INTRODUCTION
The continuous growth and expansion in size during the lifetime is a characteristic of fat tissue, unlike other tissues having limited developmental capacity (Hausman et al., 2001). The epididymal fat is a gonadal fat belonging to interperitoneal white adipose tissue, most of the epididymal fat is present near the head of epididymis (Berry et al., 2013; Lee, 2020). Based on the location and histochemical characteristics, the epididymal fat pad is divided into distal, proximal, and tail epididymal fat (Tirard et al., 2007; Lee, 2020). The epididymal fat tissue contains several cell types, including mature adipocyte, preadipocytes, immune cell, and endothelial cell (Cleary et al., 1977). The lipid-filled adipocyte in the mouse epididymal fat begins to appear as early as 7 days of postnatal age (Han et al., 2011). In the rat, the active proliferation of adipocyte and non-adipocyte cells in the epididymal fat tissue is observed from 12 to 35 days of age, and further remarkable increase of non-adipocyte cells in the number is found from 35 to 70 days of age (Cleary et al., 1977). In addition, the number and size of lipid-filled adipocytes is also rapidly increased from 35 to 70 days of age, even though changes of cells in epididymal fat tissue remain in the steady level or is slightly occurred after 70 days of age (Cleary et al., 1977), indicating dynamical functional and morphological changes in the epididymal fat cells during postnatal period.
The development and growth of fat tissue are influenced by various factors, including age, nutrition, and hormones. Knittle & Hirsch (1968) have demonstrated that nutritional difference during early postnatal period gives an effect on long-lasting changes in cell size and number of the rat epididymal fat pad. Not only the wet weight but also a relative weight to body weight of the epididymal fat are continuously increased during postnatal period (Cleary et al., 1977). The changes of fat cell number and size by hormonal regulation have been demonstrated by a number of researches (References in Hausman et al., 2001). In addition, the effect of sex steroid hormones, testosterone and estrogen, on the development of the epididymal fat has been suggested. Indeed, the presence of a relatively high concentration of androgen receptor (AR) in the epididymal fat has been demonstrated (Dieudonne et al., 1995). Moreover, the existence of estrogen receptor (ER) in the epididymal fat has been revealed (Gray & Wade, 1980), and the expressional regulation of ERα in the epididymal fat by diet has been shown (Metz et al., 2016). Other researches have found that the effect of such hormonal factors on the proliferation and differentiation of epididymal adipocyte is likely exerted through the expression regulation of various transcription factors, including CCAAT/enhancer binding proteins, insulin-like growth factor 1, peroxisome proliferator-activated receptor gamma (Dieudonne et al., 1995; References in Hausman et al., 2001). These observations suggest that estrogen could give an influence on the development of the epididymal fat tissue by regulating the expression of adipogenesis-associated molecules.
The estrogen receptor α knockout (ER α KO) mouse has a genetically non-functional ER α and is useful to examine the in vivo function of ER α. Of various abnormal characteristics found in the ER α KO mouse, it is notable that ER α KO mouse has more white adipose tissue than wild type (WT) in both male and female (Heine et al., 2000). In male ER α KO mouse, the epididymal fat is already heavier than WT mouse at 30 days of postnatal age, and the weight difference of the epididymal fat between WT and ER α KO mice become greater with age (Heine et al., 2000). The heavier epididymal fat tissue in ER α KO mouse is likely due to increases of adipocyte size and number (Heine et al., 2000). Because the differentiation and proliferation of adipocyte are regulated by a combined action of anti- and pro-adipogenic transcription factors (Mareno-Navarrete & Fernández-Real, 2012), it is reasonable to consider that a lack of functional ER α would result in expressional de-regulation of such transcription factors-associated with the development of epididymal fat during postnatal period.
Thus, the present research has attempted to determine expression patterns of several transcription factors promoting adipogenesis in the ER α KO mouse epididymal fat at different postnatal ages. In addition, expression of AR and ER β at postnatal ages has been examined. Moreover, transcriptional expression of adipocyte function-related molecules and secretory hormones in the epididymal fat during postnatal period has been studied.
MATERIALS AND METHODS
The homozygote ER α KO male mice around 2 (n=4), 5 (n=4), 8 (n=3), and 12 (n=3) months of postnatal ages were obtained from a colony maintained in Sungshin Women’s University. The ER α KO mice were produced as described in a previous report (Gieske et al., 2008).
The euthanization of mouse was performed by over-exposure to CO2 in a chamber, and a small incision was made at lower abdominal cavity of the mouse. The male reproductive tract, including testis, epididymis, and epididymal fat, was exposed through the incision and placed in a cold phosphate buffered saline (PBS) solution. The epididymal fat pad was carefully dissected and separated out from the rest. The fat pad was quickly rinsed in fresh cold PBS solution and placed in liquid nitrogen. The tissue was stored in −80°C until used for total RNA extraction.
The frozen epididymal fat was used for total RNA isolation by a standard extraction method with Trizol reagent (Molecular Research Center, Cincinnati, OH, USA). Briefly, the epididymal fat tissue was quickly homogenized in Trizol solution, and total RNA was isolated and precipitated by a sequential supplement of chloroform and isopropanol. An amount of total RNA resuspended in DEPC-dH2O was quantitated by NanoDrop Lite spectrophotometer (Thermo Scientific, Massachusetts, MA, USA).
The generation of complementary DNA (cDNA) from total RNA was carried out by using iScripTM Reverse transcription Supermix for reverse transcription (RT)-qPCR (Bio-Rad Laboratories, Hercules, CA, USA), 1 μg of total RNA, and nuclease-free dH2O to make a final volume of 20 μL. The RT reaction was executed at 25°C for 5 min, 46°C for 20 min, and 95°C for 1 min.
A mixture of 1 μL of cDNA, 7 μL of iQTM SYBR® Green Supermix (Bio-Rad Laboratories), 10 pmol of individual primer, and nuclease-free dH2O to adjust a final volume of 25 μL was subjected for quantitative real-time PCR analysis. The oligonucleotide primers were designed by Primer 3 software (https://bioinfo.ut.ee/primer3: Kõressaar et al., 2018), and sequences and detailed information of the primers are listed in Table 1. The examined molecules were CCAAT enhancer binding protein, alpha (Cebpa), CCAAT enhancer binding protein, beta (Cebpb), CCAAT enhancer binding protein, gamma (Cebpg), sterol regulatory element binding transcription factor 1 (Srebf1), Ar, ER, alpha (Esr1), ER, beta (Esr2), peroxisome proliferator-activated receptor, gamma (Pparg), fatty acid binding protein 4 (Fabp4), delta like non-canonical Notch ligand 1 (Dlk1), fatty acid synthase (Fasn), lipoprotein lipase (Lpl), leptin (Lep), adiponectin (Adipoq), and resistin (Retn). The 18s ribosomal RNA (Rn18s) was included as a PCR internal control. The PCR was performed in the condition of a pre-denaturation step at 95°C for 5 min, cycles of a denaturation step at 95°C for 30 sec, an annealing step at Tm for 30 sec, and an extension step at 72°C for 30 sec, and an extra extension step at 72°C for 10 min. The size of PCR product was confirmed by 1.2% agarose gel electrophoresis.
PCR, polymerase chain reaction; Cebpa, CCAAT enhancer binding protein, alpha; Cebpb, CCAAT enhancer binding protein, beta; Cebpg, CCAAT enhancer binding protein, gamma; Srebf1, sterol regulatory element binding transcription factor 1; Ar, androgen receptor; Esr1, estrogen receptor, alpha; Esr2, estrogen receptor, beta; Pparg, peroxisome proliferator-activated receptor, gamma; Fabp4, fatty acid binding protein 4; Dlk1, delta like non-canonical Notch ligand 1; Fasn, fatty acid synthase; Lpl, lipoprotein lipase; Lep, leptin; Adipoq, adiponectin; Retn, resistin; Rn18s, 18s ribosomal RNA.
The transcript level of target gene at a specific postnatal age was normalized to that of Rn18s transcript level, and the relative expression ratios of target gene among different postnatal ages were calculated by 2−ΔΔCt method (Livak & Schmittgen, 2001). A mean and standard error of target gene at a given age was obtained from separately triplicated RT reactions and PCRs. The data were expressed in a relative ratio of target gene at each postnatal age against its of 2 months of age. Statistical differences of expression level of target gene among different postnatal ages were determined by one-way ANOVA, followed by Duncan’s test if a significance was present. When a probability was less than 0.05, it was considered that a statistical significance was present between those age groups.
RESULTS
Expression patterns of Cebp family are shown in Fig. 1. The level of Cebpa transcript at 5 months of age was significantly lower than that at 2 months of age, but the expression level of Cebpa at 8 months of age was not different with that at 2 months of age (Fig. 1A). The dramatic increase of Cebpa mRNA level was detected at 12 months of age (Fig. 1A).
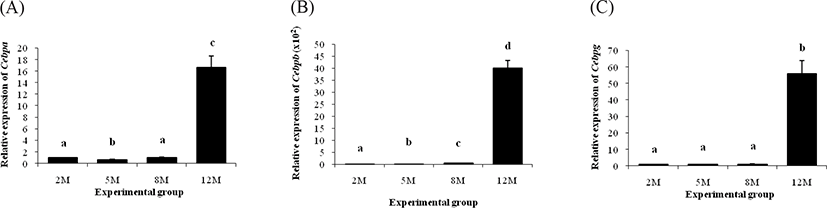
A significant increase of Cebpb transcript level was detected at 5 months of age, compared with that at 2 months of age (Fig. 1B). Expression level of Cebpb in the ER α KO epididymal fat was further increased at 8 months of age, followed by about 87 times surge of Cebpb mRNA level at 12 months of age (Fig. 1B). There was no statistical change of Cebpg transcript level in the ER α KO epididymal fat from 2 months of age to 8 months of age (Fig. 1C). However, over 50 folds increase of Cebpg was observed at 12 months of age (Fig. 1C).
A significant change of Srebp1 transcript level in the ER α KO epididymal fat was only observed at 12 months of age, but not at other ages (Fig. 2A). The level of Ar mRNA at 5 months of age was significantly decreased at 5 months of age, compared with that at 2 months of age (Fig. 2B). But, the transcript level of Ar at 8 months of age was greatly higher than those at 2 and 5 months of age (Fig. 2B). The highest level of Ar transcript was found in the ERαKO epididymal fat at 12 months of age (Fig. 2B).
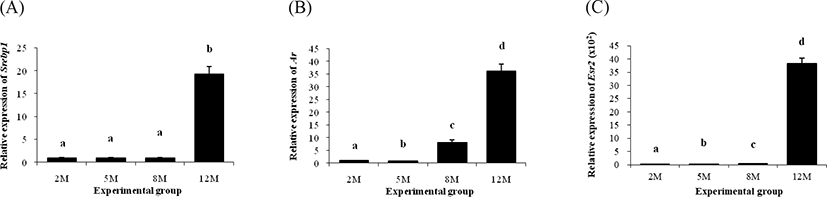
There was no Esr1 transcript detected at all experimental age groups, as expected (no data shown). A significant increase of Esr2 was observed at 5 months of age, followed by an additional rise of Esr2 transcript level in the ER α KO epididymal fat at 8 months of age (Fig. 2C). Like other molecules, the highest level of Esr2 in the ER α KO epididymal fat was detected at 12 months of age (Fig. 2C).
The mRNA level of Pparg in the ER α KO epididymal fat at 5 months of age was not significantly different with that at 2 months of age (Fig. 3A). An increase of Pparg transcript level in the ER α KO epididymal fat was found at 8 months of age, and the expression of Pparg was further increased at 12 months of age (Fig. 3A). The expression of Fabp4 in the ER α KO epididymal fat was increased at 5 months of age, followed by further surge of Fabp4 transcript level at 8 months of age (Fig. 3B). A huge rise of Fabp4 transcript level was detected at 12 months of age (Fig. 3B).
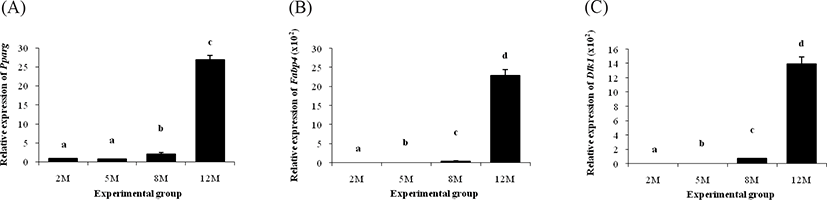
The expression pattern of Dlk1 in the ER α KO epididymal fat during postnatal period was quite similar with its of Fabp4 (Fig. 3C). The transcript level of Dlk1 at 5 months of age was significantly higher than that at 2 months of age (Fig. 3C). The amount of Dlk1 mRNA in the ER α KO epididymal fat at 8 months of age was about 50-folds greater than that at 5 months of age (Fig. 3C). Another surge of Dlk1 expression in the ERαKO epididymal fat was found at 12 months of age (Fig. 3C).
The level of Fasn transcript in the ER α KO epididymal fat at 5 months of age was significantly decreased, compared with that at 2 months of age (Fig. 4A). There was no statistical change on expression level of Fasn between 5 and 8 months of age, even though a surge of Fasn transcript level was found at 12 months of age (Fig. 4A).
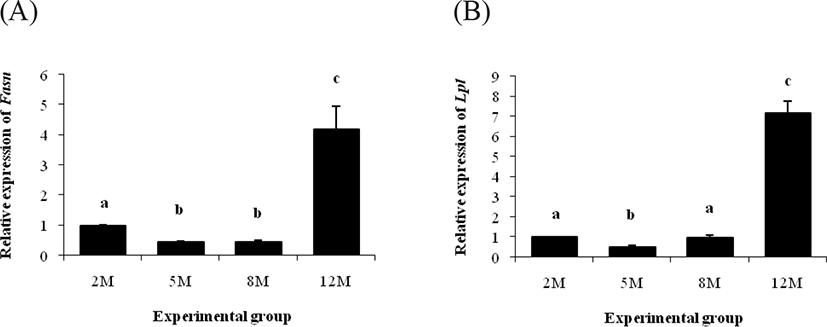
The Lpl transcript level in the ER α KO epididymal fat at 5 months of age was lower than that at 2 months of age (Fig. 4B). However, the level of Lpl mRNA at 8 months of age was returned to that at 2 months of age, followed by the highest expression level of Lpl at 12 months of age (Fig. 4B).
The transcript level of Lep was significantly increased at 5 months of age, compared with that at 2 months of age (Fig. 5A). However, the amount of Lep transcript in the ER α KO epididymal fat was not changed until 12 months of age (Fig. 5A). On the other hand, there was no significant change of the Adipoq transcript level between 2 and 5 months of age, followed by about one-fold increase of Adipoq mRNA amount at 8 months of age (Fig. 5B). A tremendous increase of Adipoq transcript level was observed in the ERαKO epididymal fat at 12 months of age (Fig. 5B).
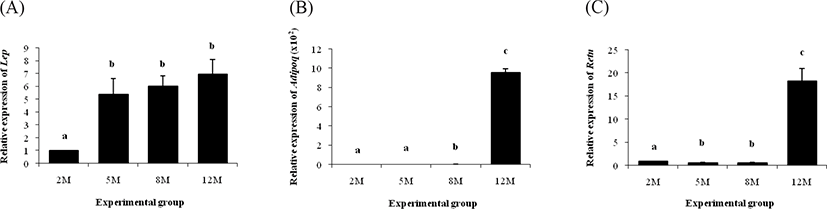
The expression pattern of Retn in the ER α KO epididymal fat during postnatal period is shown in Fig. 5C. The transcript level of Retn at 5 months of age was significantly decreased, compared with that at 2 months of age (Fig. 5C). There was no change of Retn transcript level between 5 and 8 months of age, even though a clear surge of Retn expression was detected at 12 months of age (Fig. 5C).
DISCUSSION
The expression of adipocyte development- and function-associated molecules in the ERαKO epididymal fat tissue has been determined in the present research, presumably for the first time, to our knowledge. As anticipated, the expression of Esr1 was not detected at any postnatal age, indicating the exclusion of methodological errors derived from a real-time PCR analysis. Even though expression patterns of some molecules between 2 and 8 months of ages were different with those of other molecules, the expression levels of all other molecules examined here were generally increased with age and were the highest at 12 months of age, except Lep.
The postnatal epididymal fat contains not only lipid-filled fully differentiated adipocytes but also undifferentiated preadipocytes at unneglectable amount (Cleary et al., 1977). Thus, it is likely that the growth and extension of the epididymal fat during postnatal period are associated with the increased mature adipocyte number and size, as well as proliferation of preadipocyte and differentiation rate of preadipocyte into mature adipocyte at the early postnatal age. The differentiation of preadipocyte into adipocyte requires differential expression of numerous transcription factors and/or regulatory molecules (Niemelä et al., 2008; Mareno-Navarrete & Fernández-Real, 2012). A decrease of Dlk1 expression is necessary to begin the adipogenesis and the accumulation of lipid droplets in the adipocyte (Niemelä et al., 2008; Mareno-Navarrete & Fernández-Real, 2012). Expressional increases of Cebpa and Pparg lead to activation of adipocyte-specific genes for terminal differentiation of preadipocyte, while Cebpb and Cebpg act on the early adipogenic induction of preadipocyte (Niemelä et al., 2008). Lipogenesis of adipocyte is stimulated by the action of Srebp1, and an induction of Fabp4 expression is observed during terminal differentiation of early adipocyte into fully-mature adipocyte (Niemelä et al., 2008). Even though mature adipocyte secretes soluble form of LPL, an increase of Lpl expression is found at the early stage of adipogenesis (Niemelä et al., 2008). The Lep and Adipoq, are kinds of adipokines, which are cytokines produced from adipocyte and play important functions for communication between adipocyte and target tissues, including brain, liver, immune system, and reproductive tissue (Fasshauer & Blüher, 2015). In addition, it is known that Retn is a representative cytokine secreted from adipose tissue and involves in insulin resistance and inflammation (Fasshauer & Blüher, 2015). Other than these factors, various adipogenic and antiadipogenic molecules participate in regulation of adipogenesis during the development of adipose tissue (Niemelä et al., 2008; Mareno-Navarrete & Fernández-Real, 2012). Thus, it is important to understand how expression of these molecules involved in the control of adipogenesis is regulated.
Hormonal influence on adipocyte differentiation has been examined from a number of researches. The differentiation of adipocyte is stimulated by insulin-like growth factor 1, glucocorticoids, and thyroid hormone (Niemelä et al., 2008; Mareno-Navarrete & Fernández-Real, 2012). These factors promote the adipogenesis by modulating expression of Cebpg, Pparg, and Cebpb, which are necessary for adipocyte differentiation (Niemelä et al., 2008). However, epidermal growth factor, tumor necrosis factor-α, interleukin-1, and transforming growth factor are commonly known antiadipogenic factors, which suppress adipogenesis by affecting expression of Pparg and Cebpa and inhibiting activity of Pparg (Niemelä et al., 2008; Mareno-Navarrete & Fernández-Real, 2012).
Other than peptide hormones affecting adipocyte differentiation, estrogen, a steroid hormone, has caught the attention due to its antiadipogenic and antilipogenic effects on the development of adipose tissue during postnatal period (Cooke & Naaz, 2004). As shown in the present and other researches, the epididymal fat possesses both ER α and ER β, indicating a direct effect of estrogen through functional ERs (Metz et al., 2016). In fact, a lack of functional ER α, as seen in ER α KO mouse, results in an increase of the epididymal fat mass (Heine et al., 2000). Similar findings have been observed at Cyp19 knockout (aromatase knockout, ARKO) mice, which do not produce estrogen (Jones et al., 2000). From these data, it is suggested that ERα plays an important role on differentiation and maturation of adipocyte in the epididymal fat. Our previous researches have shown expression patterns of several transcription factors involving in the regulation of adipogenesis and some secretory molecules from the epididymal fat tissue of WT mice during postnatal period (Lee, 2019; Lee & Kim, 2019). However, these findings are not directly comparable with results acquired from the current research because of different experimental materials examined, a whole epididymal fat tissue in the present study vs. a separate part of epididymal fat tissue in the earlier researches. In spite of, the highest levels of Lep, Pparg, Lpl, Fabp4, Dlk1, Retn, Adipoq, and Fasn are observed in the distal epididymal fat of WT mouse (Lee & Kim, 2019) and a whole epididymal fat of ER α KO mouse at 12 months of postnatal age, while expression patterns of these molecules, except Pparg and Dlk1, in the proximal epididymal fat of WT mouse are quite different with those in ER α KO mouse. Based on these observations, it is speculated that regional difference of Esr1 expression level within the epididymal fat tissue could influence on differential expression of adipocyte maturation and differentiation-associated factors. Regional examination of the epididymal fat of ER α KO mouse would provide more detailed information about different expressional regulation of these molecules through functional ER α.
The ER α KO mouse already has heavier epididymal fat weight than WT mouse at 30 days of postnatal age, and the degree of epididymal fat weight difference between ER α KO and WT mice becomes extended from about 1.6 times at 30 days to about 2.3 times at 270–360 days of age (Heine et al., 2000). In addition, adipocyte size and number in the epididymal fat of ER α KO mouse are bigger and larger than WT mouse at 180 days of age (Heine et al., 2000). Moreover, it is documented that estrogen through ERα exerts its effects on adipose tissue by a decrease of lipogenesis rate accompanied with a reduction of Lpl expression, an increase of lipolysis, and stimulation of adipogenesis associated with an increase of adipocyte precursor proliferation (Cooke & Naaz, 2004). A lack of estrogen action through ER α results in an increase of size and number of adipocytes at adult and developmental stage, respectively (Cooke & Naaz, 2004). Based on these observations, continuously increased expression of adipogenic factors and decreased expression of antiadipogenic factors have been expected in the ER α KO epididymal fat tissue. However, even though expression patterns of Cebpb and Fabp4 seem to follow our prospect, expression patterns of most molecules in ER α KO epididymal fat during postnatal period have not agreed with our expectation. A lack of functional ER α from the beginning in the ER α KO mouse would be a reason to explain the difference between experimental data and our speculation. Despite, the current research has been recognized for the demonstration of the existence and expression patterns of various factors-associated with adipocyte in the ER α KO epididymal fat at different postnatal ages.