INTRODUCTION
It has been suggested that endocrine disrupting chemicals (EDCs) mimic the steroid hormone and/or inhibit the action of steroid hormones or endocrine system through classical and nonclassical pathways of steroid hormone as well as receptor-independent mechanisms (Keith, 1997; Krimsky, 2000; Bandiera, 2006; USEPA, 2017). Most of the EDCs are derived from synthetic organic chemicals and the other are from nature such as plants. The EDCs have continuously increased through the development of organic chemical synthetic skills and has threated human health and wild life (Blair et al., 2000; Browne et al., 2018). It has been revealed that ECDs can effect developmental malformations, interfere reproduction, increase cancer risks, and disturb the function of the immune and nervous system (USEPA, 2017).
Recently we reported that chronic low-dose di-(2-ethylhexyl) phthatlate (DEHP) and nonylphenol (NP) has nonmonotonic estrogenic effects in mouse reproduction (Cha et al., 2017, 2018). The last few decades, most of the studies on EDCs have been based on the pharmacological dogma, that EDCs dose are poisonous (Vandenberg et al., 2012) and toxic (Vandenberg et al., 2012; Mersha et al., 2018; van der Weijden et al., 2018). Nowadays, EDCs are challenged in the view of physiological responses and reproductive tract disorders concerned with dose exposure and periods (Huang & Li, 2014; Mersha et al., 2018; van der Weijden et al., 2018). For example, low-dose expose studies have been done in behavior, Sobolewski et al. 2014; Rebuli et al., 2015), obesity (Brulport et al., 2017), metabolism (Casals-Casas & Desvergne, 2011), and others (Casals-Casas & Desvergne, 2011; Zama & Uzumcu, 2013).
NP is derived from nonylphenol ethoxylates (NPEs), which are the metabolites of alkylphenol ethoxylates. NPEs are widely used in detergents, emulsifiers, pesticides, paints and plastics, and are also found in sewage (Purdom et al., 1994; Soares et al., 2008). Soto et al. (1991) and Shelby et al. (1996) showed that a high-dose NP had uterotrophic effects on rodents, and induced transcription in ER-transfected HeLa cells and MCF7 cells. Administration with 40 mg/kg/day NP for 14 days on guinea pigs exhibits a prevention of decrease in uterine weight following castration. The estrogenic effects on histological features of these animals like those occurring in normal cycling animals (Danzo et al., 2002). In ovariectomized rats, NP at a dose of 100 and 200 mg/kg for 3 days increased uterine weights, thickened of luminal epithelum, endometrium, and myometrium; and induced proliferation in endometrium (Zhang et al., 2007). Additionally, a low-dos NP also showed estrogenic effect in female mice (Cha et al., 2017; Di et al., 2018).
DEHP, which is also known as an estrogenic EDC is widely used as plasticizers in manufacturing polyvinyl chloride, and as it is not covalently bound to plastic, it is readily emitted to the environment (Lorz et al., 2007; Halden, 2010). DEHP is found in food wraps, medical devices, and cosmetic products. In rodents, DEHP is metabolized to mono 2-ethyl hexyl phthalate (MEHP) and 2-ethylhexanol in digestive tracts, and one of its metabolite, MEHP is observed to have more toxic effects (Grande et al., 2006; Somasundaram et al., 2016). It has been verified that DEHP has reproductive toxicity in humans and animals (Lovekamp-Swan & Davis, 2003; Lorz et al., 2007). In vivo exposure to 2 g/kg DEHP on mature Sprague-Dawley rats decreased ovarian estradiol production, prolonged estrous cycles, and stopped ovulation (Davis et al., 1994). Oral exposure to 1, 10, and 100 mg/kg body weight (BW)/day of DEHP on Wistar rats for 30 days did not change BW and wet uterine weight; but increased ovarian hormones and their receptors expression; and decreased uterine diameter and numbers of uterine glands (Somasundaram et al., 2016). In addition, it has also been recently revealed that low-dose DEHP has estrogenic effects in female (Cha et al., 2018; Julien et al., 2018).
Although the possible estrogenic effects of low-dose NP and DEHP have been suggested, the uterine responsibility to chronic low-dose NP and DEHP is not clearly evaluated. The present study performed to comparatively investigate whether chronic low-dose have estrogen-like activity on mouse uterus between NP and DEHP.
MATERIALS AND METHODS
All experimental animals were conducted in accordance with the Guide for the Care and Use of Laboratory Animals published by the National Institute of Health. CD-1 mice were maintained under standard condition at Sungshin University. Circadian rhythm kept under the 14L:10D schedule with light-on at 06:00 and clean room system. Animals were fed food and drink mentioned in Cha et al. (2017). Briefly, estrogen-free rodent diet (2018 Teklad global 18% protein rodent diets; ENVIGO, Madison, WI, USA) and water were provided ad libitum in glass bottles with stainless steel sipper tubes from weaning 21 days after birth.
Administration of NP and DEHP followed OECD Guidelines for the Testing of Chemicals–Test No. 443. 10–12-week-old animals were given NP at a dose of 50 or 500 μg/L or DEHP at a dose of 133 or 1,330 μg/L dissolved in drinking water for 10 weeks, considering that a mouse drinks approximately 4–7 mL/day water. Control group was given water without test substances. Estrous cycle was checked by smearing daily for first 2 weeks, then each normal cycling CD-1 female was chosen and bred with a male for 2 weeks (n=10 / each group). Copulatory plug was checked daily, and after 6 weeks euthanized by cervical dislocation.
To get ovariectomized mice, 2–3-month-old female mice were anesthetized with pentobarbital sodium (78 mg/kg BW) and removed ovaries. After a week, injected subcutaneously (sc.) with 0.1 mL vehicle (control) or 2 μg/kg E2 (Cat #: E8875, Sigma) in cottonseed oil for 3 days at 09:00 and sacrificed on the next day.
Uteri were dissected and the uterine wet weights were measured with analytical electron balancer. A part of uterus was stored at −80° until use for molecular experiments and the others were fixed overnight in Bouin's fixative. Fixed uteri were dehydrated with ethyl alcohol using Leica TP 1020, and embedded in paraffin. The paraffin block was cross-sectioned at 4 μm (Leica RM2245 microtome) and stained with hematoxylin and eosin Y. Tissues are microphotographed using Olympus B×60 microscope and Olympus DP71 microscope digital camera. Uterine diameter was measured perpendicularly to mesometrium-antimesometrium axis on 40× microphotograph with ImageJ program. Myometrium and endometrium thickness were respectively measured from longitudinal to circular smooth muscle layers and from luminal surface to beginning of circular smooth muscle layer on 100× microphotograph with ImageJ software. Epithelial cell height was measured on 400× magnification microscope with Tcapture software. Furthermore, number of endometrial glands was counted and its morphology was analyzed. To get confidence of the data, at least 4 sections per mouse were analyzed and in all directions.
Total RNA was extracted using TRI reagent (Cat #: TR 118, Molecular Research Center, Cincinnati, OH, USA) according to manufacturer's instruction with modification. Briefly, the sample was homogenized with TRI reagent (1 mL/100 mg) and kept for 10 min at room temperature (RT). The chloroform (200 μL/mL) was added and shaken vigorously for 15 sec. Then the mixture was stored for 15 min at RT and centrifuged at 12,000 g for 15 min at 4°. The RNA was precipitated by mixing isopropanol (0.5 mL/mL), inverting several times, maintaining at RT for 10 min, and centrifuging at 12,000 g for 8 min at 4°. Purity and concentration of total RNA were assessed by NanoDrop 2000 Spectrophotometer (Cat #: ND-2000, Thermo Scientific, Wilmington, DE, USA). The total RNA was stored at −80° until used.
First strand cDNA was synthesized using AccuScript High Fidelity Reverse Transcriptase (Cat #: 600089, Agilent Technologies, CA, USA) according to the manufacturer's instruction. Shortly, reaction reagents were total RNA, AccuScript 10× RT buffer 5.0 μL, 0.5 μg/μL oligo dT primer 1.0 μL, 0.1 μg/μL random primers 1.0 μL, 100mM dNTP mix 2μL, and RNase-free DEPC-treated water. Reaction mixture was incubated at 65° for 5 min, and cooled slowly at RT to allow the primers to anneal to RNA for 10 min. Next, 100 mM DTT 4.0 μL, 40 U/μL RNase block ribonuclease inhibitor 2.0 μL (Cat #: 300152-51, Agilent Technologies, CA, USA), and AccuScript High Fidelity RT 1.0 μL were added. The mixture was incubated for at 42° for 1 hr and at 70° for 10 min to terminate cDNA synthesis. cDNA was stored at −20°.
Real-time PCR was performed using SYBR® Premix Ex TaqTM (Cat #: RR420A, TaKaRa, Japan) and Thermal Cycler Dice Real Time System TP800 (TaKaRa, Japan). Each reaction was run in triplicate. Dissociation curves were run on all reactions to ensure amplification of a single product with the appropriate melting temperature. The fold change in gene expression was calculated using the ΔΔCt method with housekeeping gene, 36B4, as an internal control.
RESULTS
The wet weight was 0.206±0.019 g in control, 0.177± 0.018 g in 50 μg/L NP, 0.208±0.001 g in 500 μg/L NP, 0.289± 0.157 g in 133 μg/L DEHP, and 0.175±0.003 g in 1,330 μg/L DEHP. The ration of uterus wet weight to BW (0.0078±0.00119) was significantly high in 133 μg/L DEHP 133 treated mice (Fig. 1). The rations were 0.00548± 0.00047 in control, 0.00478±0.00054 in 50 μg/L NP, 0.00569± 0.00077 in 500 μg/L NP, and 0.00474±0.00030 in 1,330 μg/L (Fig. 1).
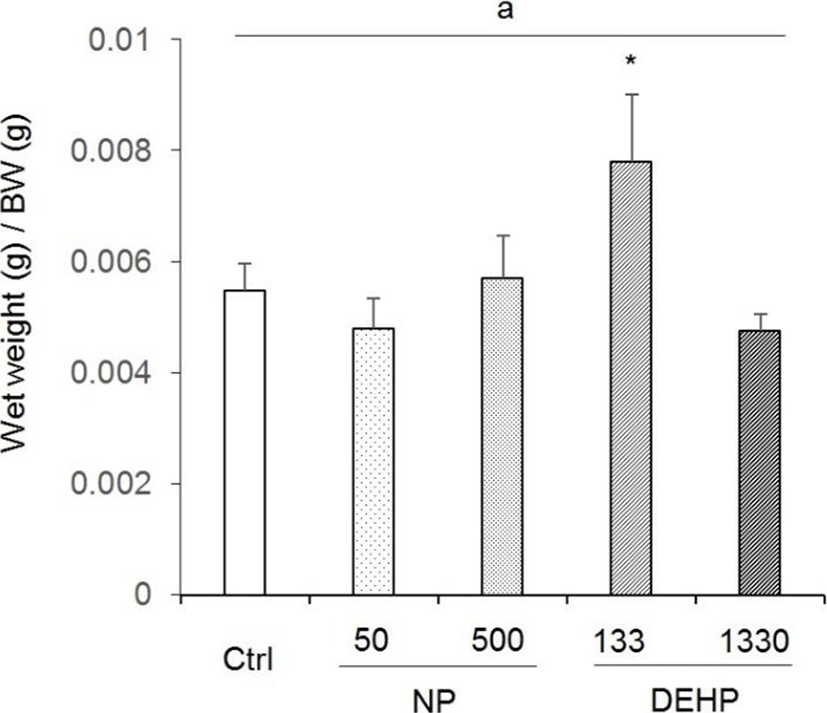
There were no statistical significance between control and EDCs treated groups in uterine diameter. However, the diameter tended to increase in 133 μg/L DEHP. Compared with the NP treated groups, the diameter in 133 μg/L DEHP significantly increased (Fig. 2).
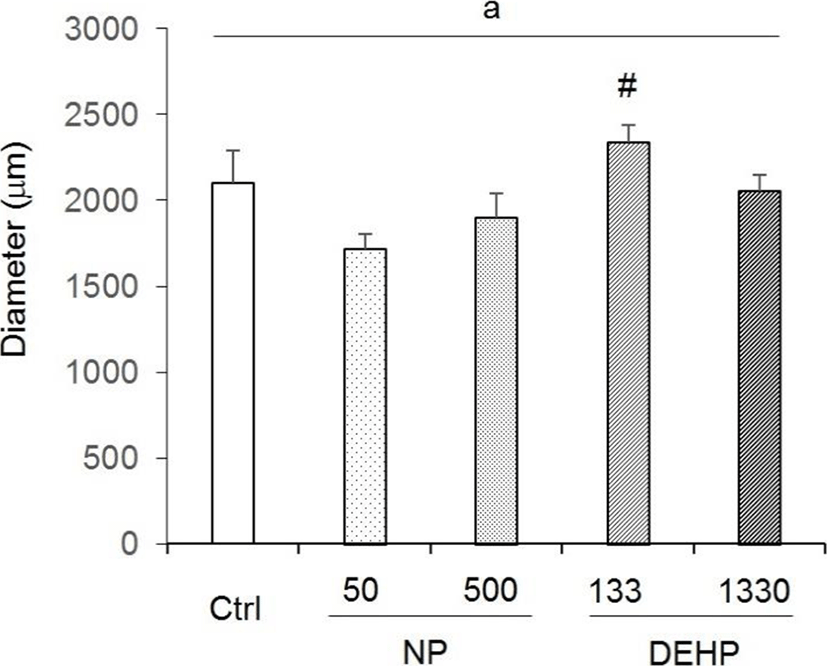
Myometrium thickness significantly increased by 50 μg/L NP, 500 μg/L NP, and 133 μg/L DEHP groups (Fig. 3). On the other hand, the thickness of endometrium was increased only by DEHP treated groups (Fig. 3).
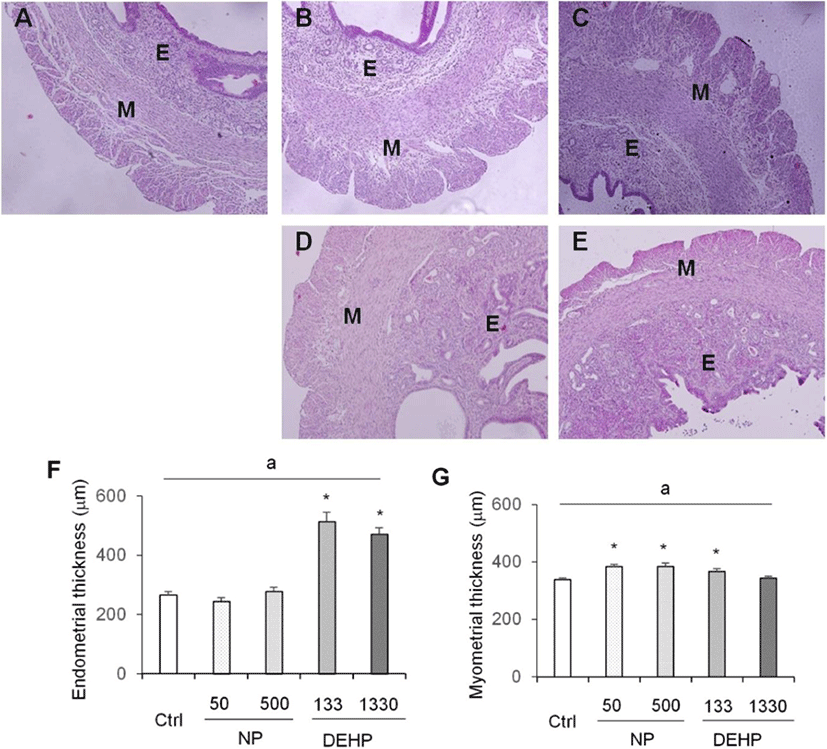
In the height of luminal epithelial cells, the effects of NP and DEHP were also different by EDCs. The height was significantly shorter in NP treated groups than the control. However, there was no difference of the epithelial height in DEHP treated groups compared with the control (Fig. 4). The number of endometrial glands were less in NP treated groups than the control and DEHP treated groups. In 133 μg/L DEHP treated group, the number of glands significantly increased (Fig. 5F). There were no difference in morphology of the glands between treatment groups. All of the groups including the control had normal, daughter, conglomerated, and few cystic glands (Fig. 5A–E).
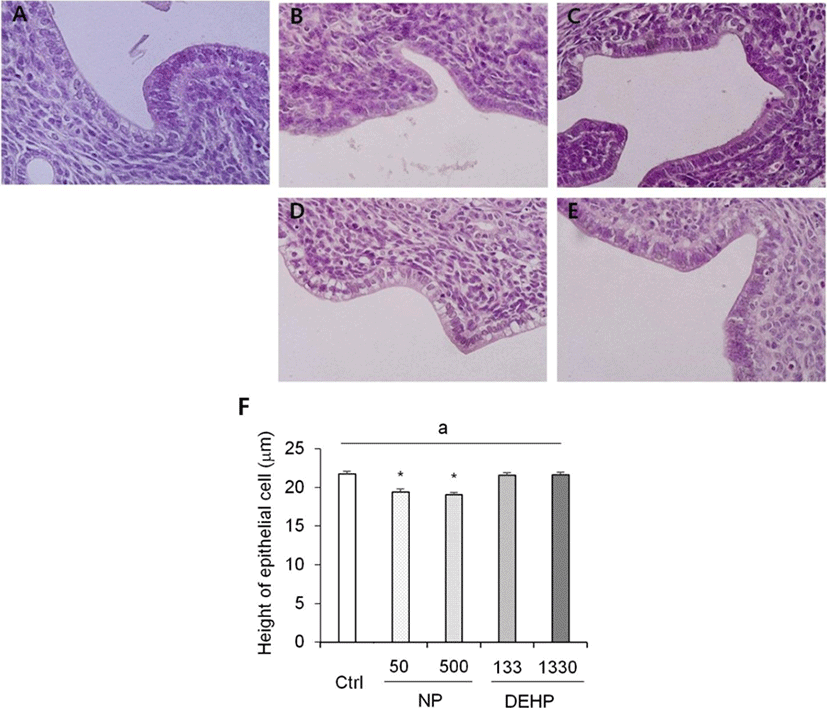
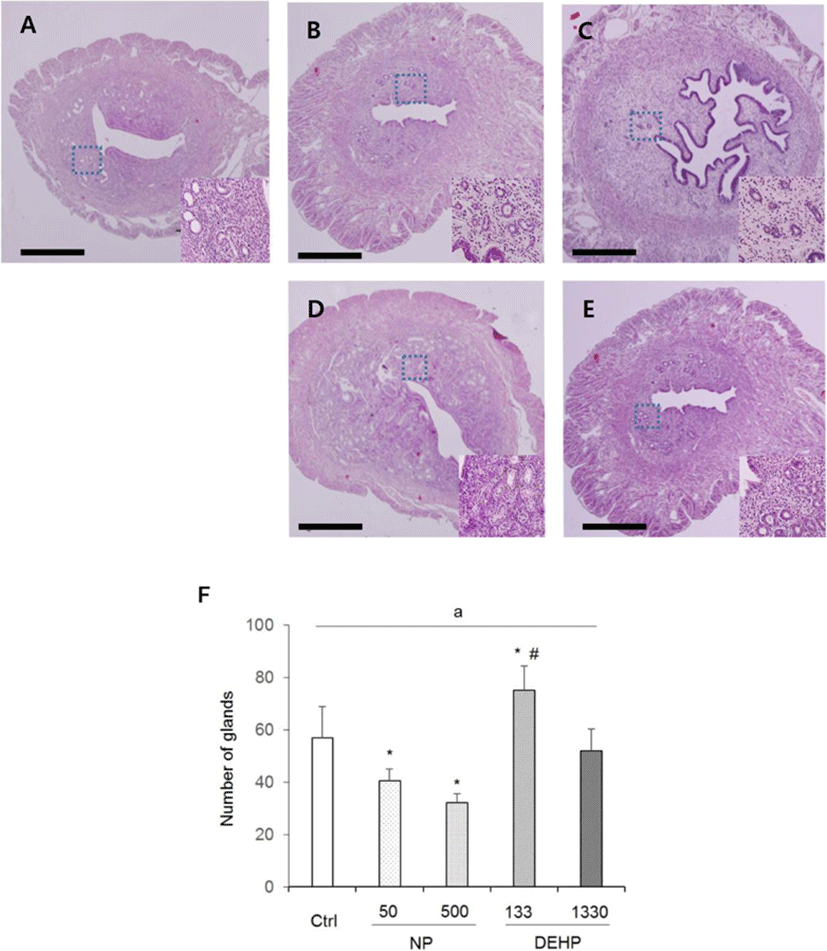
Esr1, Esr2, Prg, Lox, Egr1 and Muc1 are well known E2 downstream genes. As expected the expression levels of Esr1, Pgr, Lox and Muc1 mRNAs increase by administration E2 for 3 days as mentioned in materials and methods. Yet, Esr2 and Egr1 mRNAs did not increased (Fig. 6). Expression levels of Esr1 and Lox mRNAs were significantly higher in 500 μg/L NP, but were significantly lower in DEHP treated groups than the control (vehicle) and E2 treated group (Fig. 6A,D). In the case of Esr2, its expression levels were significantly higher in 50 μg/L NP, but were significantly lower in DEHP treated groups than the vehicle and E2 treated group (Fig. 6B). Pgr mRNA expression significantly decreased in 500 μg/L NP treatment compared with the control. The expressed mRNA levels were significantly lower in NP and DEHP treated groups than the E2 treated group (Fig. 6C). The expression levels of Muc1 mRNA significantly decreased in NP treated groups, but significantly increased in DEHP treated groups compared to the vehicle. Muc1 expression was significantly lower in NP treated groups than the E2 treated group. However, its expression was significantly higher in 133 μg/L DEHP treated group than the E2 treated group (Fig. 6E). In the case of Egr1 gene, its expression levels were not different between groups (Fig. 6F) as expected.
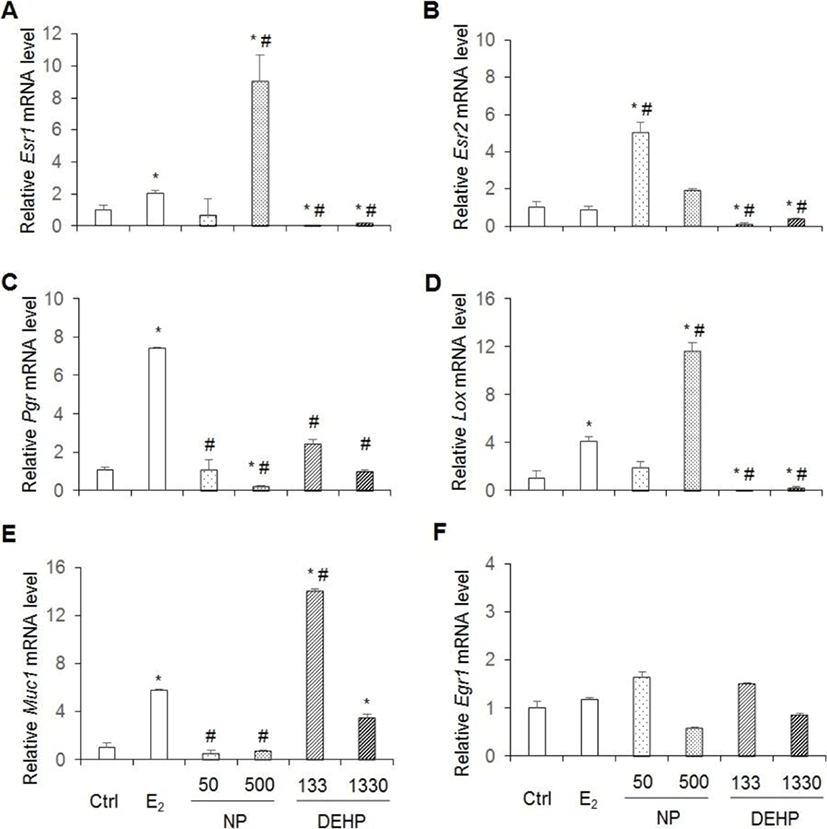
DISCUSSION
E2 (40 ng/day in mice) is known to have uterine hypertrophic effects, as it increases uterine weight, diameter, endometrium thickness and myometrium thickness, which corresponds to proliferation on endometrial, myometrial and epithelial cells as well as hypertrophy of uterine epithelial cells (Papaconstantinou et al., 2000). Previously we reported the estrogenic effects of chronic low-dose NP and DEHP in the reproduction of mouse (Cha et al., 2017; Cha et al., 2018). Interestingly chronic low-dose NP and DEHP showed the estrogenic uterine response as seen in the results.
Chronical exposure with low-dose NP, did not induce uterine weight gain nor did it increase the diameter. The endometrial thickness did not increase, but the myometrial thickness increased by 50 and 500 μg/L NP treatment. The height of luminal epithelial cells and the number of luminal epithelial glands were smaller than the control both in 50 and 500 μg/L NP. This shows that the chronic low-dose NP did not induce uterine weight gain, but stimulated the epithelial cells. Previously, it has been suggested that ERα-specific agonist induces uterine weight increase but ERβ-specific agonist does not (Frasor et al., 2003). ERα is mainly expressed in the uterine epithelium, and causes proliferation of epithelial cells based on the inter-regulation with stromal ERs (Somasundaram et al., 2016). ERβ modulates the effects of ERα and suppresses the endometrial proliferation and uterotrophic effects (Weihua et al., 2000; Somasundaram et al., 2016). Therefore, it may be suggested that this examined dose of NP in the present research did not stimulate ERα in stroma cells and others, but stimulate ERβ. In contrast, the previous suggestion of NP's role (100 or 200 mg/kg BW) in uterus as hyperplasia factor (Zhang et al., 2007) is denied in the case of chronic low-dose treatment.
In the case of DEHP, 133 μg/L DEHP induced the gain of uterine weight, and increased the diameter of uterus. Endometrial thickness increased by the both 133 and 1,330 μg/L DEHP. Myometrial thickness increased by 133 μg/L DEHP. Additionally, the number of glands increased by 133 μg/L DEHP. These results indicate that DEHP has nonmonotonic effects in uterus. Recently, it has been reported that DEHP induced leiomyoma cells to have higher viability and lower apoptosis rate (Kim, 2018). In vitro treatment with DEHP has suggested increased viability of endometrial stromal cells, a precondition to endometriosis (Scsukova et al., 2016). 30 days exposure of 200 μg/kg/day DEHP on uterus of adult female CD-1 mice reduced epithelial cell proliferation and increased the number of uterine glands. Proliferation of endometrial stromal cells increased at 200 μg/kg/day, 20 mg/kg/day and 200 mg/kg/day DEHP (Richardson et al., 2018). Besides, it is known that DEHP bind to ERα but not ERβ (Satoh et al., 2001; Takeuchi et al., 2005). Combining with the results of Frasor and Colleagues (2003), low-dose DEHP (133 μg/L) can work with ERα in uterus.
In all groups, cystic, daughter and conglomerated glands were observed. It has been reported that 25-day-old prepubertal mice that were injected 0.1 mL oil as vehicle for 20 consecutive days did not exhibit daughter and conglomerated glands (Elia et al., 2008). Another study demonstrated that all those types of glands were observed in ovariectomized mice that were injected with 20 μg/kg BW/week E2 for 30–90 (Gunin et al., 2001). The mice used in the current study were not ovariectomized. Hence, this may reflect the estrogenic action on endometrial glands of the control group as well as the experimental groups. Collectively, these histological features indicate weak potential estrogenic effects of chronic small dose exposure to the chemicals on mouse uterus.
Pgr is expressed through estradiol-induced ERα action (Somasundaram et al., 2016). The p-nonylphenol extracted from modified polystyrene induce cell proliferation with up-regulation of Pgr expression in MCF7 cells (Soto et al., 1991). At dose of 1, 10, and 100 mg/kg BW/day DEHP for 30 days, Pgr mRNA expression decreased (Somasundaram et al., 2016). However, interestingly, our results were controversy to the previous study: Chronic exposure of low-dose NP or DEHP did not induce higher expression of Pgr mRNA. 500 μg/L NP suppressed the expression of Pgr mRNA. On the other hand, in the case of Esr1 and Lox genes, chronic 500 μg/L NP administration significantly induced higher expression of Esr1 and Lox mRNAs expression, but DEHPs suppressed their expression than vehicle and E2 treatment group. It is known that Esr2 gene expression is downregulated by E2. Interestingly, Esr2 mRNA expression increased by 50 μg/L NP administration, and down regulated by chronic low-dose DEHPs. Therefore, the expression of Esr1, Lox, and Esr2 were similar on agonistic regulation by chronic low-dose NP and antagonist regulation by chronic low-dose DEHP.
Egr1 is rapidly and transiently up-regulated in uterus by E2 (Guo et al., 2014). As expected in this model, Egr1 mRNA levels did not changed. In the case of Muc1 gene, its expression is suppressed by chronic low-dose NP administration, but induced by chronic low-dose DEHP administration as in dose-dependent manner. Therefore, the expression of Muc1 was similar on agonistic regulation by chronic low-dose DEHP and antagonist regulation by chronic low-dose NP.
One of the characteristics of estrogen is biphasic physiological process, likes in gonadotropin secretion feedback (Herbison, 2008). The chronic low-dose NP and DEHP have dosage dependent gene expression response in uterus that may be the results of affinity to the ERs, though the effects of EDCs can be appeared by nuclear receptor, nonclassical receptor, and receptor-independent mechanisms. ERs are expressed in all cell types of uterus; ERα is mainly expressed in luminal epithelium and stroma; and ERβ is localized in myometrium and stroma (Weihua et al., 2000; Cunha et al., 2004; Wada-Hiraike et al., 2006). In addition, ERα and ERβ colocalize in the nuclei of stroma and in the epithelial cells of gland under the control of E2 and progesterone. Kuiper and his colleagues (1998) suggested that some phytoestrogen such as genistein, kaempferol. apigenin, and kaempferol have a bigger affinity to ERβ than ERα. Binding affinity of NP to the ERα and ERβ is 0.05 and 0.09, respectively (Kuiper et al., 1998). NP has bigger affinity than the others (octylphenol, heptyloxyphenol, etc) and affinity IC50 is 2.40–4.73×10–6. The IC50 of E2 is 8.99×10–10 (Blair et al., 2000). On the other hand, DEHP has very weak competition for the ER and 78% [3H]-E2 bind to ER with IC50 is >1.00×10–3. Furthermore, ERα and ERβ have opposite effects on gene transcription (Paech et al., 1997). To uncover the possible physiological role of chronic-low dose NP and DEHP, further studies in the level of genetic information usage is needed. This study clearly suggests that chronic lower-dose administration of NP and DEHP are still potential to have estrogen like roles in uterus as EDCs. It also shows that chronic low-dose NP has nonmonotonic effects in uterus.