INTRODUCTION
Cyprinus carpio belongs to Cypriniformes, order Cyprininae, which also includes Carassius auratus, C. curvieri, Israeli carp C. carpio, and Ctenopharyngodon idellus, comprising three genera and five species (Kim et al., 2005).
The history of carp was first documented in 500 B.C. by Fan Li in China through his book Yaneogyeong on fish culture technology. Carp farming in Korea started seriously with the establishment of a national fish farm in Jinhae in 1929 (Aquaculture, 2009).
Carp color, shape, and habits differ according to the region and environment; therefore, there is extensive research being conducted to improve their breeds (Kang, 1990). Previous carp research in Korea included the development of black carp (Kim et al., 1999), genetic comparisons of C. carpio and C. curvieri (Yoon & Park, 2006), morphological variations (Nam et al., 1989), and egg and larval morphological development (Han et al., 2001a), and global research included multi-species aquaculture systems (Azim & Wahab, 2003), growth hormones (Cook et al., 1982), and viruses (Chen et al., 2008).
Carp is the main species used in freshwater fish production. However, water pollution in rivers and dams has increased owing to domestic and industrial sewage. Therefore, carp production has decreased considerably since cage culture was prohibited as a part of a protection policy for drinking water sources. Further, ecological damage has increased owing to the introduction of exotic fish. Therefore, there has been an increased interest in seed production and fish release projects to recover these resources (Aquaculture, 2009; Han et al., 2001a).
Research on the initial life cycle of fish is fundamental for understanding the biological characteristics of the species; thus, phylogenetic systematics of similar species is important. Initial life cycle research is particularly important concerning freshwater fish to restore species and resources, since the numbers of fish and habitats are both decreasing (Blaxter, 1974; Kim et al., 2012).
Initial life cycle research on carp include the following species: Rhynchocypris oxycephalus (Han et al., 1999), Pseudopungtungia nigra (Lee et al., 2004), Gobiobotia macrocephala (Ko et al., 2011a), G. brevibarba (Ko et al., 2011b), P. tenuicorpa (Ko et al., 2012), Microphysogobio koreensis (Kim et al., 2012), and Zacco koreanus (Lee et al., 2013).
Carp life cycles have been reported in inland water culture journals and aquaculture-related books, but limited research on egg development processes and larval morphological development has been briefly reported by Uchida (1939).
Therefore, this research aims to investigate carp egg and larval morphological development as part of initial life cycle research to provide basic data for resource conservation and taxonomic research.
MATERIALS AND METHODS
Brood (total length 67.3-75.5 cm, average 71.0±3.45 cm) used in the experiment were bred in a circular rearing aquarium (600×300×100 cm) made from polyvinyl chloride (PVC), using a running water system from January to July, 2015. Extrusion pellets were used as feed, which was given twice a day. Breeding water temperature was maintained at 23.0-25.0℃(average 24.0℃). The temperature was increased to 26.0-27.0℃(average 26.5±0.05℃) to induce spawning, and an artificially produced spawning tank was installed in the aquarium to induce natural spawning to harvest fertilized eggs.
Fertilized eggs were placed in a 500 mL glass beaker, and the breeding water temperature was maintained at 23.0- 25.0℃(average 24.0℃). The number of eggs was reduced by half twice a day. The egg size of 50 randomly selected eggs was measured using a phase microscope (Leica microsystem EZ4HD, Germany). The egg size was measured nearest to 0.01 mm.
Larvae were placed in a circular PVC aquarium (600× 300×100 cm) with a still-water system and aeration to provide oxygen immediately after hatching. Larvae at 10 d after hatching were fed 4-5 artemia (nauplii of Artemia sp.) per mL, and larvae 10-30 days were fed 2-3 freshwater fleas (Daphnia similis) per mL.
Larvae morphological development processes were observed daily in five larvae under anesthezia (MS-222, Ethyl 3-aminobenzoate methanesulfonate, Sigma Aldrich Co., St. Louis, USA) after hatching using a stereoscopic microscope (Nikon SMZ18, Japan), measured to the nearest 0.01 mm. The stages of larvae morphological development were categorized according to Kendall (1984).
RESULTS
Fertilized eggs were translucent and globular, and did not exhibit an oil globule. Egg length was 1.75-1.89 mm (average 1.82±0.06 mm) (Fig. 1A). The surface of the egg was covered with a highly adhesive phlegmatic material. The demersal eggs sank underwater.
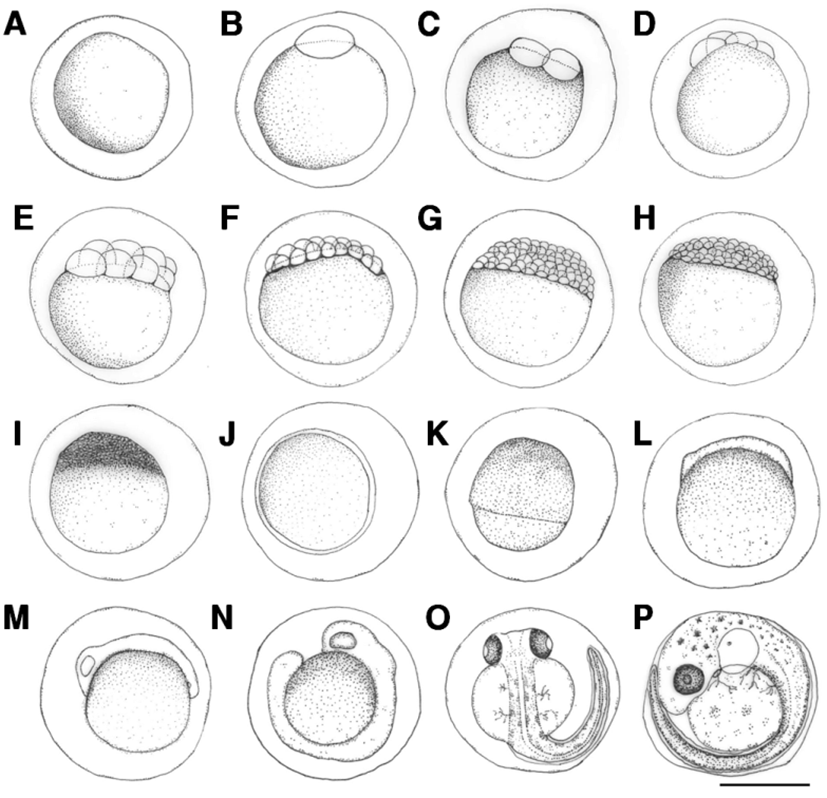
The blastoderm formed 10 min after fertilization (Fig. 1B), and reached the 2-cell stage 30 min after fertilization (Fig. 1C), and 4-cell stage after fertilization (Fig. 1D). The egg reached the 8-cell stage 1 hrs and 50 min after fertilization (Fig. 1E), 16-cell stage 2 hrs and 30 min after fertilization (Fig. 1F), 32-cell stage 3 hrs and 10 min after fertilization (Fig. 1G), and 64-cell stage 3 hrs and 50 min after fertilization (Fig. 1H). The egg reached the morula stage 4 hrs and 10 min after fertilization (Fig. 1I), blastula stage 5 hrs and 20 min after fertilization (Fig. 1J), and gastrula stage 6 hrs and 40 min after fertilization (Fig. 1K). The embryo formed when the blastoderm covered the yolk at 11 hrs and 45 min after fertilization (Fig. 1L), and optic vesicles, otocysts, and Kupffer’s vesicles formed and the embryo started moving 20 hrs and 7 min after fertilization (Fig. 1M). Kupffer’s vesicles disappeared, melanophores formed in the eyes, and the tail lengthened to separate from the yolk 22 hrs and 10 min after fertilization (Fig. 1N). Melanophores in the eyes darkened and were also found along the spine in the shape of tree branches 42 hrs and 50 min after fertilization. The membrane fin formed in the tail and blood started flowing from the heart across the yolk. The heartbeat at this stage was 68-70 beats per minute (Fig. 1O). Otoliths formed in the cephalus, tree branch-shaped melanophores were widely present in the abdomen and the yolk, and the heart rate increased to 100-107 beats per minute 70 hrs and 26 min after fertilization. Larvae immediately prior to hatching started to emerge from the egg membrane from the head to start hatching (Fig. 1P).
The length of prelarvae immediately after hatching was 5.23-5.38 mm (average 5.31±0.11 mm). The mouth and anus were unopened, pectoral fin was formed, and membrane fin was formed from the upper cephalus to lower abdomen. Melanophores in the shape of tree branches formed from the cephalus and upper egg yolk across the center of the spine to the tail end (Fig. 2A).
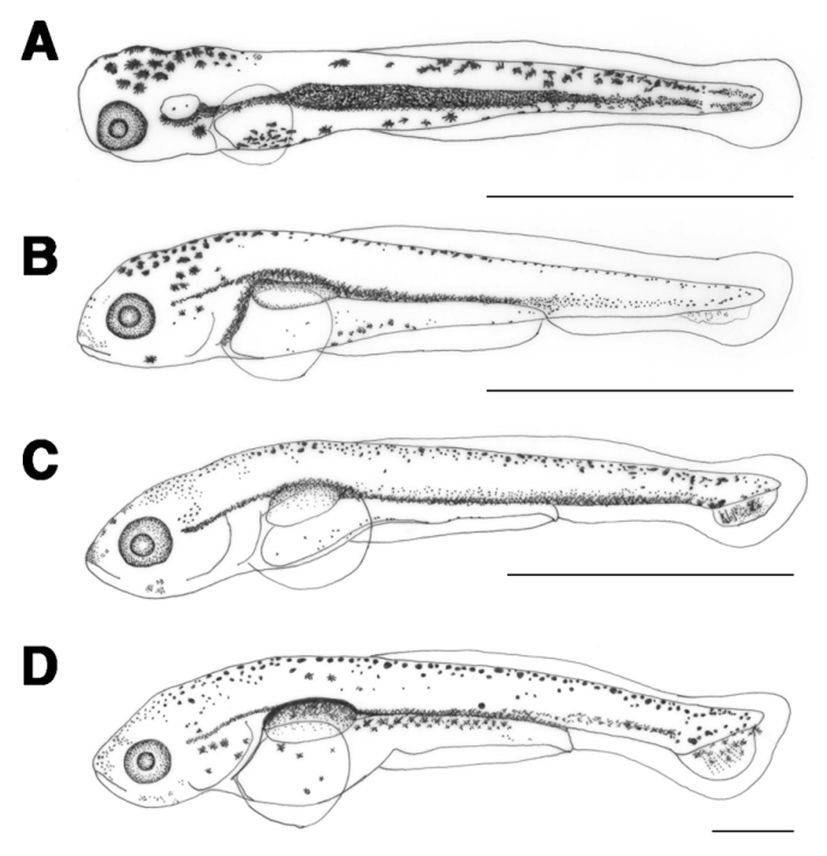
The length of prelarvae 1 days after hatching was 5.97-6.20 mm (average 6.09±0.16 mm). The mouth and anus were open, air bladder was formed, and they were able to swim. The membrane caudal fin was circular and tree branch-shaped melanophores were found in the upper air bladder. The location of anus at this stage was at 76.9% of total body length (Fig. 2B).
The length of prelarvae 5 days after hatching was 7.24-7.56 mm (average 7.40±0.22 mm). Their lower jaw was longer than their upper jaw and a soft ray started to form in the caudal fin. Melanophores were found where caudal fin rays formed (Fig. 2C).
The length of prelarvae 7 days after hatching was 7.86-8.27 mm (average 8.06±0.28 mm). Melanophores in the shape of tree branches in the upper air bladder darkened and those in the caudal fin ray widened. In the caudal fin, 6 soft rays formed and the caudal notochord started to bend at 45° (Fig. 2D).
The length of postlarvae 13 days after hatching was 9.93- 11.2 mm (average 10.6±0.92 mm). Melanophores in the shape of tree branches were found all over the body. The length of the lower jaw was greater than that of the upper jaw, and the membrane dorsal fin began to differentiate. There were 18 rays in the caudal fin and the location of the anus at this stage was at 78.1% of the total body length, which moved from the center to the back of the body (Fig. 3A).
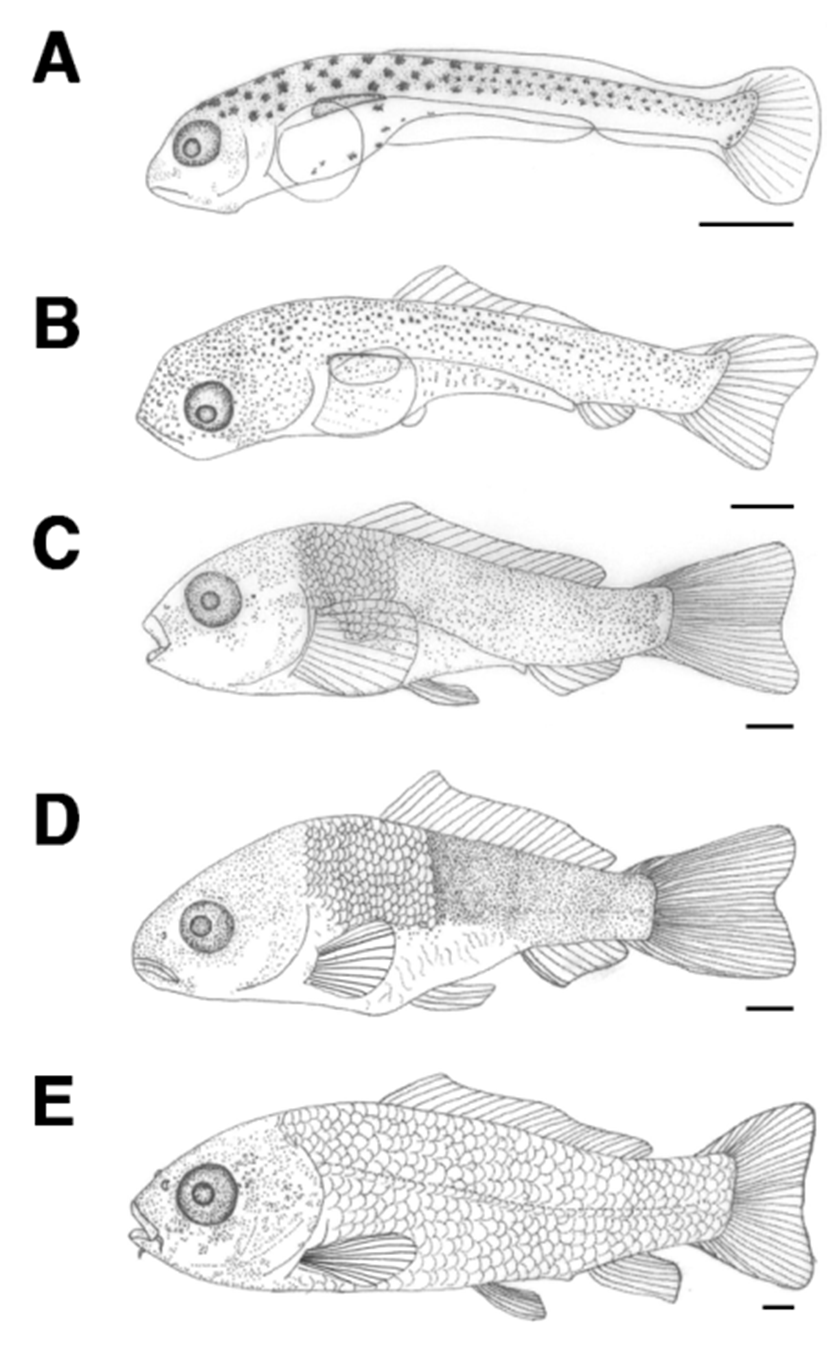
The length of postlarvae 18 days after hatching was 11.9- 13.9 mm (average 12.9±1.40 mm). Membrane dorsal and anal fins began to separate and rays started to form. Tree branch-shaped melanophores occurred in the dorsal fin. There were 9 fin rays in the dorsal fin and 6 in the anal fin (Fig. 3B).
The length of juvenile fish 23 days after hatching was 16.4–21.1 mm (average 18.7±3.34 mm). Scales formed from the top of the operculum to the anus and melanophores that previously covered the whole body now occurred in a speckled shape. The numbers of fin rays in each body part were increased to 6 in ventral fin, 19 in dorsal fin, 7 in anal fin, and 32 in caudal fin. The location of the anus at this stage was at 51.3% of the whole body length (Fig. 3C).
The length of juvenile fish, 30 days after hatching, was 21.8–22.4 mm (average 22.1±0.41 mm). One pair of whiskers has formed and scales covered 2/3 of the body. The numbers of fin rays increased to 8 in the ventral fin, 24 in the dorsal fin, and 9 in the anal fin (Table 1; Fig. 3D).
The length of juvenile fish 35 days after hatching was 29.9–30.2 mm (average 30.1±0.13 mm). Scales formed all over the body and the length of the upper jaw was greater than that of the lower jaw. Lateral lines were clearly visible and the location of the anus was at 60.4% of the body length, between the central and rear part of the body (Fig. 3E).
DISCUSSION
Spawning of C. carpio occurs amongst aquatic plants in shallow water by mature males and females at early dawn similar behavior is observed in C. carassius and C. cuvieri. Order Gobioniae (Cypriniformes), such as G. macrocephala, G. brevibarba, and M. koreensis, spawn in rapids with sand or pebbles, and Pungtungia herzi, P. tenuicorpa, and P. nigra parasitize the spawning ground of Coreoperca herzi reflecting differences in spawning habitats and behavior between species.
The average length of fertilized C. carpio eggs was 1.82±0.06 mm, which is similar to that reported in Uchida (1939) with a diameter of 1.83 mm, which was longer than that of C. carassius (Han et al., 2001a) of 1.57 mm diameter in the same order, G. macrocephala (Ko et al., 2011a) of 0.89 mm diameter in order Gobioninae, G. brevibarba (Ko et al., 2011b) with 1.24 mm, M. koreensis (Kim et al., 2012) of 1.80 mm, and R. oxycephalus (Han et al., 1999) of 1.80 mm in Leuciscinae, and shorter than Pseudorasbora parva (Han et al., 2001b) of 1.86 mm diameter, P. tenuicorpa (Ko et al., 2012) of 1.96 mm, P. herzi (Lee et al., 2002) of 2.10 mm, P. nigra (Lee et al., 2004) of 2.18 mm, and Z. koreanus (Lee et al., 2013) of 3.09 mm in order Danioniae, reflecting differences in fertilized egg size between species and orders. The size of fertilized eggs is directly proportional to the size of postlarvae thus, smaller postlarvae had smaller fertilized eggs. Since the size of fertilized eggs is also directly related to incubation time and fecundity, it is closely related to the initial breeding strategy of each species, and for species in the same genus, with similar ecological statuses.
Optimum water temperature differed in fish species according to their life cycle and ecological characteristics; water temperature is an environmental factor affecting initial egg development, and larval growth and survival (Cho et al., 2015). Comparison of the incubation time required for the egg to hatch showed that carp required 70 hrs and 26 min in average water temperatures of 20.0±0.05℃, according to a study by Cole et al. (2004), the time required for hatching was 120 h at 18℃, and 55 hours at 25℃; thus the time required was lower at higher water temperatures. C. carassius (Han et al., 2001a) needed 75 hrs and 20 min at 22.4℃, R. oxycephalus (Han et al., 1999) 88 hrs and 40 min at 19.0℃. P. nigra (Lee et al., 2004) required 189 hrs in an average water temperature of 19.0℃, G. macrocephala (Ko et al., 2011a) needed 107 hrs at 23.0℃, and G. brevibarba (Ko et al., 2011b) 100 hrs at 23.0℃. P. tenuicorpa (Ko et al., 2012) required 168 hrs in an average water temperature of 23.0℃, M. koreensis (Kim et al., 2012) needed 29 hrs at 23.0℃, and Z. koreanus (Lee et al., 2013) 68 hrs at 20.0–23.0℃. P. herzi (Lee et al., 2002) required 185 hrs and 55 min in an average water temperature of 19.0℃ and P. parva (Han et al., 2001b) required 183 hrs and 44 min at 17.6℃, reflecting differences in incubation time according to water temperature.
Most Cyprinidae, including C. carpio, C. carassius, R. oxycephalus, M. koreensis, and Z. koreanus showed a trend of shortened incubation times in water temperatures higher than 20.0℃. However, since R. oxycephalus, P. nigra, and P. herzi showed different incubation times when all species were bred in water temperatures of 19.0℃, the phenomenon may be closely related to the fertilized egg size, the larger sized eggs requiring longer incubation periods, rather than being affected by water temperature, although its effects cannot be ignored (Sado & Kimura, 2002; Lee et al., 2013).
A comparison of larval size immediately after hatching showed the average total length of C. carpio was 5.31± 0.11 mm, in contrast to a report by Uchida (1939) with a length of 5.00 mm. In contrast, Cole et al. (2004) reported the average total length of hatching prelarvae to be 3.72 mm, indicating that total length differs within species. Therefore, differences in the size of hatching prelarvae according to the regional distribution of carp should be studied.
The average total length of C. carpio was greater than that of P. parva (5.16 mm, Han et al., 2001b), M. koreensis (2.20 mm, Kim et al., 2012), C. carassius (4.28 mm, Han et al., 2001a), G. macrocephala (4.60 mm, Ko et al., 2011a), R. oxycephalus (4.94 mm, Han et al., 1999), and smaller than that of G. brevibarba (5.50 mm, Ko et al., 2011b), P. herzi (6.00 mm, Lee et al., 2002), P. nigra (5.82 mm, Lee et al., 2004), P. tenuicorpa (8.60 mm, Ko et al., 2012), and Z. koreanus (10.3 mm, Lee et al., 2013).
The time required for absorption of the egg yolk after hatching differed, being 4–5 days for C. carpio, while C. carassius (Han et al., 2001a), in the same order, required 5 days; R. oxycephalus (Han et al., 1999) in the same order, Cypriniformes, required 6 days; P. herzi (Lee et al., 2002) required 7–8 days; P. parva (Han et al., 2001b), P. nigra (Lee et al., 2004), and G. brevibarba (Ko et al., 2011b) required 3 days; P. tenuicorpa (Ko et al., 2012) and M. koreensis (Kim et al., 2012) required 2 days; G. macrocephala (Ko et al., 2011a) required 4 days; and Z. koreanus (Lee et al., 2013) required 6 days.
The time of transition to postlarvae for C. carpio was at 5 days after hatching with an average total length of 7.40±0.22 mm, in contrast to a report by Uchida (1939), who stated the transition to be at 10 days after hatching with an average total length of 8.50 mm. The time of transition to postlarvae for C. carassius (Han et al., 2001a) was at 5 days after hatching with an average total length of 5.34 mm, and for P. herzi (Lee et al., 2002) 13 days after hatching at 7.60 mm, for P. parva (Han et al., 2001b) 7 days after hatching at 6.37 mm, R. oxycephalus (Han et al., 1999) 6 days after hatching at 6.44 mm, for P. nigra (Lee et al., 2004) 6 days after hatching at 8.06 mm, for G. macrocephala (Ko et al., 2011a) 4 days after hatching at 6.10 mm, for G. brevibarba (Ko et al., 2011b) 4 days after hatching at 7.40 mm, for P. tenuicorpa (Ko et al., 2012) 2 days after hatching at 9.00 mm, for M. koreensis (Kim et al., 2012) 4 days after hatching at 3.60 mm, and Z. koreanus (Lee et al., 2013) 13 days after hatching at 16.9 mm.
The time of transition to juvenile fish for C. carpio was at 30 days after hatching with an average total length of 22.1 mm and for C. carassius (Han et al., 2001a) 31 days after hatching at 14.6 mm, reflecting little difference in terms of days after hatching, but a difference in the total length. The time of transition to juveniles for P. parva (Han et al., 2001b) was at 65 days after hatching with an average total length of 14.6 mm, and P. herzi (Lee et al., 2002) was at 45 days after hatching with 14.6 mm, and R. oxycephalus (Han et al., 1999) was at 80 days after hatching with 18.7 mm, and for P. nigra (Lee et al., 2004) 44 days after hatching with 14.5 mm, both of which took a longer time at a shorter total length to become juveniles than did C. carpio. The time of transition to juveniles for G. macrocephala (Ko et al., 2011a) was 15 days after hatching with an average total length of 8.60 mm, for G. brevibarba (Ko et al., 2011b) 15 days after hatching at 9.90 mm, for P. tenuicorpa (Ko et al., 2012) 10 days after hatching at 10.6 mm, for M. koreensis (Kim et al., 2012) 20 days after hatching at 6.50 mm, and for Z. koreanus (Lee et al., 2013) 27 days after hatching at 20.0 mm, all of which took a shorter time and total length to become juveniles than did C. carpio(Table 2).
Species | Egg diameter(mm) | Time of hatching(*WT) | Days until yolk sac absorption (day) | Newly hatched larvae size (mm) | Postlarvae size(mm) | Juveniles size(mm) | References |
---|---|---|---|---|---|---|---|
Cyprinus carpio | 1.82 | 70 h 26 mins(20.0℃) | 4-5 | 5.31 | 7.40 | 22.1 | Present study |
1.83 | - | - | - | 8.50 | - | Uchida, 1939 | |
- |
120 h (18.0℃) 55 h (25℃) |
- | 3.72 | - | - | Cole et al., 2004 | |
Carassius carassius | 1.57 | 75 h 10 mins(22.4℃) | 5 | 4.28 | 5.34 | 14.6 | Han et al., 2001a |
Gobiobotia macrocephala | 0.89 | 107 h (23.0℃) | 4 | 4.60 | 6.10 | 8.60 | Ko et al., 2011a |
Gobiobotia brevibarba | 1.24 | 100 h (23.0℃) | 3 | 5.50 | 7.40 | 9.90 | Ko et al., 2011b |
Microphysogobio koreensis | 1.80 | 29 h (23.0℃) | 2 | 2.20 | 3.60 | 6.50 | Kim et al., 2012 |
Rhynchocypris oxycephalus | 1.80 | 88 h 40 mins(19.0℃) | 6 | 4.94 | 6.44 | 18.7 | Han et al., 1999 |
Pseudorasbora parva | 1.86 | 183 h 44 mins (17.6℃) | 3 | 5.16 | 6.37 | 14.6 | Han et al., 2001b |
Pseudopungtungia tenuicorpa | 1.96 | 168 h (23.0℃) | 2 | 8.60 | 9.00 | 10.6 | Ko et al., 2012 |
Pungtungia herzi | 2.10 | 185 h 55 mins(19.0℃) | 7-8 | 6.00 | 7.60 | 14.6 | Lee et al., 2002 |
Pseudopungtungia nigra | 2.18 | 189 h (19.0℃) | 3 | 5.82 | 8.06 | 14.5 | Lee et al., 2004 |
Zacco koreanus | 3.09 | 68 h(20.0-23.0℃) | 6 | 10.3 | 16.9 | 20.0 | Lee et al., 2013 |
These differences in total length according to development processes will become clearer with further research on the characteristics of their microhabitats, and ecological and physiological research (Kim et al., 2005b; Lee et al., 2013). Since C. carpio inhabits habitats with lower flow velocity, comparative research on different habitats should be conducted in the future to verify the differences in characteristics of habitats.