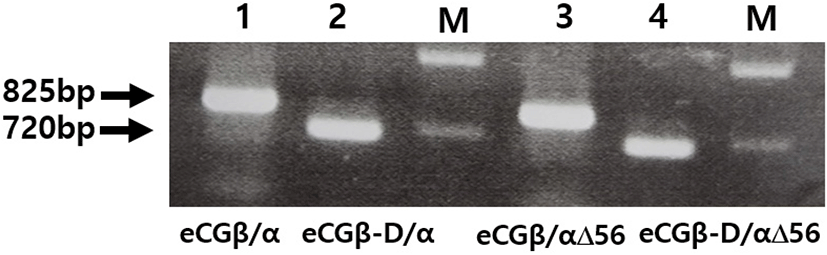
After a single chain eCGβ/α was constructed, the full-length PCR products were cloned into a pGEM-T easy vector. These PCR fragments were digested with EcoRI and SalI. The samples were then ligated into the EcoRI and XhoI sites of the pcDNA3 expression vector (designated as pcDNA3-eCGβ/α). This vector was sequenced completely to confirm the Kozak site and the absence of PCR-induced errors. Finally, four expression vectors were constructed (designated as pcDNA3-eCGβ/α, pcDNA3-eCGβ/αΔ56, pcDNA3-eCGβ-D/α, and pcDNA3-eCGβ-D/αΔ56) as previously reported (Min et al., 2019; Lee et al., 2021).
The protein level of rec-eCGβ/α wild-type was higher in the culture media of CHO-S cells in CHO-K 1 cells (Min et al., 2019; Lee et al., 2021). Although CTP-region expression of deleted mutants was a little increased and delayed, the mutants were normally expressed compared to eCGβ/α wild-type.
The effect of rec-eCGβ/α on cAMP stimulation was determined to evaluate its activity in CHO-K1 cells expressing eLH/CGR. Receptor cells were incubated with dose-dependent concentrations (0.008-1,500 ng/mL) of wild-type rec-eCGβ/α. Here, cAMP responsiveness, expressed as Delta F%, was inhibited by ligand treatment (Fig. 3). Then, we calculated the Delta F% values to express non-linear regression. These data were then calculated by cAMP concentration (nM), as shown in Fig. 4A.
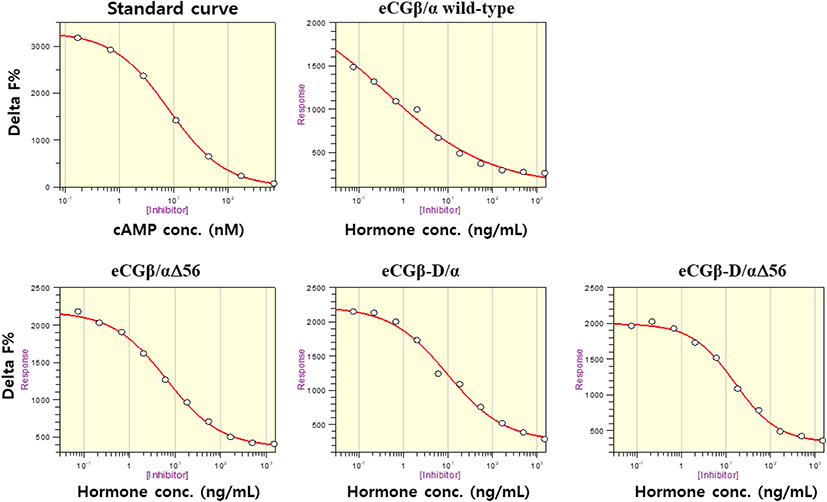
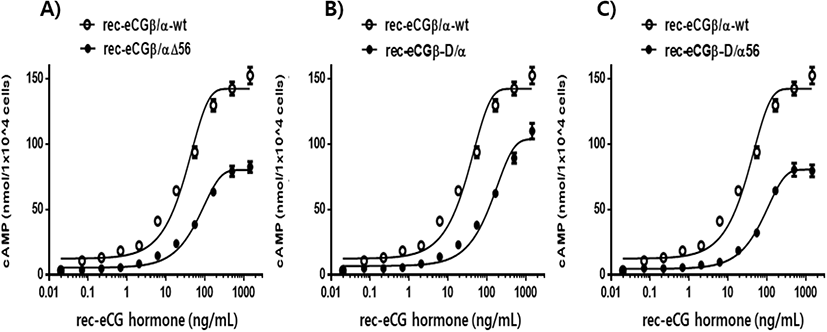
cAMP concentration increased in a dose-dependent manner relative to the concentration of the rec-eCGβ/α wild-type. The basal and Rmax cAMP responses in the eCGβ/α wild-type were 9.5±1.4 and 141.9±3.0 nM/104 cells, respectively. The half maximal effective concentration (EC50) value of the eCG-stimulated cAMP response was approximately 31.6 ng/mL (Table 1). This indicates that the rec-eCG β/α wild-type exhibited potent LH-like activity in cells expressing eLH/CGR. The dose-response curves of the three mutants for eliciting cAMP response through eLH/CGR shifted considerably to the right (Fig. 4A–C). In the basal cAMP response, three mutants (β/αΔ56, β-D/α, and β-D/αΔ56) were not affected, at 5.4±0.7, 6.5±1.1, and 5.1±1.0 nM/104 cells, respectively. However, the Rmax levels of β/αΔ56 and β-D/αΔ56 were 80.2±1.3 and 92.9±2.2 nM/104 cells, respectively, demonstrating that their levels did not increase with the high concentration treatment of agonists (Fig. 5). Rmax level was approximately 0.56-fold and 0.65-fold higher than that of the eCG β/α wild-type. However, the β-D/α mutant, deleted only in the CTP-region containing O-glycosylation sites in the eCG β-subunit, displayed 114.3±2.9 nM/104 cells, indicating that its level was approximately 80% that of eCGβ/α wild-type.
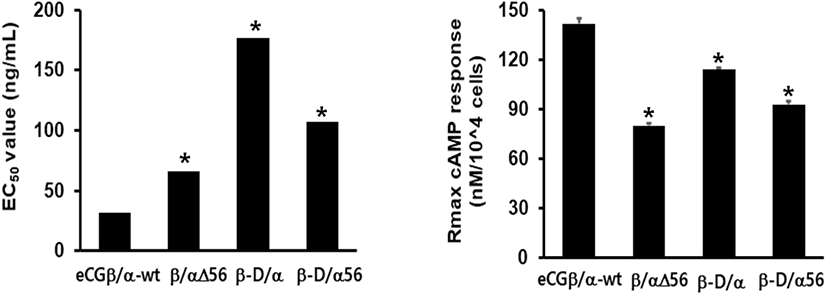
The EC50 values of rec-eCGβ/αΔ56, eCGβ-D/α, and eCGβ-D/αΔ56 were approximately 65.8, 176.8, and 106.9 ng/mL, respectively (Table 1). The EC50 value of the eCG β-subunit following C-terminal deletion decreased 5.6-fold compared to that of the eCGβ/α wild-type. This indicated that the O-linked glycosylation sites of the eCG β-subunit were critical for LH-like activity in cells expressing eLH/CGR. Thus, it can be determined that truncation of the C-terminal O-linked glycosylation sites plays a pivotal role in cAMP responsiveness (EC50 value) and maximal response in CHO-K1 cells. The EC50 value of β/αΔ56, mutated at the Asn56 of eCG α-subunit, also decreased 2.1-fold compared to that of eCGβ/α wild-type while the EC50 value of the double mutant, mutated Asn56 of the eCG α-subunit and O-linked glycosylation sites of the eCG β-subunit, was 3.4-fold lower than that of the eCGβ/α wild-type. Thus, the glycosylation sites at Asn56 of the α-subunit and the CTP of the β-subunit co-operatively promote LH-like activity in cells expressing eLH/CGR. The LH-like activity of rec-eCG mutants was markedly downregulated, which demonstrated that the glycosylation sites at Asn56 of the eCG α-subunit and CTP-region of the eCG β-subunit were essential for the LH-like activity of eCG in cells expressing eLH/CGR. Thus, these glycosylation sites are indispensable for the LH-like activity of eCG.
Values are the means±SEM of triplicate experiments. The half maximal effective concentration (EC50) values were determined from the concentration-response curves from in vitro bioassays. The cAMP responses of the basal and Rmax in rec-eCGβ/ α-wild type were shown as 1-fold.
DISCUSSION
We examined the biological roles of N-linked oligosaccharides at Asn56 of the α-subunit and O-linked glycosylation sites in the CTP-region of the eCG β-subunit. The experiments were designed as an initial attempt to identify the function of the glycosylation sites and their signal transduction for biological activity from cAMP responsiveness in cells expressing eLH/CGR.
Studies on rec-human CG (rec-hCG) suggest that the Asn52 mutant of the α-subunit (equivalent to Asn56 in the eCG α-subunit) does not affect secretion into the supernatant by transfection (Matzuk & Biome, 1988a,b). The Asn78 mutant (equivalent to Asn82 in the eCG α-subunit), however, has reported remarkably decrease in secretion. We also recently reported that the glycosylation sites of Asn82 at the eCG α-subunit and Asn13 at the eCG β-subunit play pivotal roles in from CHO-K1 cells secretion into the culture medium (Min et al., 2019). We found that the CTP-region, including O-linked glycosylation sites of the eCG β-subunit and hCG β-subunit, was not essential for secretion, in agreement with previous studies (Min et al., 2004, 2019; Lee et al., 2021). Thus, we suggest that the glycosylation sites were independent of secretion into the culture medium in the transfected cells.
Regarding biological activity in vitro, studies have reported that Asn52 of the hFSH α-subunit decreased the activity of the wild-type to 26% (Bishop et al., 1994), but the enhanced receptor binding 2- to 3-fold (Valove et al., 1994). In hCG, the Asn52 glycosylation site has been reported to be very important for signal transduction, demonstrating that the mutants at Asn52 of the hCG α-subunit were completely impaired in the cAMP response and steroid formation (Matzuk et al., 1989). Recently, we reported that Asn56 and Asn79 of the eel LH α-subunit and Asn13 of the eel LH β-subunit did not stimulate the cAMP response (Byambaragchaa et al., 2018). We found that oligosaccharide site deletions at positions Asn56 and Asn79 of the α-subunit and Asn5 on the β-subunit played an essential role in cAMP signal transduction through the eel FSH receptor. The EC50 values of Asn56 and Asn5 were 64% and 53%, respectively, of the wild-type (Kim et al., 2019). In a past study, we reported that the glycosylation sites on the eCG molecule are essential for signal transduction in the cells expressing rLHR, rFSHR, rat granulosa cells, and rat Leydig cells (Min et al., 2004, Min et al., 2019; Lee et al., 2021). Recent studies have shown that the glycosylation site at Asn56 of the eCG α-subunit plays a pivotal role in the LH-like activity of eCG in cells expressing rLHR, and the FSH-like activity of the same mutants was markedly downregulated in cells expressing rFSHR (Lee et al., 2021). Analysis of Asn56 of the eFSH α-subunit determined that its activity plays a major role in signal transduction in primary rat granulosa cells (Saneyoshi et al., 2001). Consistent with these observations, in our study, the Asn56 of eCG α-subunit plays an essential role in signal transduction in cells expressing eLH/CGR. Thus, the glycosylation site at Asn56 of the eCG α-subunit is vital for signal transduction in cells expressing eLH/CGR, rLHR, and rFSHR.
In studies of O-linked glycosylation sites in the CTP region, the biological activity by the removal of the eCG CTP region was only 75% that of eCGβ/α wild-type in cells expressing rat Leydig cells (Min et al., 2004). The LH-like activity of eCG β-D/α was 5.3-fold lower than that of wild-type eCG β/α in cells expressing rLH/CGR (Lee et al., 2021). In addition, the Rmax level of eCG β-D/α was remarkably decreased to 0.88-fold lower than that of eCG β/α wild-type. However, the signal transduction activity of mutants lacking the CTP region of the hCG β-subunit was unaffected (Sugahara et al., 1996). Studies have reported that the O-glycosylation sites (115–145 residues) at the hCG β-subunit are not affected by receptor binding and signal transduction in MA-10 Leydig tumor cells (Matzuk et al., 1990; Chen et al., 1991). However, the shorter deletion mutant (101–145 residues) of the hCG β-subunit was critical for signal transduction, indicating that truncation of this region markedly decreased the biological activity in MA-10 Leydig cells (Huang et al., 1993). In a previous study, we determined that single-chain eCGβ/α wild-type exhibited biological activity similar to that of native eCG in primary rat Leydig cells and rat granulosa cells (Min et al., 1996, 2004) and PathHunter parental cells expressing eLH/CGR (Lee et al., 2017). The dose-response curve of the double mutant (eCGβ-D/αΔ56) markedly shifted to the right in cells expressing rLH/CGR (Lee et al., 2021). In this study, the double mutant showed remarkably decreased signal transduction in cells expressing eLH/CGR. However, the same mutants exhibited similar effects on the ovulation rates of oocytes with non-functional oocytes, accounting for only 2% non-functional oocytes (Min et al., 2019).
We suggest that the O-linked glycosylation sites of the CTP region (114–149) of the eCG β-subunit is not more essential for signal transduction than the N-linked glycosylation site at Asn56 of the eCG α-subunit. However, the activity was slightly different according to the primary culture cells, CHO-K1 and PathHunter cells expressing β-arrestin 2. Further studies are required to elucidate the functional mechanisms that regulate the roles of glycosylation sites in the signal transduction of eCG molecules through eLH/CGR.