INTRODUCTION
According to the World Health Organization (WHO), obesity is a complex condition which affects virtually all age and socioeconomic groups and threatens to overwhelm both developed and developing countries. Since obesity is a medical circumstance in which excess body fat has accumulated to the extent, there has been considerable accumulation of research information about the adipose biology (Coelho et al., 2013). Several lines of evidence indicate that gonadal sex steroids are sex-specifically involved in the accumulation and distribution of adipose tissues. For example, distribution of fat is different between males and females; men have a more central accumulation of fat, whereas women have a more gluteal/femoral accumulation (Bjorntorp, 1996).
Meanwhile, it has become evident that adipose tissue is one of the major endocrine gland (Coelho et al., 2013). For a long time, adipocyte, also known as a fat cell, had been considered to be an inert cell that just stores fat. The importance of adipose tissue as an endocrine organ was fully appreciated in 1995 with the discovery of leptin, the protein product of the Ob gene (Pelleymounter et al., 1995). By the way, the endocrine potency such as aromatization of testosterone in adipose tissues also has been well noticed from studies using castrated animal models (Drago et al., 1982; Zhao et al., 2005). Analyses of human adipose tissue also revealed that several genes involved in steroidogenesis were expressed in adipose tissue (MacKenzie et al., 2008b). More recently, local production of steroids in adipocytes differentiated from mouse 3T3-L1 cell-line was reported (Li et al., 2014).
In the present study, we hypothesized that rat adipocytes have steroidogenic machinery and expression patterns of the components might be depot- and sex-specific. To verify this hypothesis, we collected the brown adipose tissue (BAT) and white adipose tissue (WAT), and subdivided the WAT into abdominal and reproductive fats from immature male and female rats. After the tissue collections, we performed semi-quantitative RT-PCRs for steroidogenic factors.
MATERIALS & METHODS
Postweaned Sprague-Dawley rats (PND 22) were obtained from Han-Lim Animal (Gyunggi-do, Korea) and reared in Sangmyung University animal facility under conditions of 12-h light/dark cycle (lights on at 07:00 h) and constant temperature of 22±1°C. Rats had free access to normal chow and tap water (ad libitum). All procedures used were approved by the Animal Care and Use Committee at Sangmyung University in accordance with guidelines established by the Korea Food and Drug Administration (KFDA).
After allowing 8 days acclimation, male and female animals were sacrificed and the adipose tissues were collected. The tissues were subdivided into abdominal (visceral fat over the intestines) and reproductive fats (also known as gonadal fats; epididymal and ovarian fat pad from male and female, respectively). Tissue samples were used to prepare the RNAs, and some part were used to confirm the morphological integrity of the collected tissues.
Tissue specimens were fixed 4% paraformaldehyde at 4°C for 24h. Fixed tissues were serially dehydrated in graded ethanol and xylene. Specimens were embedded in paraffin block. The tissues blocks were cut at 6 μm using microtome (HM350S, MICROM, Germany). Sections were stained with hematoxylin-eosin stain and examined under light microscope (BX51, Olympus, Japan).
Total RNAs were isolated from tissue samples using the single-step, acid guanidinum thiocyanate-phenol-chloroform extraction method. Total RNAs were used in RT-PCR reactions carried out with Maxime™ RT PreMix (InTron, Korea) and Accupower PCR Premix (GeneAll, Korea) according to the manufacturer’s instructions. Sequences of the primers and the specific PCR conditions used in this study were successfully worked in the previous study (Yoo & Lee, 2016), and were listed in Tables 1 and 2, respectively. The reaction products were analyzed by gel electrophoresis in 1.5% agarose gel (75V, 65min) and visualized by ethidium bromide staining. The band intensities were measured using the image analysis system (Imager Ⅲ-1D main soft ware, Bioneer, Korea). Glyceraldehydes-3-phosphate dehydrogenase (GAPDH) was used as reference gene for normalization of quantitative RT-PCRs in the present study.
All values are expressed as the means (±S.E.). Sex- and depot-dependent differences were analyzed by Student’s t-test or one-way analysis of variance (ANOVA). P values less than 0.05 were considered significant. The IBM PC programs INSTAT and PRISM 4.01 (GraphPad, USA) were used to calculate and plot the results.
RESULTS
Fig. 1 represents the cross sections of the adipose tissues used in the present study. Adipocytes of WAT from male and female animals were monovacuolar cells contain a large lipid droplet surrounded by a layer of cytoplasm, and their nuclei were flattened and located on the periphery (upper and middle rows). The overall size and shape of female reproductive adipocytes were relatively smaller and more flattened compared to those of abdominal adipocytes. Adipocytes of BAT were polygonal in shape (bottom row). Compared to WAT adipocytes, these cells have considerable cytoplasm, with much less lipid droplets scattered throughout in the cells. The nuclei were round and not in the periphery of the cells. Female brown adipocytes were amassed showing big 'island' like shape. These cross sections of adipose tissues were in good accordance with previously reported typical characteristics of rat white and brown adipose tissues (Cinti, 2000).
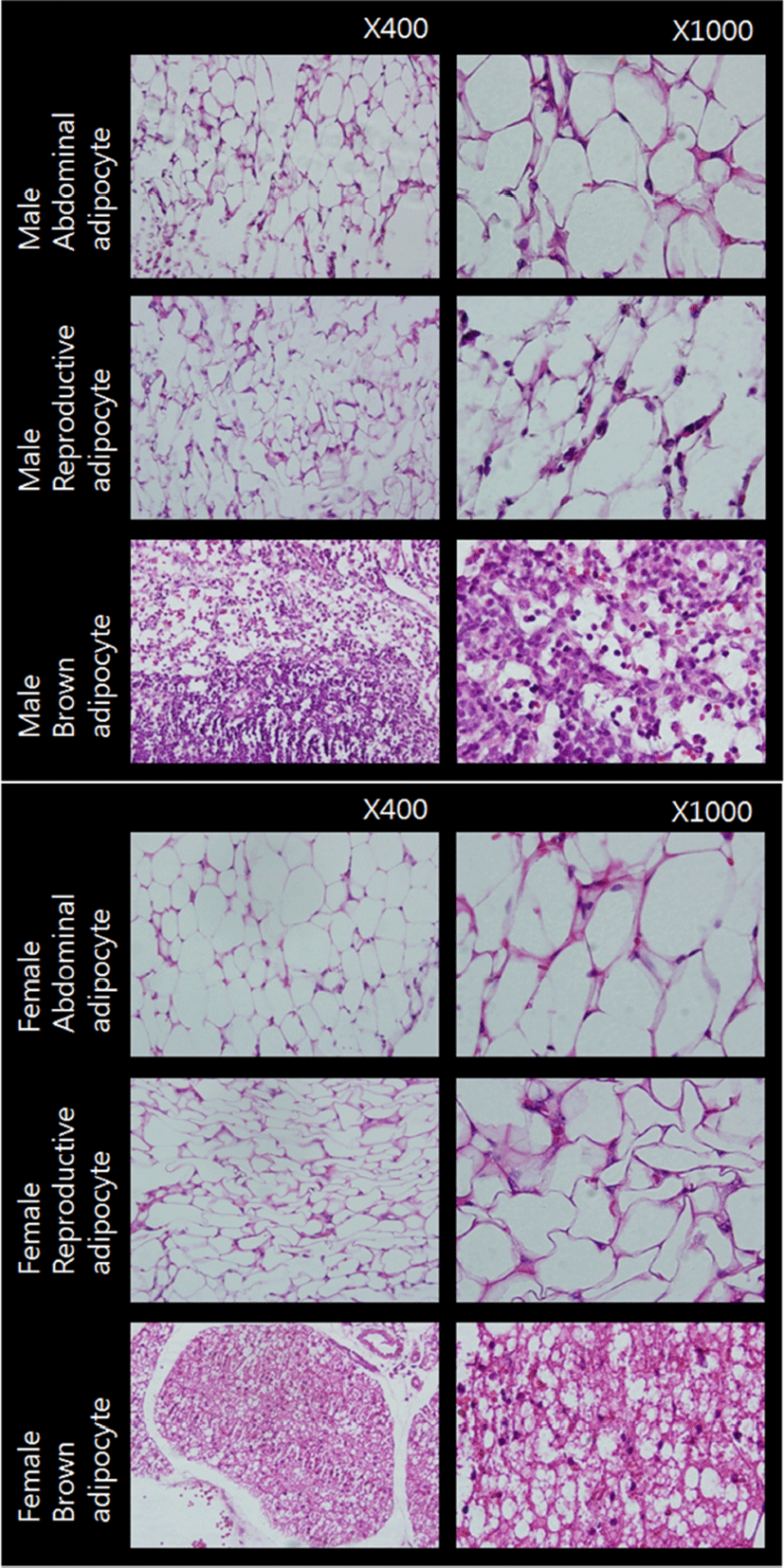
We performed quantitative RT-PCRs for 6 types of genes; Steroidogenic acute regulatory protein (StAR), Cholesterol side-chain cleavage enzyme (P450scc), 3-β-hydroxysteroid dehydrogenase (3β-HSD), Cytochrome P450 17A1 (CYP17), 17Beta Hydroxysteroid dehydrogenase (17β-HSD) and Aromatase (CYP19). All kind of transcripts were successfully amplified, and in overall aspects, the abundances of the transcripts were lower in the BAT of both sexes (Fig. 2, A-F).
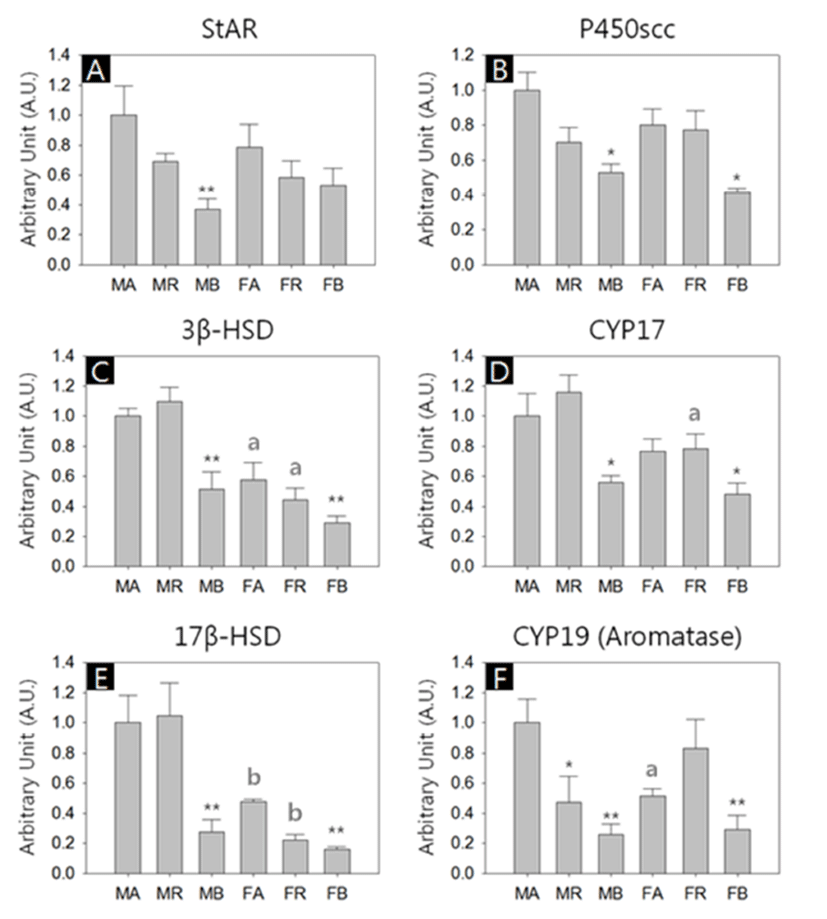
There was no significant difference of StAR transcript levels between male and female with same depots. However, the levels of the BAT were significant lower among male depots (MA:MR:MB = 1.00±0.16:0.69±0.05:0.37±0.07, p<0.01; Fig. 2A). The levels of P450scc transcripts in the BAT were significant lower compared to the other depots in both sexes (MA:MR:MB = 1.00±0.10:0.70±0.08:0.52±0.05, p<0.05; FA:FR:FB = 0.80±0.09:0.77±0.11:0.41±0.02, p<0.05; Fig. 2B). The 3β-HSD transcripts in female abdominal adipose and reproductive adipose were significantly lower than those of male counterparts (FA:FR:FB = 0.58±0.11:0.44±0.08: 0.29±0.04, p<0.05; Fig. 2C). In comparison of the levels between depots of same sex, BAT shown significant low abundance (MA:MR:MB = 1.00±0.05:1.10±0.10:0.51±0.12, p<0.01; FA:FR:FB = 0.58±0.12:0.44±0.08:0.29±0.05, p<0.01; Fig. 2C). As with P450scc, the levels of CYP17 transcripts in the BAT were significant lower compared to the other depots in both sexes (MA:MR:MB = 1.00±0.15:1.16±0.11: 0.56±0.04, p<0.05; FA:FR:FB = 0.77±0.08:0.78±0.10:0.48± 0.07, p<0.05, Fig. 2D). The CYP17 transcript levels in female reproductive adipose were significantly lower than that of male counterpart (MR:FR = 1.16±0.11:0.78±0.10, p<0.05; Fig. 2D). The 17β-HSD transcript levels in female abdominal adipose and reproductive adipose were significantly lower than those of male counterparts (MA:FA = 1.00±0.18:0.48±0.12, p<0.01; MR:FR = 1.05±0.22:0.22±0.03, p<0.01; Fig. 2E). The levels of 17β-HSD transcripts in the BAT were significant lower compared to the other depots in both sexes (MA:MR:MB = 1.00±0.16:1.05±0.22:0.28±0.08, p<0.01; FA:FR:FB = 0.48±0.02:0.22±0.03:0.16±0.02, p<0.01; Fig. 2E). The CYP19 transcripts level in female abdominal adipose were significantly lower than those of male counterparts (MA:FA = 1.00±0.16:0.51±0.05, p<0.05; Fig. 2F). In male, the levels of CYP19 transcripts of reproductive adipose and BAT were significant lower compared to the abdominal adipose (MA:MR:MB = 1.00±0.16:047±0.18: 0.26±0.07, p<0.05, p<0.01, respectively; Fig. 2F). In female, the levels of CYP19 transcripts of BAT were significant lower compared to those of other depots (FA:FR:FB = 0.51±0.05:0.83±0.19:0.29±0.10, p<0.01; Fig. 2F).
In another sets of RT-PCR, we measured the transcriptional activities of estrogen receptor-alpha (ER-α), estrogen receptor-beta (ER-β) and luteinizing hormone receptor (LHR). All kinds of transcripts were successfully amplified. Similar to steroidogenic factors, the abundances of the receptor transcripts were generally lower in the BAT of both sexes. ER-β transcripts were more abundant in male WAT depots than their female counterparts (Fig. 3, A-C). The levels of ER-α transcripts in the BAT were significant lower compared to the other depots in both sexes (MA:MR:MB = 1.00±0.09:1.02±0.11:0.64±0.10, p<0.05; FA:FR:FB = 1.05± 0.13:1.11±0.13:0.55±0.07, p<0.05; Fig. 3A). The ER-β transcript levels in female abdominal adipose and reproductive adipose were significantly lower than those of male counterparts (MA:FA = 1.00±0.12:0.55±0.17, p<0.05; MR:FR = 1.29±0.13:0.21±0.07, p<0.01, respectively; Fig. 3B). In male, the levels of ER-β transcripts of BAT were significant lower compared to those of other depots (MA: MR:MB = 1.00±0.12:1.29±0.13:0.59±0.12, p<0.05; Fig. 3B). The levels of LHR transcripts in female reproductive adipose were significantly higher than those of male counterpart (MR:FR = 0.95±0.05:1.46±0.06, p<0.01; Fig. 3C). In comparison of the LHR transcript levels between depots of same sex, BAT shown significant low abundance (MA: MR:MB = 1.00±0.05:0.95±0.05:0.31±0.03, p<0.01; FA:FR:FB = 1.22±0.09:1.46±0.06:0.38±0.07, p<0.01; Fig. 3C).
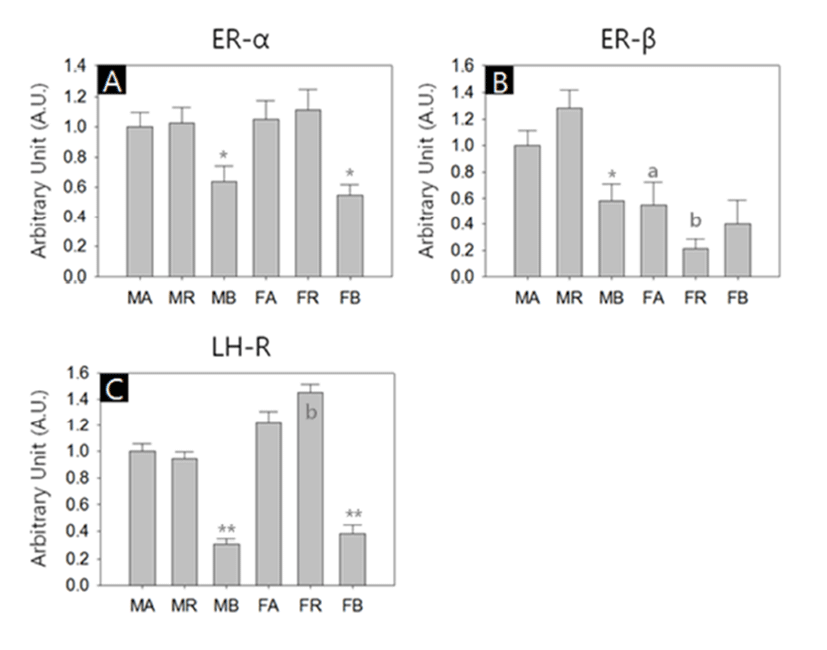
DISCUSSION
The obesity epidemic is one of most serious global issue and shows explosive growth without any sign of diminishing, while the cause and solutions remain unclear. Naturally, a large amount of research had been conducted to clarify the physiology of adipose tissues. For example, the influence of sex on adipose tissue distribution and function, and its implication in metabolic syndrome has been known for a long time (Mayes & Watson, 2004; Fuente-Martín et al., 2013). The distribution of fat in gynoid and android shapes of adult humans is a consequence of the close interrelationship of adipose tissue with androgens and estrogens (Kotani et al., 1994). On human cases, two studies revealed that there are clear differences between male female even before the onset of puberty. Sex differences in body fat distribution are present in prepubertal children but that the specific characteristics for Asians differ from African-Americans and Caucasians (He et al., 2002). The other study also demonstrated that sexual dimorphism in fat patterning is apparent prepubertally, and the magnitude of the sex difference is amplified with maturation, particularly from late puberty to early adulthood (Taylor et al., 2010). In the present study we used immature rats (PND 30), and found the sex-specific expressions of steroid synthesizing genes, generally higher in male animals.
Adipose tissues had been thought for a long time as a simple storage organ for triacylglycerol. However, view of adipose tissue dramatically changed to significant endocrine organ by the discovery of leptin in the mid-1990s (Pelleymounter et al., 1995). Indeed, adipose tissues synthesize and release not only leptin but numerous protein hormones, growth factors and cytokines, collectively known as ‘adipokines’, and steroid hormones (Li et al., 2015). Estrogens in men and in postmenopausal women are principally produced by adipose tissue from circulating steroid precursors (Grodin et al., 1973). Similar phenomenon, aromatization of androgens to estrogen, was found in castrated obese rats (Drago et al., 1982). Besides the conversion of various steroid precursors from circulation, recent studies revealed that the adipose tissues are equipped with steroidogenic machinery for de novo synthesis from cholesterol, initiated by StAR and CYP11A1 (Atzmon et al., 2002; MacKenzie et al., 2008b; Li et al., 2015). Our finding that adipose tissues express the StAR and steroidogenic enzymes in the pathway from cholesterol to active steroid hormones supports the de novo steroid synthesis in adipose tissues. Within hippocampus and cerebellum from normal and Alzheimer's disease patients, on the other hand, ten steroid-associated genes were transcribed except for CYP11B1 (11beta-hydroxylase), CYP11B2 (aldosterone synthase) and CYP17 (17alpha-hydroxylase) (MacKenzie et al., 2008a). Without these key mRNAs, human hippocampus and cerebellum rule out the de novo synthesis of aldosterone, cortisol or the sex steroids.
Two estrogen receptor isoforms, ER-α and ER-β, are present in mitochondria of WATs, suggesting important influences on adipocyte metabolism and proliferation (Pedersen et al., 2001; Dos Santos et al., 2010). In the present study, we found that the expressions of ER-α and ER-β in all adipose tissues of both sexes. ER-α expressions in BATs of both sexes were significantly less than those in the WATs. ER-β expressions in female abdominal and reproductive fats were significantly less than those of male counterparts. We speculate that the ER-β expression pattern could be a reflection of prepubertal sex differences. There is evidence that both ER-α and ER-β were expressed in human fetal brown adipose tissue(fBAT), with ERα being dominant (Velickovic et al., 2014). The authors demonstrated that ER-β was present only in mature brown adipocytes while ER-α was in mature adipocytes and preadipocytes, and suggested a dominant role for ER-α in its development and, fBAT mitochondria could be targeted by estrogens (Velickovic et al., 2014). Finally, there is a report on the HCG/LH receptor expression in human preadipocytes, and our data about LHR expression in rat adipose tissues confirm the finding (Dos Santos et al., 2007).
Special attention should be paid to transgender population when they undergo cross-sex hormone therapy. In transgender women, estrogen therapy, with or without antiandrogen therapy, was associated with lower blood pressure, while transgender men who take testosterone therapy were associated with increased body mass index (Deutsch et al., 2015). We speculate that long-term exogenous steroid supplements can induce a broad range of steroid-associated changes, including physiological and biochemical status of adipose tissues, and probably disturb body energy homeostasis and possibly cause unhealthy condition.
In conclusion, our study demonstrated that the expressions of steroidogenesis-related genes (StAR, enzymes and hormone receptors) were occurred in the immature male and female rat adipose tissues. The expression patterns were depotspecific and sex-specific, and particularly, overall expression intensities in brown adipose of both sexes shown lower than other depots. Taken together, our study suggested that the adipose tissues are not only targets but de novo synthesizing sites of sex steroid(s), though the synthesizing activities could be much less than in gonads. Further researches in this field will be helpful for understanding the adipose physiology and for medical application such as sex-specific steroid supplement therapies for older populations.