INTRODUCTION
Numerous studies have demonstrated that nonylphenol (NP) is an endocrine disrupting chemical (EDC) possessed negative effects on reproductive health (Noorimotlagh et al., 2017; Rattan et al., 2018). In rodents, administration of NP caused dysfunction of postpubertal reproductive function in female rats as well as disrupted development of gonads in male and female rats (Nagao et al., 2000), decreased epididymal weights and sperm counts (Han et al., 2004), decreased weight/volume of testis and decreased number of germ cell and Sertoli cells (Mehranjani et al., 2009), impaired sperm motility and destruction of sperm function (Uguz et al., 2009), and destruction of blood-testis-barrier in testes (Lu et al., 2014). Previously, we reported that the subchronic low-dose NP exposure resulted in adverse effects on the reproductive system of male and female F1 mice, such as decreased weight of ovary and uterus in F0 animals, decreased rate of ovulation in F1 offsprings, increased weight of the testis, and decreased weights of the epididymis, prostate and seminal vesicle (Cha et al., 2017; Kim et al., 2019).
Some EDCs such as diethylstilbesterol (DES), vinclozolin, methoxychlor and phthalates has been intensively studied on their potent transgenerational effects (Newbold et al., 2000; Anway et al., 2006; Guerrero-Bosagna & Skinner MK, 2009; Stouder & Paoloni-Giacobino, 2011; Doyle et al., 2013). Only a handful of studies, however, have been carried out to investigate the multi-generational effects the NP on the reproductive system, and the results were controversial (Chapin et al., 1999; Kyselova et al., 2003; Tyl et al., 2006). We hypothesized that the uncertainty on the multi-generational NP effects could be derived from the difference of exposure dose between the studies. The dose employed in our previous studies (18.5–19.8 ug/kg/day) was far lower than the no-observed-adverse-effect level (NOAEL, 50 mg/kg/day in rat), while the doses about to or over the NOAEL have been used in most of studies on the endocrine disrupting nature of NP.
In the present study, we used the subchronic low-dose NP exposure model to clarify the multi-generational effect of NP on the reproductive system of F2 generation male mice.
MATERIALS AND METHODS
ICR mice were provided by DBL (Chungcheongbuk-do, Korea) and reared in Sangmyung University animal facility under photoperiods of 12h light/dark with lights on at 7 AM and constant temperature of 21–23°C. Food and tap water were supplied ad libitum. The animal protocols were approved by the Animal Care and Use Committees at Sangmyung University (approval number R-1601-1). All the animals received human care in accordance with the guides for animal experiments of the Association for Assessment and Accreditation of Laboratory Animal Care (AAALAC).
After acclimation, breeding pairs of mice were divided into 2 groups; (1) Control (CTL), supplied tap water, and (2) NP-50, treated with low-dose NP (50 μg/L; Sigma, USA) via drinking water. The parental (P) generation mice had pre-mating period for 2 weeks. After pre-mating period, male and female mice were placed in the breeding cage until a vaginal plug of female mice was observed. The animals were treated with NP from pre-mating period to weaning of offsprings (F1). The F1 generation mice were weaned at postnatal day (PND) 21 and consistently treated with the NP, and when the male and female mice reached sexual maturity, the same breeding protocol which was applied to P generation mice was used. The offspring F2 generation mice were weaned at PND 21, and their anogenital distance (AGD) were measured with a digital caliper on PND 35, 40, 45, 50, and 55. Animals were sacrificed at PND 55, and sample collections were done between 18:00–19:00 pm. The weights of reproductive tissues (testis, epididymis, seminal vesicle and prostate) were measured. Tissues were fixed in paraformaldehyde (4%) for histological study.
Fixed tissues (testes and caudal edpidydimi) were dehydrated in graded concentrations of ethanol (70%, 80%, 90%, 95%, and 100%; Duksan, Korea) for 1 hour 30 minutes in each with gentle shaking and soaked in absolute ethanol overnight. The tissues were immersed in xylene (Samchun Chemical, Korea) for 30 minutes, 3 times and in paraffin (Avantik Biogroup, USA) at 56°C for 30 minutes, 3 times. The tissues were embedded in paraffin and sectioned (Microm, Germany) at 5 μm. The samples were attached on microscope slides (Marienfeld, Germany) and the slides were stained with hematoxylin (Sigma, USA) and eosin (Across, USA) for 5 minutes, respectively.
RESULTS
To find the effect of chronic low-dose exposure to NP on the weight gain of F2 male mice, we measured and compared the body weights at weaning day (PND 21) and at sacrifice day (PND 55) (Table 1). The initial body weights of the NP-50 group animals were not significantly different from those of control group animals. Also the terminal body weights of the NP-50 group animals were not significantly different from those of control animals.
CON | NP-50 | |
---|---|---|
Initial body weight (g) | 10.8±0.4 | 11.2±0.5 |
Terminal body weight (g) | 33.8±0.8 | 32.5±0.5 |
Increasing body weight (%) | 315±14 | 292±16 |
Values are expressed as mean±SE (n=9).
*p<0.05, significantly different from the control group.
NP, nonylphenol; PND, postnatal day; CON, control group provided with tap water only; NP-50, treated with nonylphenol at a dose of 50 μg/L.
Table 2 indicates that there was no statically significant difference in the AGD indices between control and NP-treated male mice from PND 35 to PND 55.
PND | CON | NP-50 | |
---|---|---|---|
Anogenital distance (mm) | 35 | 17.3±0.42 | 17.3±0.29 |
40 | 19.0±0.45 | 19.3±0.49 | |
45 | 19.9±0.41 | 19.8±0.47 | |
50 | 20.6±0.37 | 20.4±0.33 | |
55 | 21.3±0.38 | 21.2±0.42 |
Values are expressed as mean±SE (n=9).
NP, nonylphenol; PND, postnatal day; CON, control group provided with tap water only; NP-50, treated with nonylphenol at a dose of 50 μg/L.
As shown in Table 3, chronic low-dose NP exposure failed to induce any significant change in the absolute and relative weights of reproductive organs in F2 male mice, except the weights of the epididymis (p<0.05) when compared to control. However, the relative tissue weights were not significantly different between two groups.
Values are expressed as mean±SE (n=9).
*p<0.05, ***p<0.001, significantly different from the control group value by t-test.
NP, nonylphenol; CON, control group provided with tap water only; NP-50, treated with nonylphenol at a dose of 50 μg/L.
The overall histological appearances of the testis and caudal epididymis were similar to those of F1 mice organs (Kim et al., 2019). In the control testis, normal cellular architectures of seminiferous tubule were observed; tight Sertoli-Germ cell junctions were distinct and mature spermatids were abundant in the lumens (Fig. 1. CON). On the other hand, the volume of seminiferous tubule and its diameter, thickness of the basement membrane, luminal area and number of germ cell were decreased in the NP-treated testis (Fig. 1. NP-50). Sloughed and degenerate germ cells were much frequent in the NP-treated testis. Numerous mature sperms were found in the caudal epi_didymis of control animal (Fig. 2. CON). On the contrary, fewer mature sperms were found in the caudal epididymis of NP-treated animal (Fig. 2. NP-50). Notably, swollen epithelial cells were found in NP-50 group showing irregular cell arrangement.
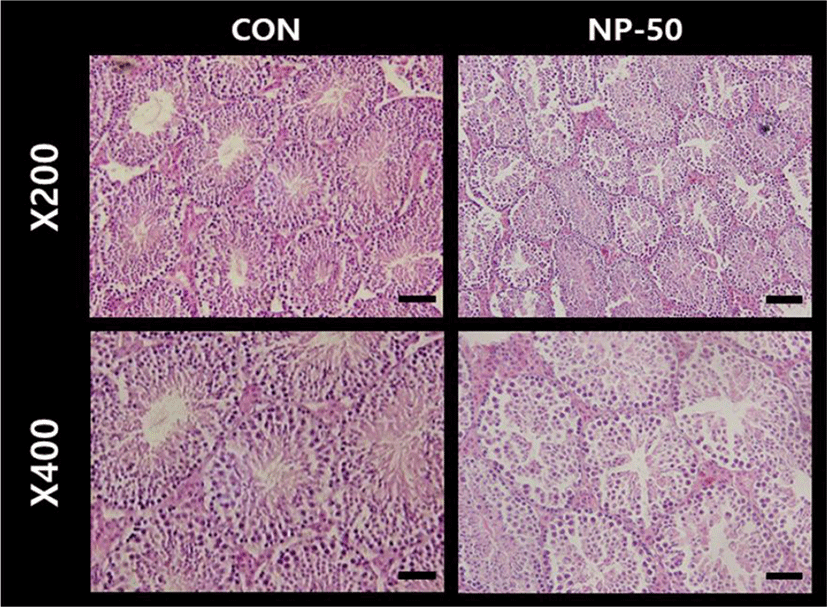
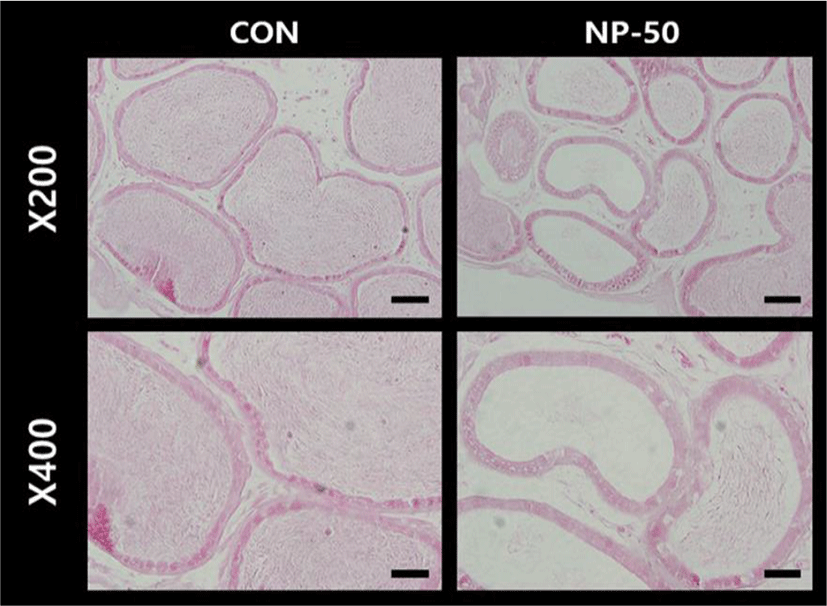
DISCUSSION
After the first report that NP has estrogenic effect in mammals (Soto et al., 1991), considerable evidence has accumulated indicating that NP could interfere with reproductive system (Nagao et al., 2001; Gong et al., 2009; Sato et al., 2009; Duan et al., 2016; Di et al., 2018). In particular, due to its estrogenic nature, relatively severe reproductive toxicity was found in NP-exposed male rodents. Para-nonylphenol (p-NP) exposure induced remarkable changes in testis architecture and significant reductions in number of germ cells and Sertoli cells in rat (Mehranjani et al., 2009). In vitro and in vivo studies indicated that NP could disrupt the structure and function of Sertoli cells, and decreased serum hormone levels in prepubertal male rats (Hu et al., 2014). Rat epididymal sperms exposed to NP exerted significantly worsening sperm parameters such as motility and acrosomal reaction (Uguz et al., 2009). Likewise, sperm counts in cauda and caput/corpus epididymides, sperm motility, daily sperm production and sperm transit time in cauda epididymis were remarkably decreased in rats gavaged with high-dose (100–300 mg/kg BW) NP for 30days (Aly et al., 2012). Previously we demonstrated that the decreases of absolute weights in the accessory sex organs and the pathohistological changes in testis and epididymis of low-dose NP treated F1 mice, showing good agreement with the adverse effects of NP exposure on male rodents in the published reports (Kim et al., 2019). In the present study, we found that no change in numerical parameters such as tissue weight and AGD in NP exposed F2 mice, but still there were almost identical patterns of pathohistologic alteration in the testis and caudal epididymis as shown in F1 mice. For examples, multiple sloughing morphologies in seminiferous tubules, decreases in tubule diameter, reduced luminal area and number of germ cells were observed in the tissue sections from NP-treated F2 mice. With the apparent reduction in luminal sperm number from NP-treated epididymis, the present study strongly indicate the impaired spermatogenesis and detrimental effect on epididymal sperms in NP exposed F2 mice. These results support a low sperm count of NP-treated animals in the previous studies (Chitra et al., 2002; Uguz et al., 2009; Aly et al., 2012), and could be an explanation clue for a global decline in human sperm quality and count (Sifakis et al., 2017). Concerning the molecular action mechanism, NP-induced apoptosis, autophagy and necrosis in rat Sertoli cell seem to be through reactive oxygen species (ROS) mediated pathway (Duan et al., 2016). Previous studies using boar and ram spermatozoa demonstrated that mitochondrial dysfunction could result in the formation of ROS (Uguz et al., 2015). In rat study, chronic oral administration of NP (250 mg/kg/day for 90 days) induced apoptosis and oxidative stress in epididymal sperm (Lu et al., 2014). Further studies on the NP action mechanism will be helpful to solve the problem with male infertility crisis.
There is general consensus among researchers that human EDC exposure is closely related to the lifestyle, and the lifestyle behaviors associated with EDCs exposures could be causes of low fertility and even serious diseases such as diabetes in adults, and could be passing through successive generations of family members (Martina et al., 2012; Lee, 2018). Several major EDCs has been studied on their potent transgenerational effects. DES exposure of the F1 generation mice during embryonic and early postnatal development at a range of dosages had adverse consequences in the F2 generations; in F2 males, the incidence of proliferative lesions of the testes was increased, and serum estradiol concentration was reduced (Newbold et al., 2000; Walker & Gore, 2011). Exposure to vinclozolin promotes transgenerational transmission of adult-onset disease states such as increased frequencies of tumors, prostate disease, kidney diseases, and immune abnormalities that develop as males age (Guerrero-Bosagna & Skinner, 2009). Particularly, the reduced spermatogenetic capacity observed in the F1 male offspring was transmitted to the subsequent generations (F2–F4) (Anway et al., 2006). Methoxychlor treatment of pregnant mice decreased the mean sperm concentrations by 30% and altered the methylation pattern of all the imprinted genes tested in the F1 offspring, and the methoxychlor effects were transgenerational but disappeared gradually from F1 to F3 (Stouder & Paoloni-Giacobino, 2011). Embryonic exposure to Di-2-ethylhexyl phthalate (DEHP) was found to disrupt testicular germ cell organization and decreased sperm count and motility in F1 to F4 offspring in a transgenerational manner, and spermatogonial stem cell (SSC) function of F3 offspring (Doyle et al., 2013).
Concerning the multi-generational effects the NP on the reproductive system, limited number of studies is available and the results were controversial. Sperm indices were unchanged in NP-exposed F0 and F1 males (30–100 or 100–350 mg/kg/day), but the testicular spermatid count and the epididymal sperm density were reduced while the testis and epididymis weights were unchanged in F2 rats (Chapin et al., 1999). A significant reduction in acrosomal integrity was seen in F1 and F2 generations of NP-treated CD-1 mice (50–500 μg/L in drinking water), and fewer offspring were observed in the second litter of the F2 generation of mice treated with NP (Kyselova et al., 2003). On the other hand, there was no NP (approximately > 150 mg/kg/day) effects on any reproductive parameter in any generation, including sperm counts of CD rats (Tyl et al., 2006). We hypothesized that the uncertainty on the multi-generational NP effects could be derived from the difference of exposure dose between the studies. The dose (18.5–19.8 ug/kg/day) employed in our previous (Kim et al., 2019) and the present studies was far lower than the NOAEL, while the doses about to or over the NOAEL have been used in most of studies on the endocrine disrupting nature of NP. Since the average daily human intake of NP was calculated to be 7.5 ug/day in Germany and to be about 25.32 ug/day in Taiwan (Guenther et al., 2002; Lu et al., 2007), the low dose used in our studies could be better to obtain practical information on the adverse effect of NP exposure to human. Unlike the deletion or substitution of nucleotide(s), the range of epigenetic modification such as DNA methylation, histone modification, altered DNA accessibility and chromatin structure induced by EDCs exposure might be variable (Xin et al., 2015). So one can assume that more of the inter-individual variability in abnormal status could be seen when there is a great difference of EDC exposure dose between studies.
In conclusion, though we found no numerically significant change in the body and tissue weights in chronic low-dose NP exposed F2 male mice, our histological studies revealed that the abnormal structures of testicular seminiferous tubules and reduced number of germ cells in the tubules and epididymi of F2 mice, showing the coterminous states with those of F1 mice. This ‘qualitative’ change of reproductive tissues found in our study could be derived from the epigenetic reprogramming by low-dose NP exposure which is frequently experienced in daily living. Therefore, further studies with careful design of dose and exposure period are needed to achieve a better understanding on the multi- or trans-generational effects of NP on the reproductive health and a human application.