INTRODUCTION
Various cellular stresses induced by physical stimuli during somatic cell nuclear transfer (SCNT) process adversely affect the reprogramming of SCNT embryos (Morgan et al., 2005; Lee et al., 2018). The main cellular stresses include reactive oxygen species (ROS) and endoplasmic reticulum (ER) stress (Hwang et al., 2012; Lee et al., 2018; Park et al., 2020). ER and oxidative stresses promote each other, which disturbs cell function and activates pro-apoptotic signals (Malhotra & Kaufman, 2007).
ER is responsible for protein synthesis and activation in the intracellular organelle. The accumulation of unfolded or misfolded proteins in the ER lumen can disorder ER homeostasis and cause ER stress (Boyce & Yuan, 2006; Malhotra & Kaufman, 2007). When ER stress occurs, unfolded protein response (UPR), which disrupts protein synthesis, decomposes unfolded proteins, and increases the expression of chaperones that cause the unfolded proteins to fold properly, reduces ER stress and restores normal function of the cells (Malhotra & Kaufman, 2007). However, if the ER stress persists in a long time or is serious, pro-apoptotic gene is activated, leading to apoptosis. It has been reported that another cellular stress, oxidative stress, is also associated with ER stress (Cullinan & Diehl, 2006). Under ER stress condition, formation and breakage of disulfide binding accumulate the ROS and cause oxidative stress (Sevier et al., 2001; Cullinan & Diehl, 2006; Zhang et al., 2015).
Tauroursodeoxycholic acid (TUDCA), an ER stress inhibitor, has been found to reduce intracellular oxidative stress and inhibit cell apoptosis in various diseases (Xie et al., 2002). TUDCA is also reported to reduce cell apoptosis by counteracting ER stress and improve embryonic development in pig (Kim et al., 2012). TUDCA activates an activating transcription factor 6 (ATF6) to improve the folding capacity of misfolded/unfolded protein (Omura et al., 2013).
In general, ER stress inhibitor was treated during in vitro culture period of IVF embryos (Yoon et al., 2014), parthenogenetic embryos (Zhang et al., 2012), or SCNT embryos (Song et al., 2014; Lin et al., 2016) to improve the development of embryos. On the other hand, the development and quality of SCNT embryos were improved by treating an ER stress inhibitor during micromanipulation process of SCNT, by inhibiting ER stress generation and reducing apoptosis (Park et al., 2019; Park et al., 2020).
It is important to determine the appropriate treatment timing of ER stress inhibitor for the improved reprogramming of SCNT embryos. Thus, in this study, it was examined the effect of an ER stress inhibitor TUDCA treatment at various stage of the porcine SCNT procedure, such as micromanipulation, in vitro culture or both micromanipulation and in vitro culture periods.
MATERIALS AND METHODS
All chemicals and reagents were purchased from the Sigma-Aldrich (St. Louis, MO, USA) unless otherwise indicated. TUDCA (Merck, Darmstadt, Germany) melted in dimethyl sulfoxide (Junsei Chemical, Tokyo, Japan) and stored at −20℃ until use.
Porcine cumulus-oocyte complexes (COCs) were aspirated from follicles (3–6 mm diameter) using a 10 mL syringe with an 18-gauge needle. COCs were washed in Tyrode’s lactate (TL)-Hepes medium containing 0.1% (w/v) polyvinyl alcohol (PVA), and cultured in 500 μL of in vitro maturation (IVM) medium at 39℃, 5% CO2 in air for 42–44 h. The IVM medium was Tissue Culture Medium 199 (TCM199; Gibco, Grand Island, NY, USA) supplemented with 3.05 mM D-glucose, 0.91 mM Na-pyruvate, 0.57 mM cysteine, 0.1% (w/v) PVA, 75 μg/mL penicillin G, 50 μg/mL streptomycin, 0.01 IU/mL follicle stimulating hormone (FSH), 0.01 IU/mL luteinizing hormone (LH), and 10 ng/mL epidermal growth factor (EGF).
Porcine ear fibroblasts (PEFs) were cultured in Dulbecco’s modified Eagle’s medium (DMEM; Gibco) containing 15% FBS (Gibco) and 1% (w/v) penicillin/streptomycin (P/S; Mediatech, Manassas, VA, USA) at 39℃, 5% CO2 in air until they reached at confluence to synchronize the cell cycle stage at the G1 phase. Cells were trypsinized with 0.05% (w/v) trypsin-EDTA (Gibco) and centrifuged (500×g) for 4 min at room temperature in Hepes-buffered TCM199 containing 0.14 mM penicillin G, 0.78 mM NaHCO3, 0.08 mM streptomycin and 3 mg/mL BSA (TCM-BSA). Donor cells were placed in 500 μL droplets of the same medium for use.
The cumulus cells of oocytes were removed by vortexing in PBS (Gibco) containing with 0.1% (w/v) polyvinyl pyrrolidone (PVP) and 0.1% (w/v) hyaluronidase for 3 min. The metaphase II oocytes were placed in a drop of micromanipulation medium of Hepes-buffered TCM–BSA containing 5 μg/mL cytochalasin B. Enucleation was carried out by removing the 1st polar body and a small amount of surrounding cytoplasm including chromosome mass using an injection pipette. Subsequently, one donor cell was injected into the perivitelline space of an enucleated oocyte. Reconstructed oocytes were placed in holding medium of porcine zygote medium-3 (PZM-3) for 10–30 min until fusion treatment.
Reconstructed oocytes were electrically fused and activated. They were placed between two wire electrodes 1 mm apart, overlaid with 0.3-M mannitol solution containing 0.05 mM calcium chloride, 0.1 mM magnesium sulfate and 0.5 mM Hepes (Duchefa Biochemie, Haarlem, Netherlands). Two electric pulses of 1.25 kV/cm were applied for each 30 μsec using an Electro Cell Manipulator 200 (BTX, San Diago, CA, USA). After electrofusion and activation, the reconstructed oocytes were placed in holding medium at 39℃, 5% CO2 in air and examined for fusion.
SCNT embryos were cultured in droplets of 30 μL fresh PZM-3 medium at 39℃ and 5% CO2 in air for 6 days. After in vitro culture, the blastocysts were washed in PBS supplemented with 0.3% (w/v) PVP (PBS-PVP). Subsequently, the blastocysts were lysed in 30 μL of Lysis/Binding (L/B) buffer (Dynabeads® mRNA Direct kitTM), and stored at −70℃ until analyses.
In treatment group 1 (NT+3 h group), micromanipulation was conducted in micromanipulation medium with 100 μM TUDCA (Park et al., 2019), and subsequently fused SCNT embryos were cultured in PZM-3 medium containing 100 μM TUDCA for 3 h prior to in vitro culture to ensure the inhibition of ER stress (Park et al., 2019).
In treatment group 2 (IVC group), micromanipulation was conducted in micromanipulation medium without TUDCA, then fused SCNT embryos were culture in PZM-3 medium with 100 μM TUDCA for 6 days.
In treatment group 3 (NT+IVC group), micromanipulation was conducted in micromanipulation medium with 100 μM TUDCA, then fused SCNT embryos were culture in PZM-3 medium with 100 μM TUDCA for 6 days.
Untreated SCNT embryos were used as control.
Some blastocysts of each groups were washed with PBS-PVP and stained with 20 μg/mL of Hoechst 33342 for 30 min. Stained embryos were fixed on a slide glass and dropped the Vecta-Shield (Vector Laboratories, Burlingame, CA, USA). Then, the slide glass was covered with a cover slip and the cell number of embryos was counted using a fluorescence microscopy (BX50, Olympus, Tokyo, Japan).
Poly(A) mRNA of the SCNT blastocysts was isolated using a Dyna-beads® mRNA Direct™ kit according to the manufacturer’s protocol. The cryopreserved embryo samples were melted and mixed with 40 μL of Dynabeads oligo(dT)25 and shaken for 8 min at room temperature to induce hybridization of poly(A) mRNA tails with the oligo(dT)25 on the beads. The beads with attached mRNA were washed twice with each 100 μL of washing buffer A and B. Beads were separated from the supernatant using a DynaMag™-Spin Magnet (Invitrogen, Carlsbad, CA, USA). Elution of the poly(A) mRNA from the beads was carried out by incubation with 12.5 μL of 10 mM Tris-HCl (elution buffer) at 75℃ for 5 min. The cDNA synthesis was carried out using AccuPower®RocketScript™ Cycle RT Premix (Bioneer, Daejeon, Korea) according to the manufacturer’s protocol. Each 10 μL of mRNA was used for a template. The reaction was conducted by Veriti® 96-well Thermo cycler (Applied Biosystems, Foster City, CA, USA) at 4℃ for 5 min, followed by 5 cycles at 37℃ for 15 sec, 50℃ for 5 min, and 98℃ for 5 min. The cDNA products were conserved at 4℃ before use.
The expression of X-box binding protein 1 (Xbp1) mRNA was detected by semi-qPCR. For semi-qPCR, cDNA samples were reverse-transcribed using the AccuPower®Taq PCR Premix (Bioneer) in accordance with the manufacturer’s protocol. PCR condition was as follows: denaturation at 95℃ for 30 sec, annealing at 60℃ for 30 sec, and extension at 72℃ for 5 min. PCR products were analyzed by UV irradiation using a Gel Doc™XR+ (Bio-rad, Berkeley, CA, USA) on 4% agarose gel (Amresco, Cleveland, OH, USA) containing 0.05% ethidium bromide (Bioneer). ER stress-associated genes such as binding protein (BiP), glucose regulated protein 94 (GRP94), activating transcription factor 4 (ATF4), and C/ EBP homologous protein (CHOP), apoptotic genes such as Bcl2-associated X protein (Bax) and caspase-3, and oxidative stress genes such as nuclear factor (erythroid-derived 2) 2 (Nrf2) and hemeoxygenase-1 (HO-1) were analyzed by RT-qPCR. RT-qPCR was carried out using 1 μL of cDNA, 2 μL of each primer pair, 3 μL of sterile water (RNase free), and 6 μL of 2X SYBR Green PCR master mix (TOPreal™qPCR 2X PreMIX; SYBR Green with high ROX, Enzynomics, Daejeon, Korea) in a StepOne Plus (Applied Biosystems). The amplification reaction was as follows: initial-denaturation at 95℃ for 15 min, followed by 40 amplification cycles of denaturation at 95℃ for 15 sec, annealing at 60℃ for 30 sec and extraction at 72℃ for 30 sec. The ∆∆CT method (comparative CT method) was used to measure the relative mRNA quantification for respective target gene. Glyceraldehyde 3-phosphate dehydrogenase (GAPDH) was used as a housekeeping gene. The primer sequences for each gene used are listed in Table 1.
Statistical analysis was carried out using the Statistical Analysis System software (v.9.4, SAS Institute, Cary, NC, USA). PCR data were analyzed using the general linear model (GLM) and the Duncan’s multiple range test. Blastocyst formation rate was analyzed by Chi-square test, and the average cell number in blastocysts was analyzed by Student t-test.
RESULTS
Xbp1 splicing tended to decrease in treatment groups (NT+3 h, IVC, and NT+IVC), but there was no significant difference from control group (23.4%), and among treatment groups (14.5%–15.3%, Fig. 1).
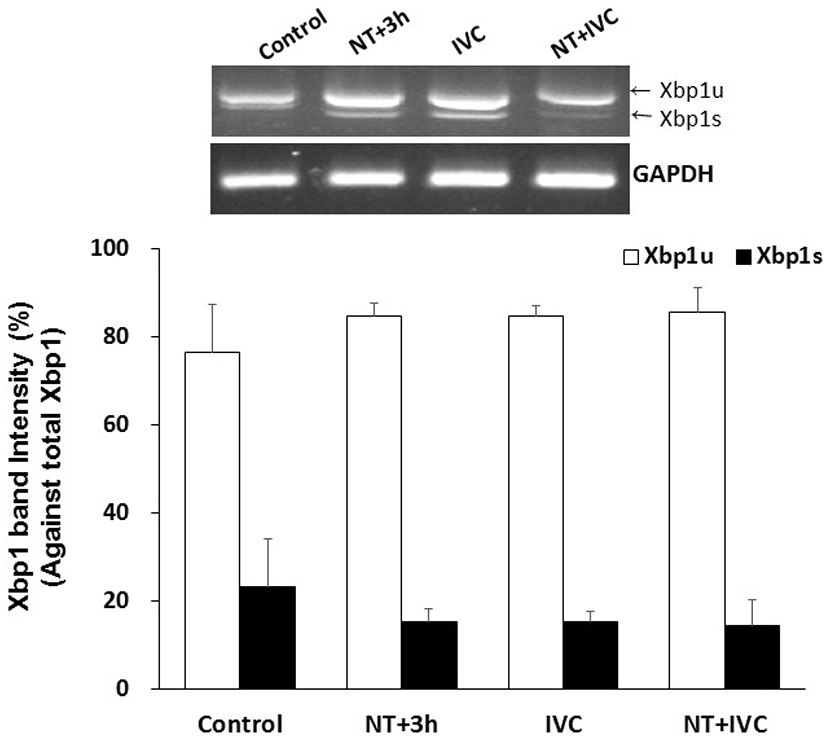
In the expression of ER stress-associated genes, the relative transcription levels of CHOP in NT+3 h group and ATF4 and CHOP in IVC group were significant lower than those of control (p<0.05, Fig. 2). In the NT+IVC group, the relative expression levels of all genes (BiP, GRP94, ATF4, and CHOP) were significantly decreased compared to those of control (p<0.05).
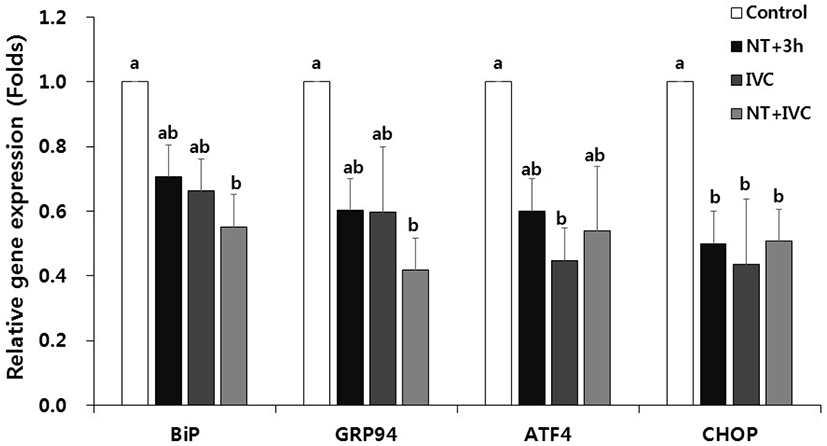
In all treatment groups with ER stress inhibitor, the relative expression levels of oxidative stress-related genes Nrf2 and HO-1, were significantly reduced compared to the control (p<0.05, Fig. 3).
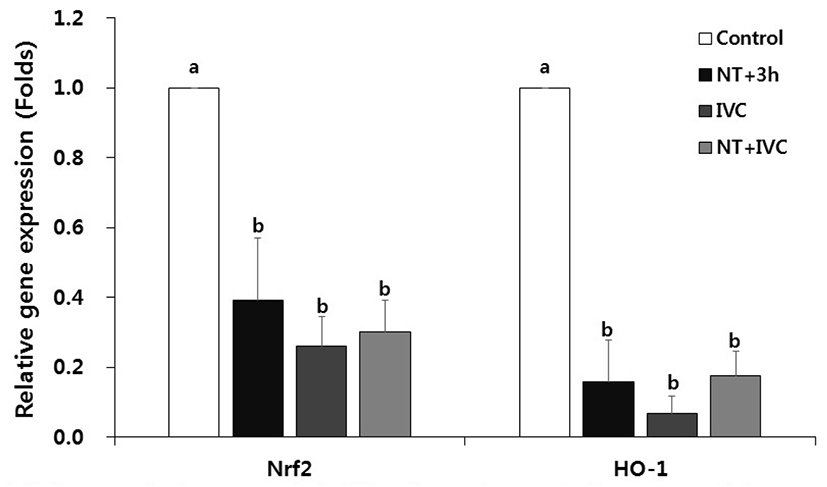
The relative expression levels of pro-apoptotic genes, Bax and caspase3 mRNAs, were significantly decreased in all TUDCA treatment groups compared to the control (p<0.05, Fig. 4).
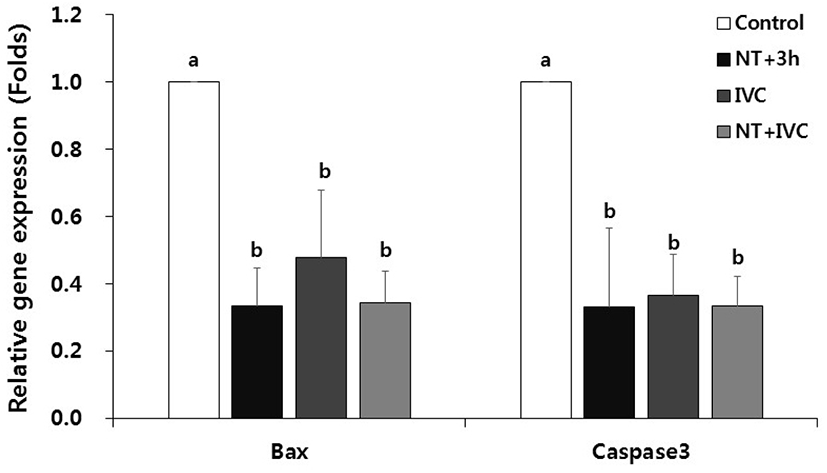
Developmental rate to the blastocyst stage tended to increase in all treatment groups, but there was no difference between the control and treatment groups and among treatment groups (17.5% to 21.7%, Table 2).
Experiment was repeated more than 12 times in each treatment group.
The mean cell number in blastocysts was significantly increased in NT+3 h (48.5±2.3) group and NT+IVC group (47.7±2.4), but not IVC group (36.5±2.4) compared to the control (35.3±2.5, p<0.05, Table 2).
DISCUSSION
When ER stress occurs, the inactivated BiP is separated from the inositol-requiring enzyme 1 (IRE1), PKR-like ER kinase (PERK) and ATF6 by the accumulation of unfolded and misfolded proteins in the ER lumen and UPR is activated (Yoshida, 2007). Activated IRE1 α leads the splicing of Xbp1 mRNA (Schröder & Kaufman, 2005; Hetz & Glimcher, 2009). PERK-mediated phosphorylation of the α-subunit of eukaryotic translation initiation factor 2 (elf2 α) induces the activation of ATF4, which stimulates the induction of CHOP (Harding et al., 2000). Activated ATF6 regulates the transcriptions of GRP78, GRP94, XBP1, and CHOP (Yoshida et al., 2001).
Only spliced Xbp1 (Xbp1s) can enter the nucleus and control UPR-related genes. Xbp1s is reported to affect the maturation of oocytes and the activation of early embryonic genomes by suppressing the generation of ER stress (Zhang et al., 2012). In this study, the Xbp1 splicing following TUDCA treatment time tend to decrease in all treatment groups, but there was no difference from the control. It can be assumed that there was no difference in expression levels when Xbp1 was measured at the blastocyst stage, because some mechanism affecting the activation of the early embryonic genome occurred during the early cleavage stage.
In this study, ER stress-associated genes (BiP, GRP94, ATF4, and CHOP) were differently expressed depending on the treatment time of TUDCA. In the NT+3 h group, CHOP expression was lower than that of the control, while in NT+IVC group the expression of all genes except ATF4 was lower than that of the control. According to the result of this study, as the treatment period increased, the number of ER stress-associated genes with significantly lower expression compared to control increased. Zhang et al. (2012) and Yoon et al. (2014) reported that the ER stress-related gene expression in the blastocyst stage was reduced when TUDCA was treated for culture period of porcine or bovine embryos. However, in other previous studies, when analyzing the ER stress-associated gene during the blastocyst stage, no significant difference was found between the ER stress inhibitor treatment group and control group (Park et al., 2019; Park et al., 2020). Meanwhile, in the previous study, the expression of Xbp1 and ER stress-associated gene at the 1-cell stage was significantly decreased in the TUDCA treatment group (Park et al., 2019; Park et al., 2020).
Nrf2, a higher factor in redox enzyme, is a typical transcription factor that responds to oxidative stress in cells (Cullinan & Diehl, 2004). When ER stress occurs, ROS is generated while forming disulfide bonds in the folding of the protein, which results in oxidative stress and Nrf2 increases the expression of HO-1 through the Nrf2 pathway (Cullinan & Diehl, 2006; Nguyen et al., 2009; Saito, 2013). In this study, the expression of oxidative stress-related genes decreased in all TUDCA treatment groups, which is similar to the previous result (Park et al., 2020). In the previous study, it was confirmed that the expression of Nrf2 and HO-1 was reduced in the 1-cell stage of SCNT embryos by TUDCA treatment during porcine SCNT process, and the expression of HO-1 was also decreased in the blastocyst stage (Park et al., 2020).
In severe ER stress, CHOP induced by the apoptotic pathway leads apoptosis by translocating the pro-apoptotic gene, Bax, from the cytoplasm to the mitochondria (Oyadomari & Mori, 2004; Sano & Reed, 2013). In addition, caspase12, which is a representative pro-apoptotic cysteine protease, activates caspase3 in the downstream, leading to apoptosis (Oyadomari & Mori, 2004). TUDCA reduces the translation of Bax into mitochondria membrane and inhibits the activation of caspase12 by preventing the release of cytochrome c (Xie et al., 2002). In this study, the expression of apoptotic gene in all treatment groups was significantly lower than that of control. In particular, Bax mRNA level was relatively lower in NT+3 h and NT+IVC groups that treated with TUDCA during the micromanipulation process of SCNT than IVC group. The decreased expression level of CHOP indicates that apoptosis induced by ER stress is inhibited. This is consistent with Park et al. (2020) reported that the expression of most ER stress-associated genes were reduced not only in the 1-cell stage but also in the blastocyst stage as a result of TUDCA treatment during the micromanipulation process.
In this study, although the expression of genes related to ER stress, ROS and apoptosis showed a tendency to decrease in all TUDCA treatment groups, the blastocyst development rate of SCNT embryos was not improved. The expression of genes in the blastocyst stage does not seem to have a direct effect on the development of SCNT embryos. However, the mean cell number in blastocysts in the NT+3 h and NT+IVC groups significantly increased compared to the control and IVC group. These results suggest that at least the quality of SCNT embryos can be improved by inhibiting or immediately reducing the ER stress during the initial micromanipulation process. These results are consistent with previous reports that ER stress inhibiter treatment during micromanipulation process of porcine SCNT can increase the blastocyst cell number rather than blastocyst development rate (Park et al., 2019; Park et al., 2020). On the other hand, in previous parthenogenesis and IVF studies, it was reported that the blastocyst development rate and cell number increased when TUDCA was treated during the IVC period (Kim et al., 2012). The reason for this difference is unclear, but in this study, the considerable physical stimulation of the SCNT process may have made this difference.
In conclusion, the result of this study suggests that treatment of ER stress inhibitor from the micromanipulation process of SCNT can improve the reprogramming efficiency of porcine SCNT embryos by inhibiting the ER and oxidative stresses that may occur early in the SCNT process.