INTRODUCTION
Mammary glands are anatomical structures that distinguish mammals from all other animals and secrete milk for offspring. These organs are considered composite secretory organs and are made up of various types of cells, including epithelial cells, adipocytes, fibroblasts, and vascular endothelial cells (Macias & Hinck, 2012). There are three major stages of mammary gland development: embryonic, pubertal, and reproductive stages. In embryonic mammary gland development, the epithelial and surrounding stromal compartments derived from embryonic ectoderm and mesoderm are formed. The epithelial placodes of ectoderm formation during early embryo development give rise to the mammary gland structures during morphogenesis (Pispa & Thesleff, 2003). The placodes fundamentally regulate epithelial-mesenchymal interactions and generate the rudimentary ductal structure of the gland present at birth. The puberty and reproductive stages of mammary gland development occur continuously through hormonal regulation associated with pubertal growth, pre gnancy, lactation, and involution. At birth, the mammary gland is a rudimentary structure consisting of only a few small ducts. These small ducts can grow allometrically following the growth phase and undergo expansion, called ductal morphogenesis, until puberty. Puberty in mammals initiates morphogenesis of branched structures in the mammary gland; this process is mutually regulated by growth hormone (GH), estrogen, and insulin-like growth factor (IGF)- 1 to generate a ductal tree (Howlin et al., 2006). During pregnancy, alveoli of the mammary glands, which secrete milk, are generated through combined action of progesterone and prolactin (PRL). In the mature structure, short branched ducts are controlled by progesterone; however, alveologenesis occurs only during pregnancy through induction by PRL and progesterone. PRL continually stimulates this process until the lactogenesis stage. When the levels of progesterone and PRL increase in the serum, branched alveoli are developed, and adipose tissue and blood flow are increased. After achieving milk production, PRL is decreased, and involution, defined as remodeling back to the original adult state, occurs. In bovines, colostrum is secreted during late gestation and for the first few days after calving. In this review, we will focus on the IGF pathway and its role in developmental processes and involute interactions in bovine mammary glands components.
The IGF pathway consists of three ligands (IGF-I, IGFII, and insulin), their receptors (IGF-IR, IGF-IIR, and insulin receptor), high-affinity binding proteins (IGF binding protein [IGFBP] 1–6), and their specific proteases (Le Roith, 2003); the complex interactions among these components maintain cellular mechanisms. As shown in Fig. 1, these IGF ligands play various roles in mammalian growth, development, and metabolism and act as endocrine factors that prevent apoptotic signaling. Mammary epithelial cells are influenced by many components of the IGF system, particularly IGFBPs.
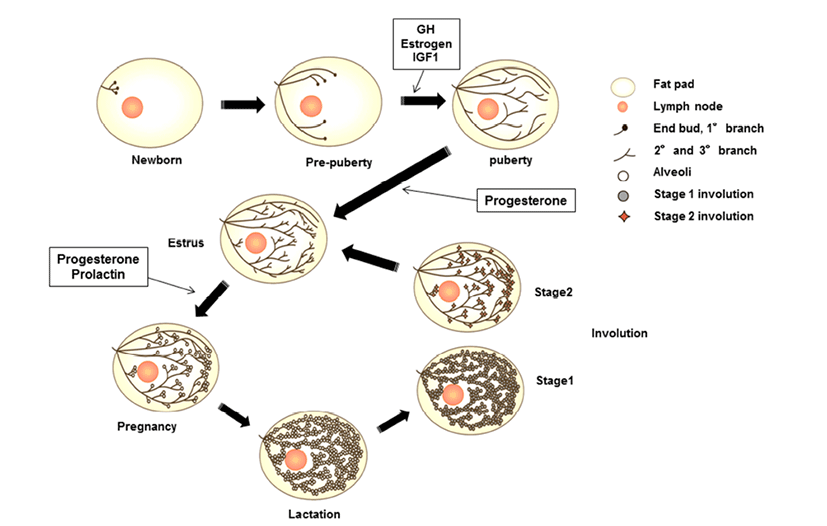
The interactions between IGFs and IGFBPs have been shown to inhibit IGF-I or IGF-II binding with IGF-IR and IGF-IIR through competitive mechanisms (Kelley et al., 1996). Bovine mammary epithelial cells have been reported to express all three IGF receptors (Hadsell et al., 1990), to synthesize IGFBPs (Gibson et al., 1999), and to produced IGF-II (Baumrucker et al., 1993). IGF-1 mRNA is expressed in mammary glands of pregnant and lactating cows (Glimm et al., 1992; Sharma et al., 1994), whereas cultured primary mammary gland cells do not exhibit IGF-1 expression. Recent studies have suggested that IGF-I plays a critical role in growth hormone (GH)-induced mammary ductal growth.
The IGF and GH interaction is important for regulation of mammalian epithelial cell proliferation and differentiation. IGF-I is circulated through its interaction with GH, which is the major inducer of alveolar growth (Kleinberg, 1997). There are correlations between IGF-I and GH expression, and these correlations may directly mediate mammary growth (Ruan et al., 1992). Additionally, GH has been shown to induce IGF-I expression in mammary tissue (Ruan et al., 1995). Furthermore, IGF-I can promote ductal development in implanted mammary glands and synergizes with estrogen to support the generation of terminal end buds (TEBs) and ductal elongation in ovariectomized mice (Ruan et al., 1995; Kleinberg, 1997). IGFs are potential mediator involved in the regulation of cell proliferation and apoptosis.
Several studies have reported that IGF-I and IGFR interactions are associated with cell proliferation and apoptosis through inhibition of Wnt signaling (Richard-Parpaillon et al., 2002; Rota & Wood, 2015). IGF may specifically influence IGF-1R owing to its greater binding affinity than IGF-II or insulin (Jones & Clemmons, 1995). Moreover, IGF-IIR binds more specifically to IGF-II than IGF-I and does not bind with insulin (Massague et al., 1982; Rechler & Nissley, 1985). In general, mammary gland development is characterized by ductal growth in nonpregnant heifers. While IGF-I is highly expressed during the early stages of mammogenesis when compared with that during lactation, this protein exhibits potential proliferative functions throughout the entire process of bovine mammary gland development. Additionally, IGF-1R and GH receptor mRNA expression levels are decreased during the lactation stage. These decreases in mRNA levels in bovine mammary tissue have been shown to be species specific (Plath-Gabler et al., 2001).
Different types of IGF-1R transcripts have been discovered in bovine mammary glands (Glimm et al., 1992). IGFBPs, which are encoded by six similar transcripts, are regulated based on their interactions with IGFs and their specific binding proteins (Ballard et al., 1989). The interactions of IGFBPs with IGF have been characterized in several studies. IGFBP bioactivity inhibits the functions of IGF-I and IGF-II by competition with IGF-IR and IGF-IIR through IGF-I and IGF-II near the cell surface. Furthermore, IGFs and IGFBPs can influence interactions with other growth pathways or IGF-independent functions (Kelley et al., 1996; Werner & LeRoith, 1996). The interactions between IGFBPs and IGFs are regulated by proteases by affecting the binding affinity of IGFBP for IGFs.
Modifications of IGFBPs, such as protein phosphorylation and binding with other factors, may affect their interactions with other proteins (McCusker et al., 1990; Oh, 1998). For example, when IGFBP-1 binds to integrin, IGFBP-3 binding at the cell surface is decreased because the specific affinity of IGFBP-3 for IGF-1 is reduced when IGFBP-3 interacts with cell surface molecules (Conover & Powell, 1991). Moreover, suppression of IGF-I release from IGFBP-3 may occur through the interaction between IGFs and IGFIR, thus reducing the activity of IGFs. IGFBP expression during mammary gland development has also been studied by analysis of the contributions of IGFBP-2 and -5 to branching mammogenesis during pregnancy (Wood & Yee, 2000).
All IGFBPs exhibit high-affinity binding with IGFs; however, IGFBPs also have other functions independent of IGFs. IGFBP-1 and -2 include the tripeptide Arg-Gly-Asp (RGD) sequence, which is recognized by cell surface integrins and cell adhesion molecules. IGFBP-1 overexpression in Chinese hamster cells increases cell migration (Jones et al., 1993). Additionally, IGFBP-3 has independent effects on the modulation of Bax and Bcl-2, inducing apoptosis in human breast cancer cells through IGF-I (Butt et al., 2000) and inhibiting proliferation in type I IGF receptor-knockout mice (Valentinis et al., 1995). Recent studies have demonstrated that IGFBP-3 regulates transforming growth factor (TGF)-β during programmed cell death through interactions between p53 and IGF-independent signaling (Rajah et al., 1997). Additionally, the TGF-β receptor can interact with IGFBP-3 (Leal et al., 1997). Moreover, IGFBP-5 has been shown to activate DNA synthesis in osteoblasts through a pathway independent of IGF-I (Andress, 1995). Both IGFBP-3 and -5 contain a putative nuclearlocalization sequence (NLS) (Radulescu, 1994) and have been shown to be expressed in the nuclei using immunelogical localization methods (Jaques et al., 1997; Schedlich et al., 2000). These results suggest that IGFBPs can also contribute to intracellular functions, such as proliferation, apoptosis, and cell migration. Thus, these observations also provide insights into whether the high concentrations of IGFBP-5 in bovine milk interact with IGF-dependent or -independent pathways. In human milk production, IGFBP- 2 is more highly expressed than IGFBP-1 and IGFBP-3 (Erickson et al., 1993; Donovan & Odle, 1994), and IGFBP-1 and IGFBP-3 are present at lower concentrations than IGFBP-2 in human milk. Porcine milk contains IGFBP-1– 4. mRNAs encoding IGFBP-2 and -3 are expressed in porcine mammary glands (Simmen et al., 1988). Thus, IGFBP-2 and - 3 in milk is derived from the mammary glands. In bovine serum, IGFBP-2 is highly expressed during the early lactation stage and decreased during the dry period (Vicini et al., 1991). Rat milk contains IGFBP-2–4, as detected by western blotting. IGFBP-2 is not expressed in maternal serum, but is expressed in lactating mammary glands, suggesting that IGFBP-2 is synthesized in mammary glands. IGFBP-4 expression has been identified in rat mammary glands, although the concentration is lower than that in serum (Donovan et al., 1995). IGFBP-5 and -6 are not present in milk during the lactation stage. IGFs and IGFBPs may enter milk from the serum by paracellular interactions and secretion by epithelial cells. However, involuting mammary glands exhibit increased levels of IGFBP-2, IGFBP-4, and IGFBP-5 mRNAs. IGF-1 is a survival factor for several different types of cells and is regulated by GH (Travers et al., 1996). Of all the hormonal changes that occur during the involution stage, PRL is able to influence only IGFBP-5 synthesis, suggesting that the prolactin is a potential inhibitor of IGFBP-5.
CONCLUSION
In bovine mammary glands, mammary gland development occurs through complex regulation of the IGF pathway. Although IGF pathway ligands (IGF-I, IGF-II, and insulin) affect various cellular mechanisms, including proliferation, migration, and apoptosis, the roles of these ligands in mammary gland development depend on associations with their specific receptors, e.g., IGFBPs and IGF-R. Fig. 2 describes the general interactions among IGFBPs, ligands, and their specific receptors in mammary epithelial cells. IGFs cannot interact with their receptors when IGF-I and IGF-II bind to IGFBPs owing to competitive interactions between IGF and IGFBPs. IGFBPs are degraded by proteases, whereas IGFs are free to bind with IGF receptors. IGFBPs are present in bovine serum, whereas IGFBPs are expressed in mammary epithelial cells. In addition, IGFBPs are secreted in bovine milk derived from mammary gland epithelial cells. Furthermore, at the involution stage, IGFBPs are decreased in milk and epithelial cells, along with PRL and GH, resulting in degradation of mammary glands. Mammary glands are considered highly sensitive to hormones and IGF signal transduction during the developmental stage. Therefore, improving our understanding of and IGFBP interactions and secretion during mammary gland development may facilitate increased milk productivity through induction of mammogenesis.
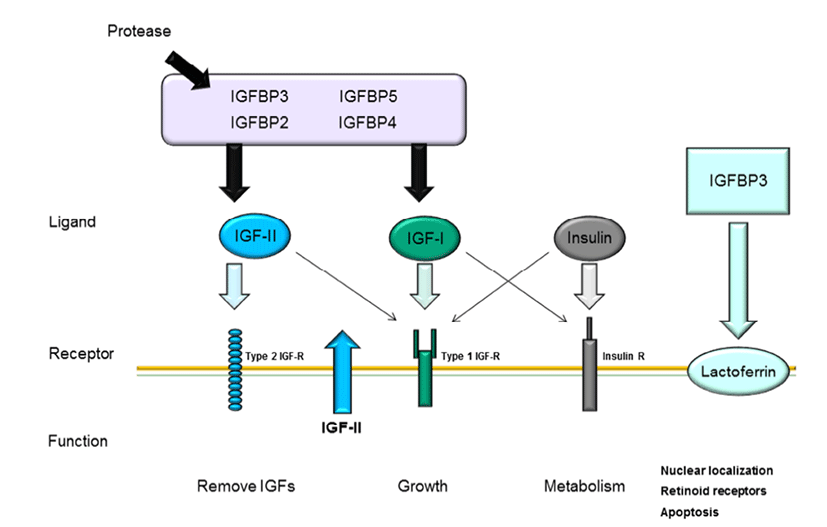