INTRODUCTION
Plk is a serine/threonine kinase, which was firstly identified in Drosophila as Polo mutant over 27 years ago (Sunkel & Glover, 1988). This kinase is well conserved from budding yeast (Cdc5) to Drosophila (polo), Xenopus (Plxs) and mammals (Plks) (de Carcer et al., 2011a). In mammalian cells, it has been reported that four Plk family members, from Plk1 to Plk4, are expressed and recently Plk5 has been characterized in the mouse and human cells (de Carcer et al., 2011a). Plks are key regulators for various cellular events during cell division including centrosome maturation, DNA checkpoint activation, mitotic entry, spindle assembly, and cytokinesis (Fig. 1) (Glover et al., 1998; Dai, 2005; Strebhardt & Ullrich, 2006).
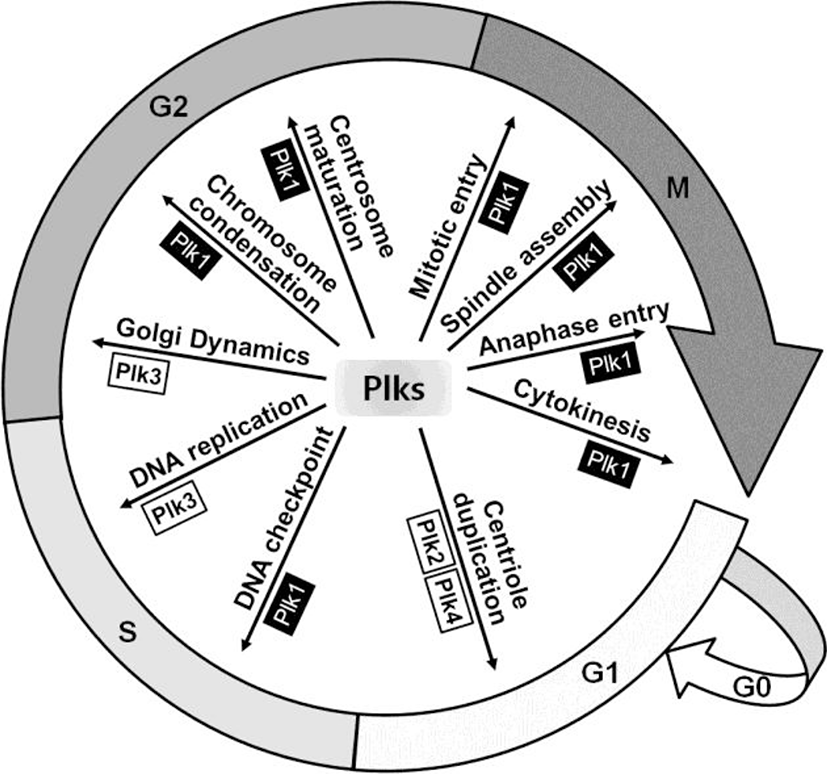
Plk1 has a crucial role in the regulation of mitotic checkpoints. Hence, overexpression of this kinase causes overriding checkpoints, which can lead to immature cell division with aneuploidy, a hallmark of cancer (Kops et al., 2005). Plk1 overexpression contributes to cancer development by promoting excessive cell proliferation through the misregulation of check point functions (Degenhardt & Lampkin, 2010). Furthermore, Plk2 and Plk3 also have a relationship with p53 and are activated by DNA damage in mitosis, whereas Plk1 is inhibited (Jang et al., 2007). Plk2 is a direct transcriptional target of p53 and Plk3-mediated phosphorylation of p53 at Ser-20 is reported to stabilize p53 (Xie et al., 2001; Burns et al., 2003). Therefore, Plk families might be a good target for cancer therapy and several Plk inhibitors are in clinical studies recently. In this article, we review characteristics and functions of Plk family members in regard with cell cycles, carcinogenesis, and apoptosis, as well as with the possibilities of Plks as cancer therapeutic targets.
The Plks have a highly conserved N-terminal catalytic domain and C-terminal domain with one or more polo box domains (PBDs) (Fig. 2) (Strebhardt & Ullrich, 2006). In the yeast and Drosophila, only a single Plk gene has been identified to date, while the higher vertebrates have multiple Plk genes of which Plk1 is most similar to Drosophila polo (Ohkura et al., 1995). Plk2 (also known as SNK) localizes at the centrosomes and plays a role in the entry into S phase (de Carcer et al., 2011a). Inhibition of Plk2 resulted in formation of aberrant number of centrioles (Warnke et al., 2004). Plk3 (also named FNK or PRK) is required at the G1-S phase transition and for DNA replication (Zimmerman & Erikson, 2007). Plk4 (also known as SAK23) is found in all animals and it acts as a critical regulator of centriole duplication both in Drosophila and mammals (Kleylein-Sohn et al., 2007). Both the mouse and human Plk5 genes encode proteins, however, to some extent they are different that the mouse Plk5 contains a complete kinase domain and PBD, while the human Plk5 encloses only a small portion of the kinase domain along with one PBD (de Carcer et al., 2011b).
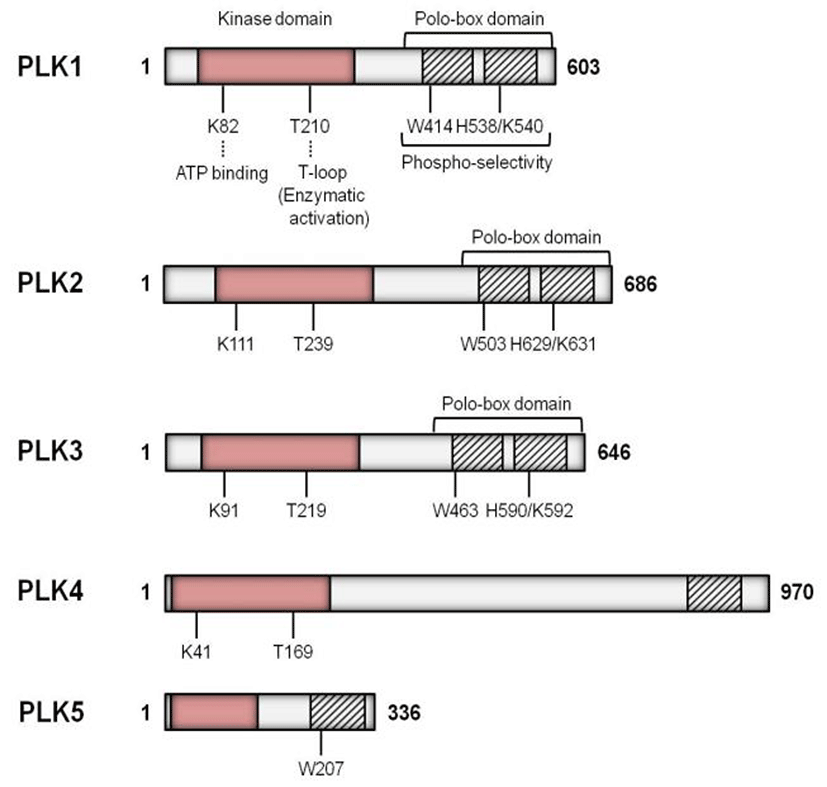
Mammalian Plks are required for the functional matu-ration of centrosomes and the assembly of a bipolar spindle. Microinjection of Plk1-specific antibody resulted in abnormal chromatin and microtubules, and hence in block of cell division (Lane & Nigg, 1996). These results were derived from small, unseparated centrosome. Shortly before the onset of mitosis, the size and number of centrosomes increase and they are required for organizing the microtubules (Karsenti et al., 1984). The functional maturation of centrosomes can be visualized by monitoring the accumulation of multiple proteins including γ-tubulin and M-phase-specific phosphoprotein (MPM-2) reactive phosphoepitopes (Lajoie-Mazenc et al., 1994). Plk1 anti-body-injected cells showed much smaller mitotic spindle poles and reduced expression of γ-tubulin and MPM-2 (Lane & Nigg, 1996).
Through recent studies, γ-tubulin is thought to be a critical element in centrosomal microtubule nucleation during late G2/early prophase (Shu & Joshi, 1995; Sunkel et al., 1995). Plk1 depletion or inhibition prevented the recruitment of γ-tubulin to centrosomes (Lane & Nigg, 1996; Haren et al., 2009). In addition, the accumulation of MPM-2 reactivity at centrosomes is inhibited in Plk1-injectied cells (Lane & Nigg, 1996).
In the previous study, it has been shown that the removal of centrosomes from mammalian cells in culture prevented every part of features of mitosis, including the activation of Cdc2 kinase (Maniotis & Schliwa, 1991). Cdc25C is an activator of Cdc2 kinase and dephosphorylates and activates the CyclinB-Cdc2 complex shortly before the entry into the mitosis. Both the DNA damage and DNA replication checkpoints regulate CyclinB-Cdc2 activation partly through Cdc25C. Plx1, Xenopus homolog of Plk, can phosphorylate and activate Cdc25C in vitro. In Xenopus oocyte maturation, Plx1 is activated in vivo with the same kinetics as Cdc25C (Qian et al., 1998). Depletion of Plx1 activity in Xenopus oocytes significantly inhibited the activation of Cdc25C and CyclinB-Cdc2 and inhibition of Plx1 in early Xenopus embryos resulted in an arrest of cleavage with abnormal spindles (Qian et al., 1998). These studies demonstrated that Plx1 plays important roles both in the activation of Cdc25C in the mitosis as well as in meiosis.
Through in vitro study in mammalian cells and Drosophila system, it has been known that Plk1 is required for important steps in the onset and completion of mitosis, such as bipolar spindle assembly, segregation of chromosomal DNA (Ohkura et al., 1995), centrosome maturation (Lane & Nigg, 1996) and anaphase promoting complex regulation (Descombes & Nigg, 1998). Recently, nuclear distribution gene C (NudC) and centrosomal ninein-like protein (Nlp) have been identified as Plk1 target proteins (Zhou et al., 2003). Plk1 phosphorylates NudC and regulates cytokinesis (Zhou et al., 2003). Nlp, a substrate of Plk1, interacts with the γ-tubulin ring complex and stimulates microtubule nucleation (Casenghi et al., 2003).
Plk2 is expressed in mainly G1 phase and important for the reproduction of centrosomes and centriole duplication (Cizmecioglu et al., 2008; Cizmecioglu et al., 2012). Mutation in the Plk2 polo-box inhibits normal localization of centrosome and centriole duplication (Cizmecioglu et al., 2008). Interestingly, Plk2 and Plk4 have a functional interaction and Plk2 mediated centriole duplication is associated with a Plk4 activity (Cizmecioglu et al., 2008). In mouse, depletion of Plk2 impairs embryonic growth and normal placental development resulted from decreased cell proliferation (Ma et al., 2003).
Plk3 is also a key regulator of cell cycles during mitosis. In S phase, Plk3 regulates DNA replication with phosphorylation of related proteins, topoisomerase IIα and DNA polymerase δ (Iida et al., 2008). In addition, Plk3 regulates Golgi dynamics during G2 phase for mitotic entry in mitotic cells (Lopez-Sanchez et al., 2009).
It has been well known that Plk1 expression increased in various cancer tissues such as non small cell lung cancer (Wolf et al., 1997), squamous cell carcinoma of the head and neck (Knecht et al., 1999), esophageal cancer, gastric cancer (Tokumitsu et al., 1999) and colorectal cancer (Macmillan et al., 2001). In Plk1 siRNA-treated HeLa cells, the cell proliferation was dramatically inhibited whereas the cell viability decreased (Liu & Erikson, 2003). Especially, apoptosis was induced in Plk1-depleted cancer cells (Liu & Erikson, 2003). These results demonstrate that Plk1 is related to the causes of cancer such as abnormal centrosome formation with abnormal cell cycles and apoptosis.
It is well known that centrosomes are critical in causing genetic instability in cancer cells (Brinkley, 2001). They cause the abnormalities in spindles and disturb the chromosome segregation, which are found with reflective alterations in key cellular functions, such as regulation of apoptosis, control of cell cycles, and regulation of cell growth. Centrosomal abnormalities have been detected in early forms of the human prostate cancer (Pihan et al., 2001). Extra centrosomes in cancer cells may lead to chromosome mis-arrangements and damages which are causes of aneuploidy. This condition may induce the loss of tumor suppressor genes or activate oncogenes (Spankuch-Schmitt et al., 2002). Therefore those authors suggested that the centrosomes are driving force for cancer formation, not a consequence of it.
Plk1 is also associates with apoptosis. Fas-associated death domain-containing protein (FADD) was identified as a first adapter molecule that bridges the Fas-receptor during apoptosis (Peter et al., 1999). The phosphorylation of FADD serves as a critical marker for the human cancer diagnosis (Shimada et al., 2005). Plk1 phosphorylates FADD at Ser-194 in response to taxol treatment and is regulated through a negative feedback loop by its substrate, FADD (Jang et al., 2011). In addition, Plk1 binds to the major tumor suppressor gene, p53, in cultured mammalian cells, and pro-apoptotic function of p53 is vanished via interaction with Plk1 (Ando et al., 2004). Inhibition of Plk1 function prevented centrosome maturation and induced cell death in carcinoma cells and dominant negative Plk1 induced tumor-selective apoptosis in tumor cell lines (Lane & Nigg, 1996). Overexpression of Plk3 suppressed cell proliferation and induced apoptosis (Conn et al., 2000).
The precise role of Plk1 in cancer cell is not apparent yet. However, based on the above findings, Plk1 has a critical role in the development of cancer. Therefore, regulation of Plk1 function might be an important appliance in cancer therapy. Because Plk1 activates Cyclin B/Cdc2 complex via Cdc25C and stimulates cell proliferation, the silencing of Plk1 will result in an inactivation of Cyclin B/Cdc2 complex-mediated mitotic arrest followed by apoptosis. In Plk1 siRNA-treated cells, cell proliferation was reduced and apoptosis was increased (Liu & Erikson, 2003). Therefore, pharmacological inhibitors of Plk1 may be valuable tools for treatment of cancer (Degenhardt & Lampkin, 2010; McInnes & Wyatt, 2011).
The fact that centrosome abnormalities in mitotic cells may lead to chromosome missorting and damage causing cancer suggests the possibility that Plk1, a key regulator of centrosome maturation, might regulate carcinogenesis and can be used as a therapeutic target for cancer treatment. In many clinical researches, Plk1 expression was enhanced in the human cancer cells, but detailed mechanism of Plk1 in cancer development is not clearly understood yet. Nowadays, Plk1 is emerging as an attractive target for anticancer agents. Furthermore, the collected information about the functions of the other Plk families suggests that we can now selectively inhibit the Plk1 activity without affecting other isoforms. Therefore, the more scrutinized research on each Plk family member and its regulation in normal mitotic as well as in meiotic cells may enlarge our knowledge in cell biology and also provide us an opportunity to find out the breakthrough for cancer treatment.