INTRODUCTION
Because skin functions as a protective barrier against harmful environments, skin wounds must be repaired properly and efficiently. The wound healing process consists of complex, dynamic overlapping phases that include hemostasis, inflammation and tissue remodeling remodeling (Martin 1997; Eming et al., 2007; Guo & dipietro, 2010) Wound healing progression depends on well-organized coordination between the wound site and the surrounding environment, such as the immune system and endogenous stem cells, including both dermal sheath stem cells and mesenchymal stem cells (Jahoda & Reynolds, 2001; Gurtner et al., 2008; Wang et al., 2013). During the inflammation phase, macrophages and neutrophils infiltrate the wound area and produce cytokines to promote the healing process. During the tissue formation and remodeling phase, fibroblast migration and proliferation and the expression of extracellular matrix components contribute to the process of skin repair.
While stem cells are reported to facilitate skin wound healing by enhancing neovascularization (Choi et al., 2013) and promoting microvascular remodeling (Dulmovits & Herman, 2012), low cell survivability after transplantation to the wound site is a major limitation for the therapeutic application of stem cells (Modo et al., 2002). In addition, safety issues regarding the use of stem cells have not been completely resolved (Kucia et al., 2005). Because stem cell-conditioned medium (SC-CM) contains a multitude of trophic and survival signals, such as growth factors, chemokines and cytokines (Chen et al., 2009), using SC-CM in wound healing is an alternative approach to overcome the limitations of cell-based therapy. For example, paracrine factors secreted by adipose-derived stem cells (ADSCs) are reported to influence wound re-epithelization by promoting keratinocyte migration in vivo and by functioning as immunosuppressants (Chen et al., 2008; Park et al., 2008). Though, paracrines are known to be a beneficial effect of ADSCs, very little is known about the regulatory mechanism controlling the expression of ADSC paracrine factors.
Ell3 is a transcription elongation factor that belongs to the eleven-nineteen lysine-rich leukemia (ELL) family (Miller et al., 2000). In mouse embryonic stem cells, Ell3 is a super elongation complex component that mediates RNA polymerase II occupancy at enhancer of development- related genes, which primes future gene activation following differentiation induction signals (Lin et al., 2013). In estrogen receptor-positive (ER+) breast cancer cells, Ell3 forms a transcriptional complex with ER, GATA3 and FOXA1 that regulates the expression of interleukin-20 (IL-20), which leads to the stabilization of p53 and the chemosensitization of cancer cells upon treatment with cis-diamminedichloroplatinum (CDDP) (Ahn et al., 2015; Lee et al., 2017).
The molecular mechanism related to the tissue repair is still largely unknown. Recent investigations of wound healing mechanism revealed that wound healing process is involved with inflammatory response and there is a continual competition between pro-inflammatory and anti-inflammatory signals during the process (Eming et al., 2007; Eming et al., 2010). Because Ell3 regulates the expression of IL-20 that is reportedly related to epithelial wound healing (Kolumam et al., 2017; Zhang et al., 2017), we questioned whether the expression level of Ell3 is associated with the wound healing activity of the secretome of ASC by regulating other cytokines including pro-inflammatory cytokine, IL-1 and IL-6 (Slaats et al., 2016).
Therefore, we studied whether Ell3 regulates the efficiency of ADSC-CM to heal skin wounds in vitro and in vivo. We found that Ell3 suppression in ADSCs decreases the therapeutic efficacy of ADSC-CM with respect to dermal fibroblast migration and proliferation in vitro and wound healing activity in vivo. We report that Ell3 is related to the expression of VEGF and MMP family genes, which are prominent wound healing-related ADSC factors.
Materials and Methods
ADSCs (SEFO, Seoul, Korea) were grown in alpha-Minimum Essential Media (a-MEM, Gibco, MA, USA) supplemented with 10% fetal bovine serum (FBS) (Gibco) and 1% penicillin/streptomycin (Invitrogen, CA, USA). Mouse embryonic fibroblasts (MEF) and normal human dermal fibroblasts (NHDF) were grown in Dulbecco’s Modified Eagle’s Medium (DMEM, Gibco) supplemented with FBS and 1% penicillin/streptomycin. All cell lines were incubated at 37℃ and 5% CO2. Non-specific control siRNA (siNS, D-001810-10) and human Ell3 siRNA (siEll3, L-014601-02-0010) were purchased from Dharmacon, Inc. (IL, USA). For Ell3 knockdown, ADSCs were transfected with 100 nM siNS or 100 nM siEll3 using lipofectamine 3000 (Invitrogen,CA, USA), and the cells were harvested 48 hours after transfection for subsequent analyses.
Total RNA was extracted using TRIzol (Invitrogen,CA, USA), and 5 µg of total RNA was used to synthesize cDNA according to the manufacturer’s instructions. Quantitative gene expression analysis was performed using real-time RT-PCR with the primer pairs listed in Table 1.
Briefly, 24 hours after siRNA transfection, normal ADSC culture media was replaced with serum-free media (SFM a-MEM), and the cells were incubated for an additional 48 hours. The culture media was harvested and centrifuged at 1,000 rpm for 3 minutes, and the supernatant was collected and filtrated with a 0.45-µm syringe filter for the experiment.
Briefly, 4×104 MEF or NHDF were cultured in CM prepared from siNS- or siEll3-transfected ADSCs, and cell numbers were counted every 24 hours for 4 days using a hemocytometer to analyze the effects of CM on cell proliferation. All samples were assayed in triplicate, and the means for each experiment were calculated.
The effects of ADSC-CM on the migration potential of MEF and NHDF was assessed using the scratch/wound healing and transwell migration assays. For the scratch/wound healing assay, 1×105 MEF or NHDF were seeded onto 6-well plates, and mitomycin C (10 µg/mL) (Sigma Aldrich, MI, USA) was added for 2 hours when the cell densities reached ~90% to prevent cell proliferation. After the cells were washed with SFM, the monolayers were wounded using a micropipette tip. The cells were cultured in CM for 2 days, and the degree of cell wounding was measured every 24 hours using a microscope. For the transwell migration assay, 2×103 MEF or NHDF were seed onto the upper well of a transwell plate (6.5 mm diameter, 8 µm pores; Corning, NY, USA). The lower portion of the well contained CM derived from siNS- or siEll3-transfected ADSCs. After incubation for 24 h, the cells were fixed in 4% paraformaldehyde (Santa Cruz, CA, USA) and stained with crystal violet (Sigma Aldrich).
Balb/c mice (8-week-old males, body weight: 20-23 g) were obtained from Orient, Inc. (Seong-Nam, Korea). After hair removal, the skin of the mice was wounded using a punch, and the wounded spots were treated with 100 μL of CM prepared from siNS- or siEll3-transfected ADSCs; SFM was used as the negative control. Wounded mouse skin tissues were harvested 7 days after CM treatment and then fixed in 4% formaldehyde.
Results and Discussion
To study the role of Ell3 in the secretome activity of ADSCs to repair skin wounds, we first suppressed Ell3 expression using a siRNA targeting Ell3 in ADSCs. As shown in Fig. 1A, Ell3 expression was suppressed up to 50% by siRNA transfection. We used media harvested from ADSCs transfected with siNS or siEll3 for 24 hours (siNS CM or siEll3 CM) as MEF culture media to examine the effects of CM on the proliferation of mouse fibroblasts. The proliferation rate of MEF cultured in siEll3 CM was significantly decreased compared to that of MEF cultured in siNS CM (Fig. 1B). The migration rate of MEF cultured in siEll3 CM was lower than that of MEF cultured in siNS CM, as determined by the scratch wound healing assay (Fig. 1C). The transwell assay also revealed that the migration ability of MEF cultured in siEll3 CM was lower than that of MEF cultured in siNS CM (Fig. 1D). Consistently, the expression of MMP family genes, which regulate diverse cell functions, including proliferation and migration, was lower in ADSCs cultured in siEll3 CM than in those cultured in siNS CM (Fig. 1E).
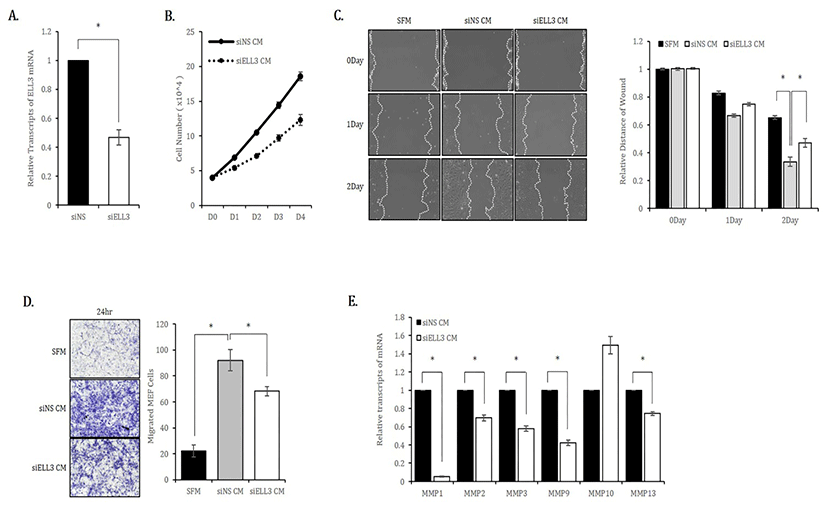
We next examined whether the results observed in mouse fibroblasts were reproduced in human fibroblasts. Similar to MEF, the proliferation rate of NHDF cultured in siEll3 CM was lower than that of NHDF cultured in siNS CM (Fig. 2A). The scratch/wound healing and transwell assays confirmed that Ell3 knockdown could suppress the ability of ADSC-CM to promote NHDF migration (Fig. 2B,C). Consistent with the results in MEF, the expression of MMP family genes in NHDF was significantly lower when cells were cultured in siEll3 CM (Fig. 2D).
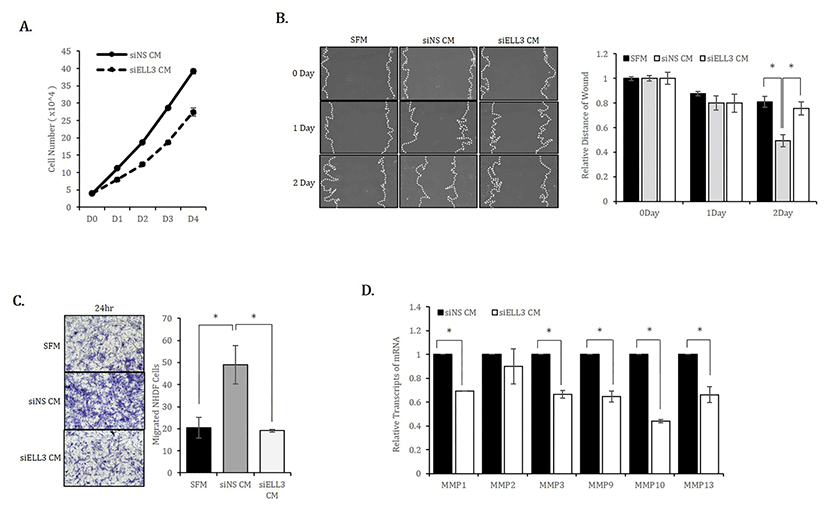
Based on these results, we concluded that Ell3 suppression in ADSCs diminishes the ability of CM to promote fibroblast proliferation and migration, which are two prominent in vitro parameters to estimate wound healing ability.
To evaluate the effect of Ell3 suppression on the wound healing ability of ADSC-CM in vivo, we applied siNS CM or siEll3 CM to mouse skin wounds that were induced by punch (Fig. 3A). SFM was applied to the wounds as a negative control, and the degree of open, fully thick excisional wound contraction was observed. As shown in representative lesions that were assessed 7 days after CM or SFM application, skin wounds treated with siEll3 CM recovered to a lesser extent than those treated with SFM (Fig. 3B). Assessing wound repair efficiency by examining the relative wound area on the indicated days after the application of CM or SFM showed that siEll3 CM could not enhance the wound healing rate, whereas siNS CM significantly promoted wound repair (Fig. 3C). In addition, H&E staining of wounded skin tissue revealed that siEll3 CM promoted inflammation (Fig. 3C). Because these results support the possibility that siEll3 CM promotes the expression of proinflammatory cytokines in fibroblasts, we analyzed the effect of Ell3 suppression on the expression of proinflammatory cytokines, including interleukin-1 (IL-1) and interleukin-6 (IL-6), in MEF treated with siNS CM or siEll3 CM. As expected, the expression levels of IL-1 and IL-6 were significantly increased in MEF treated with siEll3 CM compared to the levels in those treated with the control (Fig. 3D). Overall, our work further elucidates the role of Ell3 in the wound healing efficiency of ADSC-CM, although the downstream target genes regulated by Ell3 remain to be identified.
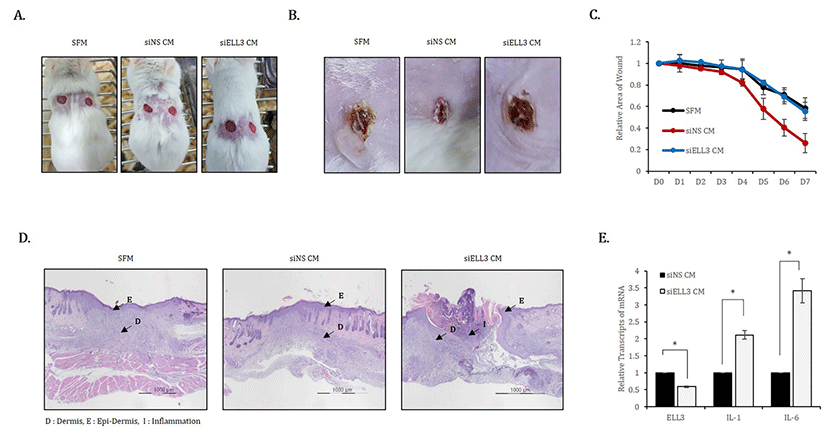