INTRODUCTION
Nonylphenol (NP), a subset of alkylpenol ethoxylates, is widely used as surfactant in the manufacturing industry and is present in detergents, paints, pesticides, personal care products, and plastics (de Weert et al., 2008). Since the original findings that NP has the estrogenic activity (Soto et al., 1991), previous studies demonstrated and suggested that NP was an endocrine disrupting chemical (EDC) and could interfere with reproductive system in rodents (Nagao et al., 2001; Gong et al., 2009; Mehranjani et al., 2009; Uguz et al., 2009; Aly et al., 2012; Lu et al., 2014). In human, presence of NP and its metabolites in several tissues and blood is evident (Muncke, 2009; Huang et al., 2016; Acir & Guenther, 2018). So it is certainly possible that human exposure to NP could induce reproductive toxicity as found in the rodent studies. Indeed, possible detrimental effects of NP on male fertility were systematically documented in recent review studies (Noorimotlagh et al., 2017; Noorimotlagh et al., 2018). To confirm the adverse effects of NP on human, however, the low-dose tests that reflect the levels in range similar to those found in human bodies should take precedence. In addition, most of NP exposure occurs in living environment, so relatively long-term exposure via drinking water is suitable for simulating routine human exposure.
Present study was undertaken to clarify the effect of subchronic low-dose NP exposure to F1 generation male mice. To fulfill this goal, we employed the Organization for Economic Cooperation and Development (OECD) test guideline 443, an ‘extended one-generation reproductive toxicity study’. The dose (50 μg/L) and delivery method (via drinking water) were chosen based on the previous studies that demonstrated the adverse effects on reproductive organs in mice (Kyselova et al., 2003; Cha et al., 2017).
MATERIALS AND METHODS
ICR mice were provided by DBL (Chungcheongbuk-do, Korea) and reared in Sangmyung University animal facility under photoperiods of 12 h light/dark with lights on at 7 AM and constant temperature of 21–23℃. Food and tap water were supplied ad libitum. The animal protocols were approved by the Animal Care and Use Committees at Sangmyung University (approval number R-1601-1). All the animals received human care in accordance with the guides for animal experiments of the Association for Assessment and Accreditation of Laboratory Animal Care (AAALAC).
After acclimation, breeding pairs of mice were divided into 2 groups; (1) Control (CTL), supplied tap water, and (2) NP-50, treated with low-dose NP (50 µg/L; Sigma, USA) via drinking water. The parental generation mice had pre-mating period for 2 weeks. After pre-mating period, female and male mice were placed in the breeding cage until a vaginal plug of female mice was observed. The animals were treated with NP from pre-mating period to weaning of offsprings (F1). Then F1 generation mice were weaned at postnatal day (PND) 21 and consistently treated with the NP until sacrifice. Anogenital distance (AGD) were measured with a digital caliper on PND 35, 40, 45, 50, and 55. Animals were sacrificed at PND 55, and sample collections were done between 18:00–19:00 pm. The weights of reproductive tissues (testis, epididymis, seminal vesicle and prostate) were measured. Tissues were fixed in paraformaldehyde (4%) for histological study.
Fixed testes were dehydrated in graded concentrations of ethanol (70%, 80%, 90%, 95%, and 100%; Duksan, Korea) for 1 hour 30 minutes in each with gentle shaking and soaked in absolute ethanol overnight. The tissues were immersed in xylene (Samchun Chemical, Korea) for 30 minutes, 3 times and in paraffin (Avantik Biogroup, USA) at 56℃ for 30 minutes, 3 times. The tissues were embedded in paraffin and sectioned (Microm, Germany) at 5 μm. The samples were attached on microscope slides (Marienfeld, Germany) and the slides were stained with hematoxylin (Sigma, USA) and eosin (Across, USA) for 5 minutes, respectively.
RESULTS
In order to evaluate the effect of chronic low-dose exposure to NP on the weight gain of F1 male mice, we measured and compared the body weights at weaning day (PND 21) and at sacrifice day (PND 55) (Table 1). The initial body weights of the NP-50 group animals were significantly lower than those of control group animals (p<0.05). Likewise, the terminal body weights of the NP-50 group animals were significantly lower than those of control group animals (p<0.05). However, the weight gains were not significantly different between two groups.
CON | NP-50 | |
---|---|---|
Initial body weight (g) | 11.5±0.3 | 10.2±0.5* |
Terminal body weight (g) | 30.3±0.5 | 28.7±0.5* |
Increasing body weight (%) | 260±4 | 285±13 |
Values are expressed as mean±SE (n=9).
CON, control group provided with tap water only; PND, postnatal day; NP-50, treated with nonylphenol at a dose of 50 μg/L.
Table 2 indicates that there was no statically significant difference in the AGD indices between control and NP-treated male mice from PND 35 to PND 55.
PND | CON | NP-50 | |
---|---|---|---|
Anogenital distance (mm) | 35 | 18.6±0.38 | 18.3±0.33 |
40 | 19.4±0.32 | 19.4±0.24 | |
45 | 20.1±0.29 | 20.1±0.20 | |
50 | 20.8±0.23 | 20.9±0.15 | |
55 | 21.4±0.15 | 21.4±0.12 |
Values are expressed as mean±SE (n=9).
NP, nonylphenol; PND, postnatal day; CON, control group provided with tap water only NP-50, treated with nonylphenol at a dose of 50 μg/L.
As shown in Table 3, chronic low-dose NP exposure induced a significant increase in the absolute weight of the testes (p<0.05). Conversely, the NP exposure caused significant decrease in the absolute weights of the epididymis (p<0.01), prostate (p<0.05) and seminal vesicle (p<0.05) compared to the levels of control animals. However, the relative tissue weights were not significantly different between two groups.
Values are expressed as mean±SE (n=9).
CON, control group provided with tap water only; NP-50, treated with nonylphenol at a dose of 50 μg/L.
In the control testis, normal cellular architectures of seminiferous tubule were observed; tight Sertoli-Germ cell junctions were evident and spermatids were abundant in the lumens (Fig. 1. CON). On the other hand, the volume of seminiferous tubule and its diameter, thickness of the basement membrane, luminal area and number of germ cell were decreased in the NP-treated testis (Fig. 1. NP-50). Also, some sloughing morphologies were observed in the NP-treated testis. Numerous mature sperms were found in the caudal epididymis of control animal (Fig. 2. CON). On the contrary, fewer mature sperms were found in the caudal epididymis of NP-treated animal (Fig. 2. NP-50). Notably, swollen epithelial cells were found in NP-50 group showing irregular cell arrangement.
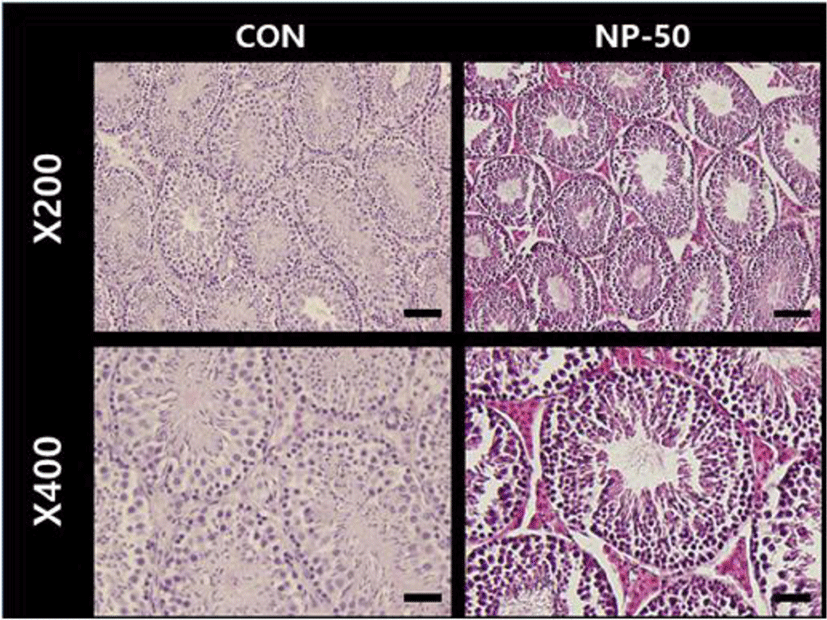
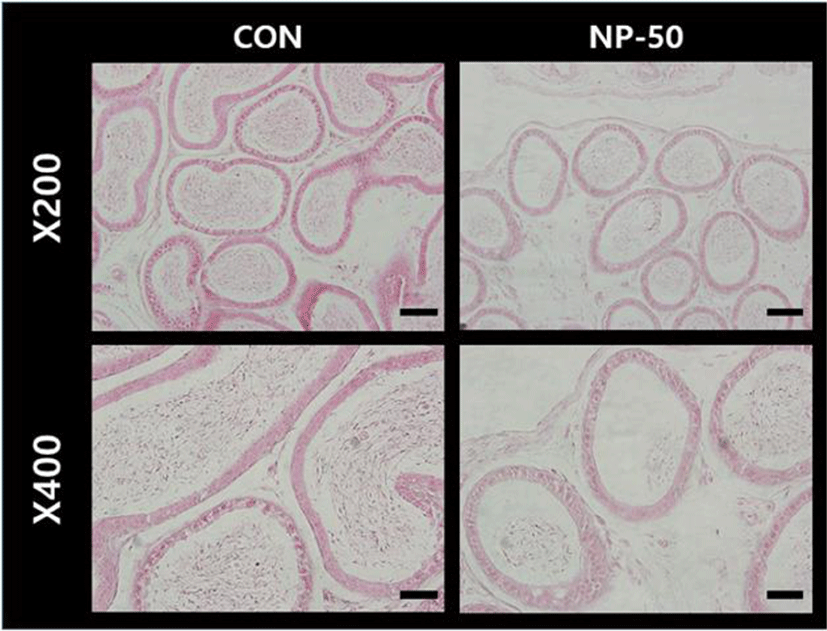
DISCUSSION
The estrogenic potential of NP in mammals has been well established since early 1990s (Soto et al., 1991). Concerning endocrine disturbing activities of NP, rodent studies clearly demonstrated and suggested that NP could interfere with both male and female reproductive system (Nagao et al., 2001; Gong et al., 2009; Sato et al., 2009; Duan et al., 2016; Di et al., 2018). More specifically, ample evidence has been accumulated confirming the adverse effects of NP on the testis and epididymis. Exposure to para-nonylphenol (p-NP, 250 mg/kg/day, oral administraion) resulted in significant decreases of the weight and volume of testis, volume of seminiferous tubules and its diameter, thickness of the basement membrane, height of the germinal epithelium, total number of types A and B spermatogonia, spermatocyte, spermatid and Sertoli cells in rat (Mehranjani et al., 2009). Another study with Sertoli cells and serum reproductive hormones in prepubertal male rats, both in vitro and in vivo, showed that NP could disrupt the structure and function of Sertoli cells in vitro and hormone levels in serum even at low doses (Hu et al., 2014). The NP (60 mg/kg)-induced apoptosis, autophagy and necrosis in Sertoli cell seem to be through ROS-mediated pathway (Duan et al., 2016). Exposure of epididymal rat sperm to NP (250–500 ug/mL) significantly reduced motility, acrosomal integrity and acrosomal reaction (Uguz et al., 2009). Absolute testes and epididymides weights, sperm counts in cauda and caput/corpus epididymides, sperm motility, daily sperm production and sperm transit time in cauda epididymis were significantly decreased in rats gavaged with NP (100–300 mg/kg/day, for 30days) (Aly et al., 2012). Oral administration of NP (250 mg/kg/day for 90days) may damage the structure and function of testis, induce apoptosis and oxidative stress in epididymis or even have cytotoxic effects on epididymal sperm (Lu et al., 2014). In the present study, we found that the decreases of absolute weights in the accessory sex organs and the histopathological changes in testis and epididymis of low-dose NP treated mice, shown good agreement with the detrimental effects of NP exposure in previous reports. In particular, NP exposure with very low dose which is reflecting human daily intake level could elicit similar detrimental effects in the previous studies mostly adopted high-dose models. The multiple ‘sloughing morphology’, defined as a separation of at least one germ cell layer from the seminiferous epithelium, was observed in our testis sections from NP-treated animals, indicating the impaired spermatogenesis. Also, apparent reduction in sperm number from NP-treated epididymis in the present study could be closely related to the sperm count decrease in NP-treated animals (Chitra et al., 2002; Uguz et al., 2009; Aly et al., 2012).
NP and its metabolites are found in several human tissues and biological fluids such as adipose tissues, liver, amniotic fluid, breast milk, urine and semen (Muncke, 2009; Huang et al., 2016; Acir & Guenther, 2018). For example, the maximum NP concentration in adipose tissue was 85 ng/g lipids (Muller et al., 1998). Since the presence of NPs in human tissues was evident, it is certainly plausible that human exposure to NP could induce reproductive toxicity as found in rodent studies. However, to obtain practical information on the adverse effect of NP on human physiology, low-dose exposure issue must be carefully considered. The initially proposed no-observed-adverse-effect level (NOAEL) of NP in rat was 50 mg/kg/day (Cunny et al., 1999; Fourie et al., 2001). Recently, using mathematical models, the NOAEL of NP was revised to 15 mg/kg/day (Mao et al., 2019). Therefore, doses higher than NOAEL have been used in mostly all of laboratory animal researches, and such high-dose exposure is not likely to happen in our everyday lives. Based on an analysis of 60 different foodstuffs and food consumption rates in Germany, the average daily human intake of NP was calculated to be 7.5 ug/day, and for infants exclusively fed with breast milk or infant formulas, daily intakes are estimated to 0.2 and 1.4 ug/day, respectively (Guenther et al., 2002). In the present study, estimated daily intake of NP was 18.5–19.8 ug/kg/day when body weight was about 30 g. This level was about 1,000 times lower than currently accepted NOAEL for rat, and we thought our low-dose NP exposure model was much closer to the daily human intake.
Low-dose effects were defined by the National Toxicology Program (NTP) as those that occur in the range of human exposures or effects observed at doses below those used for traditional toxicological studies (Vandenberg et al., 2012). Actually, there is growing evidence to support low-dose effects of NP (Melnick et a., 2002; Di et al., 2018; Patino-Garcia et al., 2018; Yu et al., 2018). Nonmonotonic dose-response curves, a nonlinear relationship between dose and effect where the slope of the curve changes sign somewhere within the range of doses examined, is best explanation for the low-dose (i.e. nanomolar concentration) effects of EDCs including NP so far (Vandenberg et al., 2012).
The present study demonstrated that exposure to subchronic low-dose NP induced abnormality of reproductive organs in male F1 mice. Since the NP dose used in this study is closely related to the average human daily NP exposure, our results could have profound meaning, a serious alert on the male infertility (de Jager et al., 1999).