INTRODUCTION
Cryopreservation of reproductive cells and tissues is one of the most important parts of biomedical and agricultural researches, which can be used to overcome an infertility of human beings and to preserve valuable genetic resources of endangered and transgenic animals. One of the powerful methods to cryopreserve reproductive cells with decreased cryoinjuries is ultra-rapid freezing method such as vitrification. Recently, a vitrification method has been applied widely to cryopreserve the cells in the mammalian species, including pig (Isachenko et al., 1998), cattle (Fuku et al., 1995), mice (Kono et al., 1991), and human (Boyer et al., 2012). Especially in pig oocyte, it is well known that the oocytes matured in vitro are very difficult to cryopreserve because of its higher sensitivity to cryoinjuries compared to other mammalian oocytes like human (Fabbri et al., 1998), cattle (Hwang et al., 2013), and small rodent (dela Pena et al., 2001).
According to our previous report, lipid content, quality, size, and shape of oocytes were responsible for cryotolerance (Hwang & Hochi, 2014). In pig, limited studies have been demonstrated a successful vitrification using immature and in vitro matured oocytes (Rojas et al., 2004; Albarracin et al., 2005). Vitrification of in vitro-matured oocytes might be occur various kinds of cryoinjuries caused by high concentration of cryoprotectant (CPA) in the vitrification medium (Hwang & Hochi, 2014). Exposure of oocyte to CPA has been shown to induce cryoinjuries such as microtubule depolymerization and premature exocytosis of cortical granules resulting decreased survival rates and developmental failure (Coticchio et al., 2009; Lowther et al., 2009). The CPA exposed oocytes during vitrification had been changed not only distribution and function of mitochondria but also mitochondrial ultrastructure (Vallorani et al., 2012; Dai et al., 2015). An abnormal distribution and dysfunction of mitochondria in oocytes were closely involved in the activation of apoptotic mechanisms resulting oocyte dead (Vallorani et al., 2012). Therefore, vitrification method for pig oocyte is still required to be improved much in survival, fertilization, and developmental rates (Mullen & Fahy, 2012; Somfai et al., 2012).
To improve survival rates of vitrified oocytes, it is essentially needed a modification of vitrification procedures, development of vitrification devices, and treatment of chemicals during a vitrification procedure. Many chemicals were applied during recovery culture such as signal pathway inhibitors, antioxidants, and cytoskeletal stabilizers (Hwang et al., 2013; Hwang et al., 2016; Pitchayapipatkul et al., 2017). One of the common cytoskeletal stabilizers is cytochalasin B (CB) widely applied for softening of oocyte before conducting micromanipulation such as somatic cell nuclear transfer because of its safety on oocytes (Hwang et al., 2015). Chemical treatments during post-warming culture after vitrification can be one of the possible approaches to help oocyte to regain its ability to develop. Therefore, the present study was conducted to determine optimal treatment timing of CB during vitrification and warming procedures.
MATERIALS & METHODS
In experiment 1, the survival rates of the post-warming pig oocytes were analyzed by fluorescein diacetate (FDA) assays with 4 classifications as shown in Fig. 1. After vitrification/warming and recovery culture, the pig oocytes were stained with FDA and analyzed its survival rates. The oocytes with strong and intermediate green fluorescence were determined as alive, but weak and none signals were classified as dead. In experiment 2, CB was treated before (CB-Vitri) and after (Vitri-CB) vitrification. Then, the survival rates of post-warming oocytes were analyzed by morphology and FDA assay to determine an appropriate timing and effect of CB treatment.
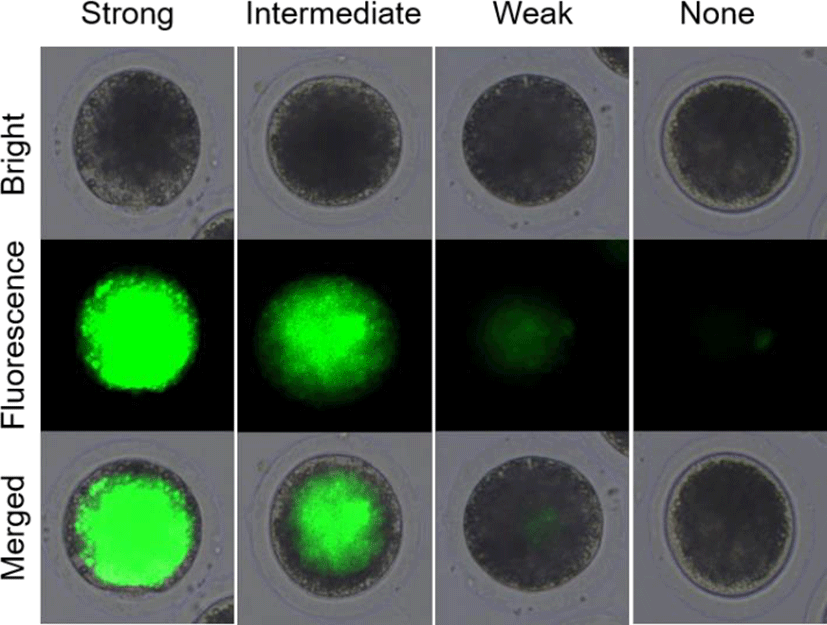
All chemicals were purchased from Sigma-Aldrich Chemicals (St. Louis, MO, USA) unless stated otherwise. Prepubertal pig ovaries were obtained from local slaughterhouse Nonghyup Moguchon, Gimje, Korea) and transported to the laboratory within thermos containing 30℃ to 35℃ of saline. Then, 3–6 mm follicles were aspirated by disposable syringe with an 18 gauge needle and cumulus oocytes complexes (COCs) with several compact layer of cumulus cells were selected for in vitro maturation. The COCs were cultured in the hepes-buffered Tissue Culture Medium (TCM)-199 (Gibco BRL, NY, USA) supplemented with 10% porcine follicular fluid (pFF), 3.05 mM D-glucose, 0.57 mM cysteine, 0.91 mM sodium pyruvate, 0.5 μg/mL FSH, 0.5 μg/mL LH, 10 ng/mL EGF, 75 μg/mL penicillin G and 50 μg/mL streptomycin in a four-well dish for 22 h at 38.5℃ under 5% CO2 in air. Then the oocytes were matured in vitro within the maturation medium described above without FSH and LH for additional 22 h at 38.5℃ under 5% CO2 in air. After in vitro maturation, the oocytes were examined comprehensively for the extrusion of the first polar body after removal of cumulus cells by treatment of 0.1% hyaluronidase solution with gentle pipetting.
The matured oocytes were conducted to a vitrification and warming according to the method described previously (Hwang et al., 2013). Briefly, the oocytes were equilibrated in the base medium [TCM-199 with 20% fetal bovine serum (FBS, Gibco BRL)], supplemented with 7.5% ethylene glycol (EG), and 7.5% dimethyl sulfoxide (DMSO) for 5 min. Then, the equilibrated oocytes were transferred into the vitrification solution consisting of 20% FBS, 15% EG, 15% DMSO, and 0.5 M sucrose for 1 min at room temperature. During that time, around 15 oocytes were mounted onto the tip of the Cryotop (Kitazato BioPharma, Shizuoka, Japan) with a minimal volume of the vitrification solution (< 0.1 μL) and then quickly plunged into liquid nitrogen (LN2). After storage for more than 1 week in the LN2, the vitrified oocytes were warmed by soaking the tip of the Cryotop into the pre-warmed base medium containing 1 M sucrose at 38.5℃ for 1 min. Then, the oocytes were transferred into the base medium containing 0.5, 0.25, and 0 M sucrose (for 3, 5, and 5 min, respectively) at room temperature (25.0℃). The predicted freezing and warming rates of the Cryotop method informed by the manufacturer were -23,000 and 42,000℃ /min, respectively.
After vitrification and warming, the oocytes were washed 3 times and conducted to recovery culture with TCM-199 with 5% FBS, 0.2 mM sodium pyruvate, 75 μg/mL penicillin G, and 50 μg/mL streptomycin in a 4-well dish for 2 h at 38.5℃ under 5% CO2 in air (30 oocytes per well). The CB was treated before (CB-Vitri) vitrification procedure for 30 minutes and after (Vitri-CB) vitrification procedure from start of recovery culture for 30 minutes with concentration of 7.5 μg/mL.
The survival of post-warming oocytes was evaluated based on our previous study (Hwang et al., 2013). Briefly, the survival was estimated by morphological changes on oolemma and zona pellucida firstly. Demarcation of membrane, zona pellucida fracture, and cytoplasmic distribution were comprehensively checked by microscope. Then, the survival rates based on esterase enzyme activity by FDA assay was assessed. After recovery culture with or without CB, the oocytes were washed 3 times in PBS for 1 min, followed by incubation with 2.5 mg/mL of FDA for 1 min. Then, the oocytes were washed 3 times again in PBS containing 0.1% BSA and observed under an epifluorescence microscope (Leica Microsystems, Wetzlar, Germany). As shown in Fig. 1, the alive oocytes showed strong and intermediate green fluorescence, whereas the dead oocytes showed a weak or none fluorescent signals.
RESULTS
According to the results indicated in the Table 1, the post-warming oocytes showed significantly decreased the number of the alive compared to the fresh control (31.8% vs. 86.4%) (p<0.05). In detail, the significant difference (p<0.05) was found only in strong fluorescence (18.2% vs. 70.5%), but not in intermediate fluorescence groups (13.6% vs. 15.9%). On the other hand, the number of the dead oocytes were significantly increased (68.2% vs. 13.6%) compared to fresh control (p<0.05). The rates of the dead oocytes in both weak (40.9% vs. 13.6%) and none (27.3% vs. 0.0%) fluorescence groups were significantly different compared to the fresh control (p<0.05), respectively.
FDA, fluorescein diacetate.
Pig oocytes were treated with CB before and after vitrification procedures to determine appropriate treatment timing for the improvement of survival rates in the post-worming oocytes. After recovery culture, all the vitrified oocytes were subjected to test its viability by morphological inspection and FDA assay. As shown in Table 2, the Vitri-CB group showed significantly higher survival rates (91.6% vs. 83.7%) in morphological inspection compared to the CB-Vitri group (p<0.05), respectively, but lower than that of the Fresh Control (100.0%) (p<0.05). Interestingly, the CD-Vitri group showed comparably lower survival rate than that of Vitri-Control (88.7%). According to the results of FDA assay, the Vitri-CB group showed significantly higher (44.2%) survival rates compared to CB-Vitri (36.7%) and Vitri-Control (35.1%) groups (p<0.05), respectively.
FDA, fluorescein diacetate; Vitri-Control, vitrification only; CB-Vitri, CB treatment before vitrification/warming; Vitri-CB, CB treatment after vitrification/warming.
DISCUSSION
Morphological inspection of an oocyte quality and viability by assessment of morphological changes on oolemma and zona pellucida is widely used to select good quality oocytes and embryos (Van Soom et al., 2003; Hamamah, 2005). To determine the viability and quality of oocyte, demarcation of membrane, perivitelline space, zona pellucida fracture, and cytoplasmic distribution were firstly needed to be checked before conducting the next step of experiment associated with assisted reproductive technology (Wang & Sun, 2006; Balaban et al., 2012). However, the result of morphological assessment does not reflect always to fertilization rates and oocyte/embryo quality after conducting assisted reproductive technology (De Sutter et al., 1996; Balaban et al., 1998; Hwang et al., 2016). Previous study showed that high survival rates by morphological inspection did not reflect high quality of oocyte/embryo and developmental competence (Hwang et al., 2016).
On the other hand, the FDA assay has been widely applied to determine the viability and quality of embryos (Mohr & Trounson, 1980; Noto et al., 1991) and oocytes (Didion et al., 1990; Hwang et al., 2016). Because many lipid droplets were in cytoplasm of pig and cattle oocytes, the oocytes were not visible inside by microscope (Kikuchi et al., 2002). Therefore, the FDA assay to determine survival rates and to compare the difference with survival rates by morphological inspection of post-warming pig oocytes.
In the present study, the survival rate of the post-worming oocytes was different between morphological inspection and FDA assay. Although high rate of the vitrified oocytes looked like survive (83.7% to 91.6%) by morphological inspection, the survive rate of the oocytes was decreased (35.1% to 44.2%) by FDA assay. It can be postulated that the morphological inspection was not sufficient to assess viability, at least in the post-warming pig oocyte. To improve the accuracy of FDA assay result, it was subdivided into 4 classifications of strong, intermediate, weak, and none green fluorescence as shown in Fig. 1. As shown Table 1, the post-warming pig oocytes vitrified by conventional vitrification protocol showed significantly (p<0.05) decreased numbers of alive oocytes compared to the Fresh Control group like our previous study (Hwang et al., 2016). It could be understood that the decreased survival rates were caused by subdivided categorization of alive and dead oocytes. And this result could be indicated that the post-warming oocytes were well divided into alive and dead because only the oocyte having higher enzymatic activity (activation of fluorescence) and cell-membrane integrity (retention of fluorescent) can be detected strong and intermediate green fluorescence. In the present study, the increased number of weak fluorescence in the post-warming oocytes could be indicated that the oocytes lost enzymatic activity and cell-membrane integrity during vitrification procedures.
To overcome cryoinjuries occurred by vitrification procedures, many kinds of attempts have been applied directly or indirectly to the oocyte and embryo in many species (Hwang et al., 2013; Chasombat et al., 2015; Hwang et al., 2016). In the present study, it was focused on the physical damages of the oocytes during vitrification procedures. It is well known that the vitrification procedures could affect cytoskeletal damage such microtubule dysfunction, spindle disorder, and mitochondrial function (Hara et al., 2012; Almasi Turk & Roozbehi, 2013; Amoushahi et al., 2017). In the present study, we also tried to determine the appropriate timing for CB treatment either before or after vitrification procedure. Based on our results, the optimal timing of CB treatment was immediately after warming during recovery culture. The CB treatment before vitrification showed adverse effect on the survival rates of the post-warming oocytes similar with previous study (Marco-Jimenez et al., 2012). We concluded that this phenomenon might be related to shrinkage during vitrification procedure. The oocytes vitrified by CB might be shrunk easier than untreated oocyte because of its softened cytoskeletal followed by more difficult to swell up during warming.
In conclusion, the application of subdividing method is a very delicated method to determine the viability of post-warming pig oocyte. Also, the increased survival rates of post-warming pig oocyte treated with Vitri-CB method are firstly described here. The main finding is that short-term (30 min) treatment of the CB during recovery culture could be helpful to refresh the post-warming pig oocyte resulting improved survival rates.