INTRODUCTION
The epididymal fat is a kind of intraperitoneal white adipose tissue and is located at the head of epididymis (Fig. 1) (Niemalä, 2008; Berry et al., 2013). Like other adipose depot, the epididymal fat is composed of several cell types, including mature adipocytes in variable size, immune cells, endothelial cells, and preadipocytes (Cleary et al., 1977; Dani & Billon, 2012). The lipid-filled adipocyte in epididymal fat region of mouse appears as early as at 7 postnatal age and is originated from undifferentiated mesenchymal cells (Han et al., 2011). In vitro experiment with cells isolated from primitive epididymal fat tissue reveals that the adipogenic differentiation of epididymal progenitor cells requires cell-to-cell and cell-to-matrix interaction and occurrence of normal angiogenesis controlled by macrophage and vascular endothelial growth factor systems (Han et al., 2011).
Unlike other tissues in the body which do not grow over the size at the adult, the fat tissue is capable to continuously expend its mass afterward (Hausman et al., 2001). The increase of epididymal fat weight becomes observed after the birth (Bjorntorp et al., 1979), and the postnatal growth of epididymal fat is associated with the increase of adipocyte in size and number, depending on the stage of development (Cleary et al., 1977). While the development of epididymal fat during early postnatal period is governed by the increase of adipocyte number, the increase in size of each adipocyte contributes to the expansion of epididymal fat at the later postnatal age (Knittle & Hirsch, 1968; Johnson & Hirsch, 1972; Cleary et al., 1977). In addition, the cellular composition of epididymal fat is substantially changed during postnatal period. The highest ratio of preadipocytes in the epididymal fat is found at the early prenatal period, followed by a drastic decrease of the ratio after the puberty (Björntorp et al., 1979). These findings suggest the presence of distinct regulatory mechanisms at different development stages, in accordance with a whole postnatal growth.
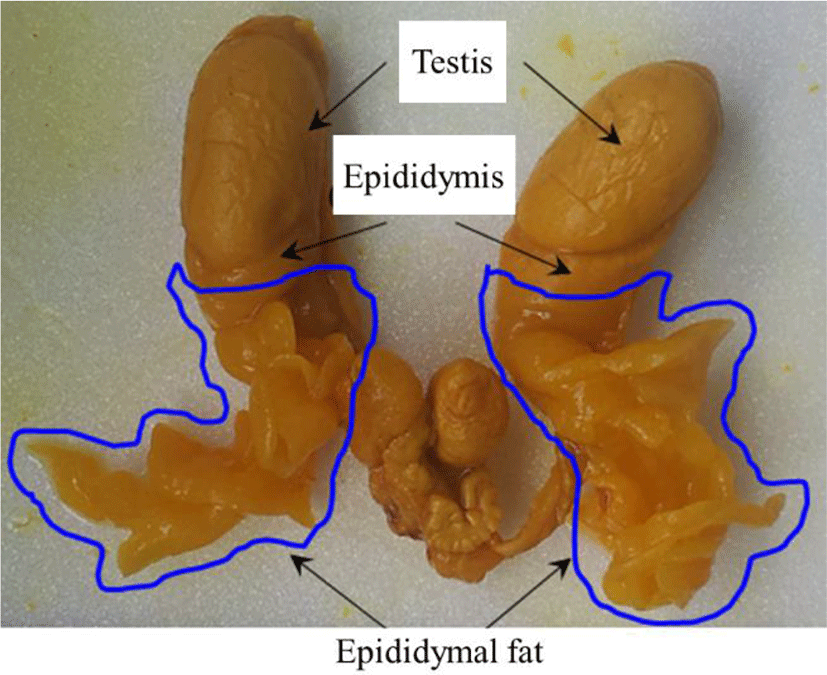
The differentiation and proliferation of preadipocyte into mature adipocyte require expression of a series of adipogenic molecules (Hausman et al., 2001; Dani & Billon, 2012). Differential expression of adipocyte-related genes in the epididymal fat during postnatal development has been examined by our and other researches (Liu et al., 2011; Lee & Kim, 2018). Even though expressional patterns of some adipocyte-associated genes in the epididymal fat of rat and mouse are generally similar, expression of other gens related with adipocyte differentiation and proliferation is different. For example, expressional level of fatty acid binding protein 4 (Fabp4) in the mouse epididymal fat is gradually increased with age (Liu et al., 2011), while transcript level of Fabp4 in the rat epididymal fat becomes decreased as aged (Lee & Kim, 2018). In contrast, expressional patterns of peroxisome proliferator-activated receptor gamma (Pparg) in the mouse and rat during postnatal period are opposite to those of Fabp4 (Liu et al., 2011; Lee & Kim, 2018). In addition to differential expression of adipocyte-associate genes between species and at different postnatal ages, regional difference of the epididymal fat on gene expression has been observed. Tirard et al. (2007) have shown that histochemically distinct two epididymal regions, proximal and distal, are present. The proximal epididymal fat part is in direct contact with the epididymis, and the distal epididymal fat region is extended toward the abdominal cavity (Tirard et al., 2007). Moreover, different expressional patterns of aldo-keto reductase 1B7, cyclooxygenase 2, and GATA-3 genes between proximal and distal epididymal fat have been observed (Tirard et al., 2007). The comparison of findings from our previous and other researches also supports the existence of regional difference of epididymal fat on gene expression of adipocyte-associated molecules (Liu et al., 2011; Lee & Kim, 2019). However, direct comparison of expressional patterns of adipocyte-associated molecules between different epididymal fat region of mouse during postnatal period has not been carried out.
In the present research, expressional patterns of some adipocyte-associated genes in the proximal epididymal region of mouse at different postnatal ages had been evaluated with quantitative real-time polymerase chain reaction analysis. From the current study, we attempted to reveal the regional variance in the mouse epididymal fat on gene expression of adipocyte-associated molecules.
MATERIALS AND METHODS
Male C57BL/6N mice at 1 month of postnatal age were obtained from Samtako (OSan, Korea). At the arrival, the mice were randomly assigned into four different groups, a young adult group (2 months of age), two fully mature adult group (5 and 8 months of age), and an old group (12 months of age), and each experimental group had six mice. The animals were maintained in controlled conditions and permitted for food and water ad libitum. This work was carried out in accordance with the guide for the care and use of laboratory animals of National Research Council in Korea.
At the proper postnatal age, the animal was euthanized by CO2 stunning in a chamber. An incision was made on lower abdominal part, and the male reproductive tract was pulled out through the incision (Fig. 1). In cold-PBS buffer, the whole epididymal fat pad was dissected out from the rest of male reproductive tract. The proximal epididymal fat surrounding the efferent ductules was carefully separated from the distal epididymal fat, the peripheral part of epididymal fat pad. Two parts of the epididymal fat pad were rapidly frozen in liquid nitrogen and separately stored in –80°C for further use.
One milliliter of TRI REAGENT solution (Molecular Research Center, Inc, Cincinnati, OH) was applied to each proximal epididymal fat sample for homogenization. Then, chloroform and isopropanol were sequentially added to extract total RNA from tissue homogenate, and an acquired total RNA pellet was re-dissolved in DEPC-dH2O. The yield and concentration and quality of total RNA were evaluated by the NanoDrop Lite spectrophotometry (Thermo Scientific, Wilmington, DE) and 1.2% agarose gel electrophoresis, respectively.
One microgram of total RNA was used to construct the first-stranded complementary DNA (cDNA) by reverse transcription (RT) reaction in iScriptTM Reverse transcription Supermix for RT-qPCR (Bio-Rad Laboratories. Inc., Hercules, CA). The total RNA was mixed with oligo-dT primer, RTase, 5X buffer, and nuclease-free dH2O, and the final volume of a RT reaction was 20 μL. The RT reaction was performed in 25°C for 5 min, 46°C for 20 min, and 95°C for 1 min, and generated cDNA was directly utilized for quantitative real-time polymerase chain reaction (PCR).
Table 1 provides information of oligonucleotide primers for molecules analyzed by quantitative real-time PCR. Primer 3 software (http://www.bioneer.co.kr/cgi-bin/primer/primer3.cgi: Whitehead Institute/MIT Center for Genomes Research, USA) was used to design the PCR primers.
One microlitter of cDNA was mixed with 10 pmol of each primer, 7 μL of iQ™ SYBR® Green Supermix (Bio-Rad Laboratories), and dH2O to make 20 μL of PCR mixture. The condition of PCR was a pre-denaturation at 95°C for 5 min, cycles of a denaturation at 95°C for 30 sec, an annealing at Tm for 30 sec, and an extension at 72°C for 30 sec, and an additional extension at 72°C for 10 min. The expected size of PCR product was confirmed with 1.2% agarose gel electrophoresis.
As an internal control of PCR, 18S ribosomal RNA (Rn18s) was added for quality control purpose. In order to get a mean and standard error for expressional level of a particular gene at an age, independently quadrupled or quintupled RT reactions and PCRs were executed. The expressional levels of target genes were normalized with that of Rn18s and were expressed as a relative ratio against Rn18s.
To determine statistical significance of expressional levels of the particular molecule among experimental groups at a postnatal age, one-way ANOVA analysis was first carried out. If there was a statistical significance present, Duncan’s test, a post-hoc analysis, was followed. If p value was less than 0.05, it was considered that there was significant difference among experimental groups, presented in different letters.
RESULTS
The expression of Pparg was significantly decreased at 5 months of age, compared with that at 2 months of age (Fig. 2A). However, a surge, over 5-folds increase, of Pparg transcript level was observed at 8 months of age (Fig. 2A). There was no significant change of Pparg expressional level at 12 months of age, compared with at 8 months of age (Fig. 2A).
The change of transcript level of Dlk1, delta like non-canonical Notch ligand 1 (Dlk1) at 5 months of age was not significantly different with that at 2 months of age (Fig. 2B). But, a significant increase of Dlk1 transcript level was detected at 8 months of age (Fig. 2B). A further increase of Dlk1 expressional level was observed at 12 months of age (Fig. 2B).
Similar to Pparg, a significant decrease of fatty acid synthase (Fasn) transcript level at 5 months of age was found (Fig. 3A). The expression Fasn was however greatly increased at 8 months of age, compared with that at 5 months of age (Fig. 3A). At 12 months of age, the level of Fasn transcript was significantly lower than that at 8 months of age, but still higher than that at 2 and 5 months of age (Fig. 3A).
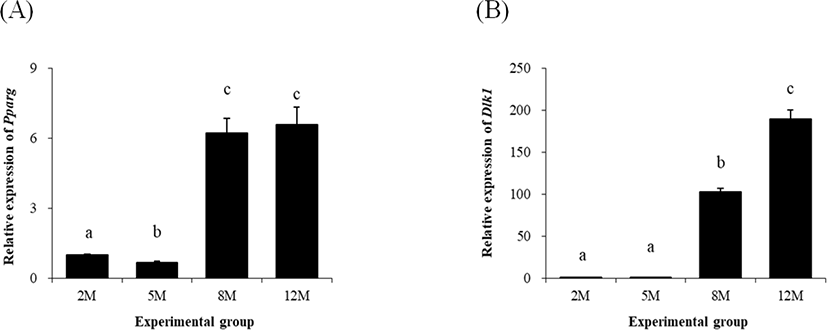
The level of lipoprotein lipase (Lpl) transcript at 5 months of age was significantly lower than that at 2 months of age (Fig. 3B). But, the expressional level of Lpl at 8 months of age was much higher than those at 2 and 5 months of age (Fig. 3B). The transcript level of Lpl at 12 months of age was significantly decreased, but was higher than those at 2 and 5 month of age (Fig. 3B).
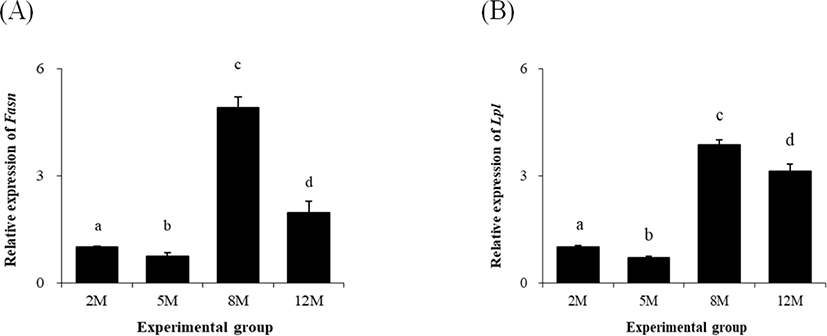
The lowest level of leptin (Lep) in the proximal epididymal fat of mouse was detected at 2 months of age (Fig. 4A). The abundance of Lep transcript was significantly increased at 5 months of age, and an additional extension of Lep transcript level was observed at 8 months of age (Fig. 4A). However, expressional level of Lep at 12 months of age was significantly lower than that at 8 months of age and comparable with that at 5 months of age (Fig. 4A).
The level of Retn expression was not significantly changed between 2 months and 5 months of age (Fig. 4B). But, the level of Retn transcript was significantly increased at 8 months of age, followed by a considerable drop at 12 months of age, which was yet higher than those at 2 months and 5 months of age (Fig. 4B).
Expressional level of adiponectin (Adipoq) at 5 months of age was not significantly changed, compared with that at 2 months of age (Fig. 5A). But, a huge increase of Adipoq transcript level was detected at 8 months of age (Fig. 5A). The level of Adipoq transcript was significantly lower than that at 8 months of age but still higher than those at 2 months and 5 months of age (Fig. 5A).
The expressional pattern of Fabp4 was quietly similar with that of Adipoq, as shown in Fig. 5B. The level of Fabp4 at 5 months of age was not significantly different with that at 2 months of age (Fig. 5B). However, over 100-fold increases of Fabp4 transcript level was observed at 8 months of age (Fig. 5B). The level of Fabp4 transcript at 12 months of age was significantly lower than that at 8 months of age but significantly higher than those at 2 months and 5 months of age (Fig. 5B).
DISCUSSION
Expressional levels of adipocyte-associated molecules in the proximal epididymal fat at the old age were mostly higher than that at the early age, even though expressional patterns of some genes were different with those of other genes. Except Dlk1, the highest transcript levels of other adipocyte-associated molecules in the proximal epididymal fat were detected at 8 months of postnatal age.
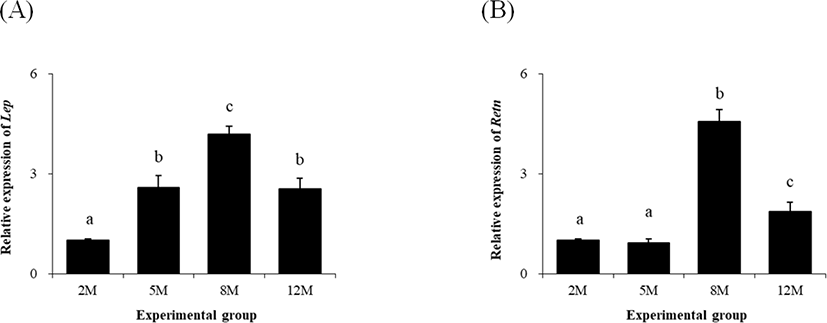
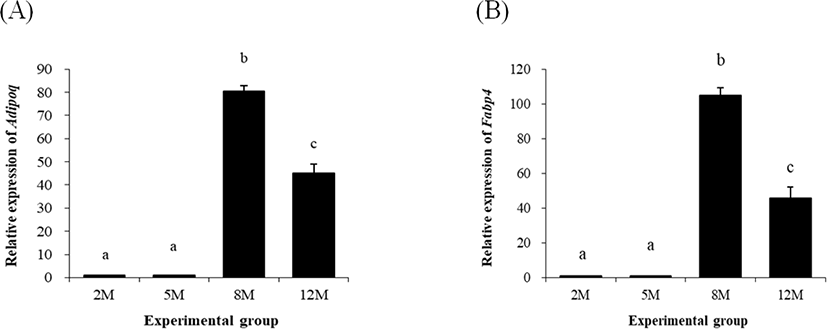
The leptin, resistin, and adiponectin are most prominent adipocytokines secreted from adipose tissue, primarily involving in regulation of energy balance among tissues at various levels (Cao, 2014). It is well recognized that leptin regulates the release of secretory molecules from hypothalamus for control of food intake and energy spend (Cao, 2014). Leptin also exerts its effect on the male reproduction, including regulation of spermatogenesis, development of puberty, and sperm maturation (Zhang & Gong, 2018). Liu et al. (2011) have shown an increase of Lep in the epididymal fat of aging mouse, and our previous research has also revealed gradual increase of Lep in the distal epididymal fat of mouse with age (Lee & Kim, 2019). However, the present study detected that the level of Lep transcript in the proximal epididymal fat was significantly decreased at 12 months, compared to the level at 8 months of age. Together, these results suggest that an overall increase of Lep expression in the mouse epididymal fat at 12 months of age is likely influenced more by expressional change of Lep in the distal epididymal fat than by expressional change of Lep in the proximal epididymal fat.
Expressional levels of Adipoq and Retn in the rat whole epididymal fat are increased with age (Lee & Kim, 2018) and in the mouse distal epididymal fat (Lee & Kim, 2019). Interestingly, the present research shows the highest transcript levels of Adipoq and Retn in the mouse proximal epididymal fat at 8 months of age, followed by significant decreases at 12 months of age. Ouchi et al. (2003) have shown a decrease of Adipoq expression in adipose tissue as body mass is increased. Indeed, Liu et al. (2011) have revealed a decreased expressional level of Adipoq in the mouse epididymal fat at 14 months of age, compared with that at 6 months of age. However, from our previous findings, the level of Adipoq transcript in the mouse distal epididymal fat at 12 months of age is significantly higher than that at 5 months of ag and as close as that at 8 months of age (Lee & Kim, 2019). These observations suggest that an increase of Adipoq expression in the mouse epididymal fat until 8 months of age could be a synergic effect of proximal and distal epididymal fat, while a decrease of Adipoq transcript level at 12 months of age would be a results from the proximal epididymal fat overwhelming the effect observed in the distal epididymal fat. Expressional patterns of Retn in different mouse epididymal fat parts of mouse are very close to those of Adipoq. Thus, it is speculated that overall level of Retn transcript at 12 months of age would be lower than that at 8 months of age. It is considered that adiponectin and resistin exert opposite effects on insulin-resistance and atherogenesis (Cao, 2014). However, based on the findings of similar expressional patterns of Adipoq and Retn in the epididymal fat, it is assumed that adiponectin and resistin would play a physiological role in the epididymal fat and/or male reproductive tract in unknown manner.
The expressional level of Fabp4 in the mouse epididymal fat is decreased with age (Liu et al., 2011). However, our previous study has shown a gradual increase of Fabp4 in the distal epididymal fat of mouse (Lee & Kim, 2019). It has been suggested that such disagreement on expressional patterns of Fabp4 could be due to differential expression of Fabp4 between the proximal and distal epididymal fat part (Lee & Kim, 2019). Indeed, the present study shows that the expressional level of Fabp4 at 12 months of age is significantly lower.
Than that at 8 months of age. Thus, it is reasonable to consider that a decrease of Fabp4 transcript level in the mouse epididymal fat at the old age would be a result of a reduction of Fabp4 expression in the proximal part of mouse epididymal fat. As a fatty acid binding protein, FABP4 promotes the accumulation of lipid droplets in adipogenic cells (Kletzien et al., 1992). Because the size of fat cells becomes enlarged and the storage of lipid components are increased as aged (Cleary et al., 1977), it is thought that expression and/or activity of FABP4 in the epididymal fat at the old age would be higher than that at the young age. A future research is suggested to examine posttranscriptional regulation of Fabp4 expression to retain FABP4 protein and/or activity level in the epididymal fat at the old age.
Dlk-1, a.k.a. preadipocyte factor-1(Pref-1), is an inhibitory factor of adipogenesis to suppress the differentiation of preadipocyte into mature adipocyte (Wang & Sul, 2009). Thus, expression of Dlk-1 is higher in preadipocyte than in mature adipocyte (Wang & Sul, 2009). In the rat epididymal fat, expressional level of Dlk-1 is extraordinarily high at the early postnatal age, and basal level of Dlk-1 transcript is detectable at 5 months of age (Lee & Kim, 2018). However, the expression of Dlk-1 in the rat epididymal fat at old age is significantly increased (Lee & Kim, 2018). Interestingly, our previous (Lee & Kim, 2019) and current researches have demonstrated the basal expression of Dlk-1 at 2 and 5 months of age and surges of Dlk-1 expressional level at 8 and 12 months of age in the mouse distal and proximal epididymal fat regions. These observations imply that Dlk-1 in the epididymal fat would act a factor with unknown function, other than inhibiting differentiation of preadipocyte to adipocyte. Further researches are suggested to examine possible regulatory effect of Dlk-1 derived from the epididymal fat on the testicular functions.
Of several adipogenic factors, Pparg is a key regulator for differentiation of preadipocyte into mature adipocyte and preservation of differentiated status of mature adipocyte (Tamori et al., 2002). The expressional level of Pparg in the distal epididymal fat part of mouse is gradually increased with aging (Lee & Kim, 2019). Even though a similar expressional pattern of Pparg is also found in the proximal epididymal fat from the present research, a significant decrease of Pparg at 5 months of age in the proximal epididymal fat is a notable observation different with results acquired from the distal epididymal fat region. There is no clear explanation suggested for such change on Pparg expressional pattern. It is possible that expressional decrease of Pparg at 5 months of age in the proximal epididymal part would be negligible in the level of a whole epididymal fat. This suggestion is partly supported by Liu et al.’s findings (2011), showing a significantly higher level of Pparg transcript at 14 months of age than that at 6 months of age.
Lipolysis, a process of triglyceride hydrolysis, and lipogenesis, a synthetic pathway to store fatty acid, are found in adipocyte, and these two opposite mechanisms are regulated by various molecules, including LPL and FASN, respectively. Even though expressional level of Lpl in the rat epididymal fat is decreased as aged (Kirkland et al., 1993), our previous (Lee & Kim, 2019) and current researches have shown the higher level of Lpl transcript level in both proximal and distal epididymal fat regions at the old age than that at early postnatal age. It is notable that expressional level of Lpl at 5 months of age in the proximal epididymal fat region is significantly lower than that at 2 months of age. This result is absolutely opposite to our expectation described from the previous report (Lee & Kim, 2019), assuming a significant increase of Lpl expression in the proximal epididymal fat at 5 months of age. Moreover, expressional level of Lpl in the proximal epididymal fat at 12 months of age is unexpectedly lower than at 8 months of age. Expressional pattern of Fasn in the proximal epididymal fat is quite similar with that of Lpl. However, unlike in the distal epididymal fat (Lee & Kim, 2019), expressional level of Fasn in the proximal epididymal fat at 12 months of age is substantially reduced, even though significant decreases of Fasn transcript level at 5 months of age are noticeable in both proximal and distal epididymal fat regions. Such unanticipated expressional patterns of Lpl and Fasn in the mouse epididymal fat at 5 and 12 months of age are not understandable at this point. A functional study of Lpl and Fasn in the mouse epididymal fat at a given postnatal age would provide possible explanation for such fluctuation of postnatal expression.
In summary, the current study exhibits the differential patterns of gene expression of adipocyte-associated molecules between the proximal and distal epididymal fat parts of mouse during postnatal period. The function of epididymal fat pad is not clearly elucidated yet. Chu et al. (2010) have demonstrated that the surgical removal of epididymal fat causes the cease of spermatogenesis in the testis, without a change of serum testosterone or luteinizing hormone concentration. These observations imply that locally-acting factor(s) derived from the epididymal fat would influence on the regulation of spermatogenesis in the testis. Our previous (Lee & Kim, 2019) and current researches have revealed expressional patterns of adipocyte-associated molecules in different two epididymal fat regions of mouse during postnatal period. Such observations suggest the existence of different regulatory mechanisms for adipocyte-associated gene expression in these epididymal fat regions. Even though exact functions of these adipocyte-associated molecules in the epididymal fat have not been elucidated, it is carefully suggested that the secretory molecules derived from the epididymal fat, such as leptin, resistin, and adiponectin, would influence on the regulation of testicular functions.