INTRODUCTION
The epididymis is a part of the excurrent duct of male reproductive tract and is the place in which sperm becomes acquiring fertilizing capacity (Robaire & Hermo, 1988). The epididymis is structurally and functionally separated into 4 different regions, including initial segment, caput epididymis, corpus epididymis, and caudal epididymis (Robaire & Hermo, 1988). Among these regions of the epididymis, the caudal epididymis plays an important role for storage and quiescence of mature sperm (Robaire & Hermo, 1988). Thus, it is fully reasonable to consider that functional regulation of the caudal epididymis could give an influence on the male reproductive ability.
Like the other parts of the epididymis, the caudal epididymis has a layer of epithelial cells which faces the luminal space and is surrounded by multiple smooth muscle layers (Robaire et al., 2006). However, the caudal epididymis has different structural and histological features, compared with other compartments of the epididymis (Robaire et al., 2006). For examples, luminal diameter of the caudal epididymis is wider than the caput and corpus epididymis (Robaire et al., 2006). The highest proportion of the principal cells within the epididymal epithelium is found in the caudal epididymis (Robaire et al., 2006). Even though the clear cells, a cell type found in epididymal epithelium, are also present in the caput, corpus, and caudal epididymis, apical cells and narrow cells are absent or scare in the caudal epididymis (Robaire et al., 2006). It is relatively well determined that each cell type within the epididymal epithelium has unique structural characteristics and histological features, relating with its functions (Robaire et al., 2006). Therefore, it is proposed that functional regulation of different epididymal compartments is, in part, governed by behavioral modulation of epithelial cells.
The functions of cells in an animal tissue having multicellular types, such as the epididymis, could be regulated by direct cell-cell communication via connexin (Cx)-based gap junction linking adjacent cells (Lawrence et al., 1978). Exchanges of ions, small metabolites, and second messengers between neighboring cells are allowed through the gap junction (Valiunas et al., 2005). Gap junction is structurally consisted of 6 Cxs, and there are at least 21 Cx isoforms found in vertebrates (Goodenough & Paul, 2009). Expression of several Cx isoforms has been found in many types of mammalian tissues and even a specific cell type (Goodenough & Paul, 2009). In case of the epididymis, the presence and expression of 8 to 9 different Cx isoforms have been identified from our earlier researches and others (Cry, 2011; Lee, 2013; Seo et al., 2010). Also, the expression of Cx isoforms in the epididymis is segmental specific and/or postnatal development age-specific (Lee, 2013; Seo et al., 2010). Even though Cry et al (1996) has demonstrated the presence of Cx43 in the base of the epithelium between basal and principal cells in the rat epididymis, little information is available for the localization of other Cx isoforms in the epididymis.
Expressional regulation of Cx isoforms in the epididymis has been examined in our previous studies and others. St-Pierre et al (2003) have shown the drastic decrease of Cx43 expression in the initial segment, caput epididymis, and corpus epididymis, but not in the caudal epididymis by propylthiouracil-induced hypothyroidism at the neonatal age. Exposure to estradiol benzoate (EB), an estrogen agonist, or flutamide (Flu), an antiandrogenic compound, at the neonatal or weaning age results in aberrant expression of several Cx isoforms in the initial segment and corpus epididymis of the adult rat (Lee, 2014, 2015a, Lee, 2015b). However, it is notable that the expressional changes of Cx isoforms induced by EB or Flu treatment are different in each epididymal segment and/or a type of the treatment (Lee, 2014, 2015a, 2015b). Moreover, expressional patterns of Cx isoforms in a given region of the adult epididymis differ with the time of exposure to EB or Flu during postnatal period (Lee, 2014, 2015a). Most recently, our research has demonstrated expressional changes of Cx isoforms in the adult caudal epididymis administrated with EB or Flu at the early neonatal age (Lee, 2016). From these observations, it has been suggested that the exposure to EB or Flu at the different postnatal age would result in alternation of gene expression of Cx isoforms in the adult caudal epididymis. Thus, the present research was designed to examine the expressional change of Cx isoforms in the caudal epididymis of the adult rat treated with EB or Flu at the weaning age.
MATERIALS AND METHODS
Pregnant female Spragure Dawley rats were purchased from Samtako (OSan, Korea) and were individually caged. Each female rat was randomly assigned into one of five experimental groups; a control, a low-dose estradiol benzoate (EB)-treated (EB-L, 0.015 µg of EB/kg body weight (BW)), a high-dose EB-treated (EB-H, 1.5 µg of EB/kg BW), a low-dose flutamide (Flu)-treated (Flu-L, 500 µg of Flu/kg BW), or a high-dose Flu-treated (Flu-H, 5 mg of Flu/kg BW) group. For each experimental group, five to seven male pups obtained from the delivery of the female rats were used for the present research. Experimental animals were allowed to freely access to drinking water and food for entire experiment period.
Once the experimental animal became at the weaning age, a vehicle (peanut oil) or a chemical compound was subcutaneously administrated into the animal. To prepare the EB or Flu solution, the EB and Flu powder purchased from Tokyo Chemical Industry Co. (Tokyo, Japan) was first completely dissolved in 100% EtOH, and then the EB or Flu solution was diluted in peanut oil. At the weaning age, body weight of the experimental animal was measured, and the amount of EB or Flu solution to be injected was calculated. The injected volume of EB or Flu solution didn’t exceed 0.05 mL. A total of 31 male rats were used for this study, including control group (n=5), EB-L group (n=7), EB-H group (n=7), Flu-L group (n=6), and Flu-H group (n=6). The present study was carried out in accordance with the guide for the care and use of laboratory animals of National Research Council in S. Korea.
The tissue was isolated from the experimental animals at 4 months of postnatal age. First, the animals were euthanized by CO2 stunning, and then an incision on lower abdomen was made with a pair of scissors. The male reproductive tract was taken from the animal and was placed in the cold PBS solution. The testis and epididymal fat were separated from the epididymis, and the epididymis was quickly dissected into four different regions, such as initial segment, caput epididymis, corpus epididymis, and caudal epididymis. Each part of the epididymis was rinsed in fresh cold PBS solution and rapidly frozen in liquid nitrogen. The tissue was stored in -80oC until used for the isolation of total RNA.
The extraction of total RNA from collected caudal epididymis was carried out as following. The tissue was homogenized in total RNA extraction solution (iNtRON Biotech, Sungnam, Korea) with a homogenizer (Fisher Scientific, Pittsburgh, PA). Total RNA precipitated by isopropanol was washed with 70% DEPC-treated EtOH , and air-dried total RNA pellet was resuspended in DEPC-treated RNase-free dH2O. The concentration and ratio of total RNA were measured with NanoDrop Lite spectrophotomer (Thermo Scientific, Wilmington, DE). One microgram of total RNA was used to determine the quality of the total RNA sample by agarose gel electrophoresis.
One microgram of total RNA was utilized to construct the first-stranded cDNA with oligo-dT primer and ImProm-IITM reverse transcription system (Promega, Madision, WI). The reaction of reverse transcription was performed in a sequential process of 25℃ for 5 min, 42℃ for 90 min, and 70℃ for 15 min. Generated cDNA was directly utilized for quantitative real-time PCR analysis. Table 1 shows detailed information of oligonucleotide primers used for quantitative real-time PCR.
To conduct the quantitative real-time PCR, a mixture of 1 µL cDNA, 10 pmol primer set, 10 µL PCR master mixture (Finnzymes, Espoo, Finland), and DNase-free dH2O to make a total volume of 20 µL was prepared for PCR analysis. The PCR condition was as followings; a pre-denaturation step at 95℃ for 30 sec, 40 cycles of denaturation at 95℃ for 30 sec, annealing at Tm for 30 sec, and extension at 72℃ for 30 sec. Cyclophilin A (Ppia) was included for a quality internal control of PCR. The sizes of all PCR products were checked with the electrophoresis in 1.2% agarose gel.
A mean and a standard error of an experimental group were obtained from the data of independently triplicated reverse transcription reaction and PCR. The data represent the relative expressional ratio between Ppia and target Cx isoform. Statistical differences of Cx expressional levels among EB or Flu treatment groups and control group were determined by one-way ANOVA, if necessary, followed by Duncan’s test. Once p<0.05, it was considered the presence of statistical significances among experimental groups.
RESULTS
Expression of Cx26 was significantly increased by EB treatment, even though there was no significant difference on EB-induced transcript level of Cx26 gene between a low-dose treated group and a high-dose treated group (Fig. 1A). Induction of Cx26 gene expression was also observed in flutamide-treated groups (Fig. 1A). However, the increment of Cx26 transcript by the treatment of a low-dose flutamide was not significantly different with that by the treatment of a high-dose flutamide (Fig. 1A).
No significant change of Cx30.3 transcript level was detected by EB treatment at both doses (Fig. 1B). Unlikely, the treatment of flutamide resulted in a significant increase of Cx30.3 gene expression (Fig. 1B). But, expressional change of Cx30.3 gene between two flutamide-treated groups was not significantly different (Fig. 1B).
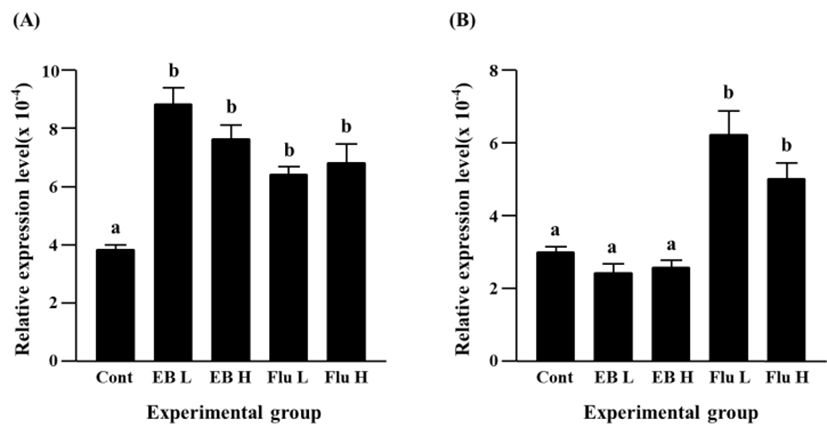
A significant increase of Cx31 expression was detected with a low-dose EB treatment (Fig. 2A). A further increase of Cx31 transcript level was found in a high-dose EB treatment, and the increment of Cx31 expression by a high-dose EB treatment was significantly higher than that by a low-dose EB treatment (Fig. 2A). Expression of Cx31 gene was also significantly increased by flutamide treatment (Fig. 2A). Like in case of EB treatment, a high-dose flutamide treatment resulted in more expression of Cx31 gene than a low-dose flutamide treatment (Fig. 2A).
The EB treatments at different doses caused significant decreases of Cx31.1 gene expression, and there was no significant different on transcript level of Cx31.1 gene by a low-dose and a high-dose EB treatments (Fig. 2B). In contrast, a significant increase of Cx31.1 gene expression was found in a low-dose flutamide-treated group (Fig. 2B). The treatment of a high-dose flutamide resulted in a further increase of Cx31.1 transcript level in the adult caudal epididymis (Fig. 2B).
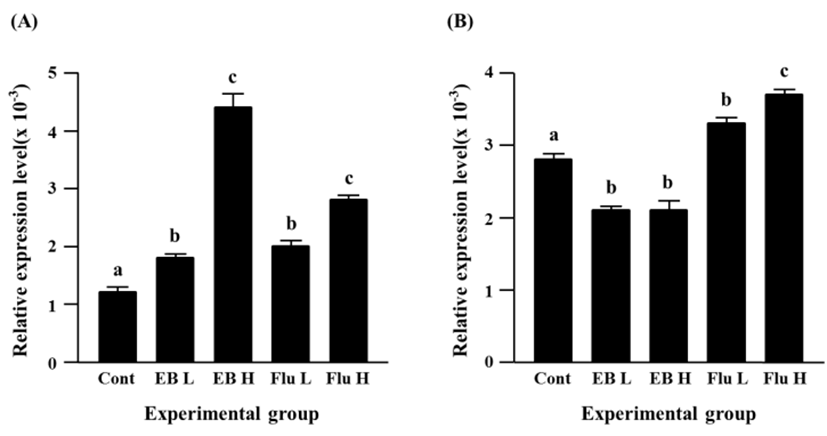
Expressional level of Cx32 gene was significantly increased by EB treatment, even though there was no significant difference on the increment of Cx32 transcript levels between a low-dose and a high-dose EB treatment (Fig. 3A). The flutamide treatment also caused an increase of Cx32 transcript level (Fig. 3A). It is notable that an induction of Cx32 gene expression in a high-dose flutamide-treated group was about 10 times higher than that in control group (Fig. 3A).
The exposure to a low-dose EB resulted in a significant decrease of Cx37 expression, while there was no significant change of Cx37 transcript level by a high-dose EB treatment, compared with the control (Fig. 3B). Transcript level of Cx37 was significantly increased by a low-dose flutamide treatment, and a further significant increase of Cx37 gene expression was observed in a high-dose flutamide-treated group (Fig. 3B).
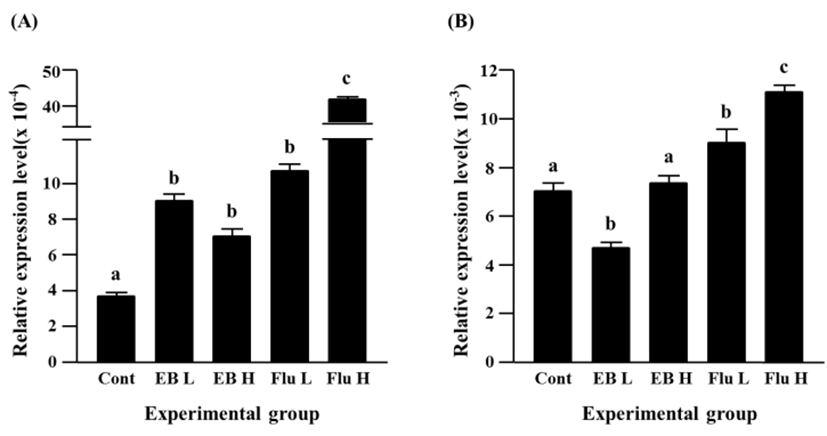
The treatment of a low-dose EB didn’t give on an influence on expression of Cx40, however a significantly increased expression of Cx40 gene was observed in a high-dose EB-treated group (Fig. 4A). The flutamide treatments induced significant increases of Cx40 gene expression, and the increment of Cx40 transcript level was significantly higher in a high-dose flutamide treatment than a low-dose flutamide treatment (Fig. 4A).
The EB treatments resulted in significant increases of Cx43 transcript levels (Fig. 4B). The increment of Cx43 expression by a low-dose EB treatment was significantly higher than that by a high-dose EB treatment (Fig. 4B). The expression of Cx43 gene was also significantly increased by the exposure to flutamide, even though there was no significant difference on the transcript level of Cx43 gene between a low-dose flutamide group and a high-dose flutamide group (Fig. 4B).
The expression of Cx45 gene was suppressed by the EB treatment (Fig. 4C). A significant decrease of Cx45 transcript level was detected by a low-dose EB treatment, and a further decrease of Cx45 expression was found in a high-dose EB group (Fig. 4C). Compared with the control, the transcript level of Cx45 gene was significantly decreased by a low-dose flutamide treatment, while a significant increase of Cx45 expression was found in a high-dose flutamide group (Fig. 4C).
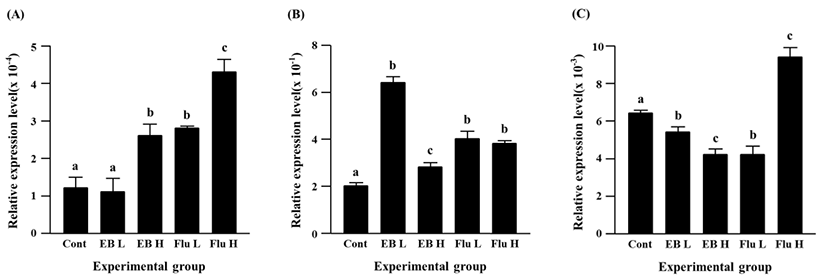
DISCUSSION
Alteration of normal gene expression in the epididymis is frequently observed by the exogenous administration to steroidal agonistic and/or antagonistic compounds. Specially, exposure to such substances during the early postnatal period results in more evident outcomes in abnormal expression of various genes in the epididymis (Gorowska et al., 2014). The data from the present research clearly show that a single administration of EB or Flu at a weaning age is sufficient to disrupt normal expression of Cx isoforms in the adult caudal epididymis.
Segmental-specific expression patterns of Cx isoforms in the epididymis have been examined from our previous study (Lee, 2013). It is acceptable to consider that the findings from the current research should be compared with the outcomes conducted with same epididymal segment, rather than with those obtained from the different epididymal segment with same treatment. Thus, the significant findings driven from the present research have been evaluated with those from the treatment of EB or Flu at 7 days of postnatal age, previously reported (Lee, 2016).
The treatment of a low-dose EB at 7 days of age results in a significant increase of Cx32 transcript level and causes meaningful decreases of Cx30.3, Cx31.1, Cx37, and Cx45 transcript levels in the adult caudal epididymis (Lee, 2016). However, as observed in the present research, the same treatment at 21 days of age causes major increases of transcript levels of Cx26, Cx31, Cx37, and Cx43 but significant reduction of Cx31.1 and Cx45 transcript amounts. Interestingly, expression of Cx40 is only not influenced by a low-dose EB treatment at any postnatal age. The low-dose EB treatments at different postnatal ages result in differential expression patterns of other Cx isoforms, except Cx31.1 and Cx45, in the adult caudal epididymis. At this point, any clear explanation is not suggested to describe such differential responses on the expression of Cx isoforms by same treatment of EB or Flu at different postnatal age. Atanassova et al (2001) show the regional and postnatal age-specific expressional patterns of estrogen receptor alpha (ERα) in the epididymis and vas deferens. In addition, they demonstrate the disruption of expressional patterns of ERα by neonatal exposure to diethylstilbestrol (Atanassova et al., 2001). In addition, Putz et al (2001) show that the neonatal EB treatment affects normal gene expression in the adult epididymis. Based on others’ findings, it is proposed that the different postnatal time exposed to a low-dose EB would influence on the distinguishable expressional patterns of Cx isoforms in the adult caudal epididymis. Moreover, it could not rule out a possibility that expression of each Cx isoform would be differentially regulated by a low-dose EB due to its own specific responsiveness to EB, as shown in Hermoso et al’s data (1997). Expressional changes of most Cx isoforms, except Cx37 and Cx40, by the treatment of a high-dose EB are not quite different with those by the treatment of a low-dose EB, even though there are some expressional variations of Cx31, Cx43, and Cx45 genes. There is no evident suggestion to describe such differential responses at some Cx isoforms to two EB concentrations. Detailed genomic analyses of Cx isoforms would be helpful to elucidate possible answers.
It is interested that the Flu treatments at two doses result in significant increases of transcript levels of all Cx isoforms, except a significant decrease of Cx45 transcript level by a low-dose Flu treatment. These observations slightly differ with those from the treatment of Flu at 7 days of age (Lee, 2016). The expressional level of androgen receptor (AR) in the epididymis is gradually increased with postnatal ages, especially during the early postpartum period (You & Sar, 1998). In addition, the differentiation of the epididymal epithelial cells does not occur until the second week of postnatal age (Robaire et al., 2006). The columnar cell, a precursor of principal cell, first appears at the third week of postnatal age and becomes fully differentiated into basal cell and principal cell at the fourth week of postnatal age (Robaire et al., 2006). Because the differentiation of epithelial cells in the epididymis is under age-dependent manner, it is suggested that exogenous treatment of EB or Flu at different postnatal age could give an influence on the differentiation of the epididymal epithelial cells during the early postnatal period. The change of the epithelial differentiation in the epididymis would result in the modification of cellular composition and thus normal gene expression. Thus, it is reasonable to consider that the treatment of EB or Flu at different age during the early postnatal period causes different expressional patterns of Cx isoforms in the epididymis.
In conclusion, the present research shows that the exposure to steroidal agonist or antagonist at the weaning age could modify normal expression of Cx isoforms in the adult caudal epididymis. However, it could not be directly addressed from the current study if the EB or Flu treatment at the early postnatal age results in malfunction of the adult caudal epididymis. However, it is postulated that expressional changes of Cx isoforms induced by EB or Flu treatment would give disruptive impact on the epididymal function, even though the effect could be negligible.