INTRODUCTION
Nucleobindin precursor protein, which was identified for the first time in the human and mouse cell lines, is known to have two isotypes, nucleobindin 1 (NUCB1) and nucleobindin 2 (NUCB2) (Miura et al., 1992; Kanai & Tanuma, 1992; Barnikol-Watanabe et al., 1994). This precursor protein contains multiple functional domains including a signal peptide on the N-terminal side, a leucine/isoleucine rich region, a DNA binding domain and a putative nuclear targeting signal, while the second half contains two Ca2+-EF-hand motifs and a leucine zipper motif in the C-terminal region (Barnikol-Watanabe et al., 1994). However, NUCB2 only functions physiologically in humans and rodents (Miura et al., 1992). NUCB2 produces nesfatin-1 (residues 1-82), nesfatin-2 (residues 85-163), and nesfatin-3 (residues 166-396) by the enzyme pro-hormone convertase (PC)-1/3 after putative post-translational processing. Until now, a physiological activity has only been demonstrated for nesfatin-1 (Oh-I et al., 2006; Stengel et al., 2012a). The nesfatin-1 molecule is composed of three domains: N-terminal (N23), middle part (M30) and C-terminal (C29). The M30 active core appears to play the key role in the induction of physiological effects of this peptide, and especially in its anorectic responses (Pałasz et al., 2012).
Central Expression of Nesfatin-1
Nesfatin-1 is initially known to be expressed in the rat hypothalamic nuclei for ingestive behavior regulation such as the paraventricular nucleus of the hypothalamus (PVN), supraoptic nucleus (SO), arcuate nucleus (ARC), lateral hypothalamic area (LHA), zona incerta and the nucleus of the solitary tract in rats (Oh-I et al., 2006). Furthermore, nesfatin-1 also exists in the additional hypothalamic nuclei, pituitary, cerebrospinal fluid and brain stem, including rostral raphe pallidus (rRPa), Edinger-Westphal nucleus (EW), locus coeruleus (LC), ventrolateral medulla (VLM) and the dorsal motor nucleus of the vagus nerve (Brailoiu et al., 2007; Chen et al., 2012; Foo et al., 2008; Fort et al., 2008; Goebel et al., 2009a; Goebel et al., 2011a; Kohno et al., 2008; Stengel et al., 2009c; Stengel et al., 2010b; Xu et al., 2009). Interestingly, nesfatin-1 immunoreactive neurons co-localize with a number of brain transmitters, such as the melanin-concentrating hormone (MCH), cocaine-and amphetamine-regulated transcript (CART)/pro-opiomelanocortin (POMC), α-melanocyte-stimulating hormone (α-MSH), mammalian target of rapamycin (m-TOR), vasopressin, oxytocin, neuropeptide Y (NPY), Agouti-related protein (AgRP), somatostatin, growth hormone-releasing hormone (GHRH), thyrotropinreleasing hormone (TRH), corticotropin-releasing factor (CRF) and neurotensin (Brailoiu et al., 2007; Foo et al., 2008; Fort et al., 2008; Inhoff et al., 2010; Kohno et al., 2008; Li et al., 2012; Maejima et al., 2009; Okere et al., 2010).
The various studies were performed about the central action of nesfatin-1. The initial report showed that the post third ventricular (3v) or fourth ventricle (4v) injection of nesfatin-1 decreases dark phase food intake in freely fed rats (Oh-I et al., 2006). Similarly, injection of nesfatin-1 into the lateral brain ventricle (intracerebroventricular, icv) of ad libitum fed rats reduced the dark phase food intake and controlled the water drinking (Shimizu et al., 2009a; Stengel et al., 2009a; Stengel et al., 2010a; Yosten & Samson, 2009; Yosten et al., 2012). Likewise, microinjection of nesfatin-1 directly into the PVN also suppresses the dark phase food intake in male rats (Maejima et al., 2009; Stengel et al., 2009a).
Nesfatin-1, which is released from the POMC/CART neurons, directly inhibits the NPY/AgRP neurons, causing their hyperpolarization, through the ATP-dependent potassium channels Kir6.2. There are some suggestions that the hyperpolarization of orexigenic ARC neurons can be a key phenomenon in anorexia, induced by nesfatin-1 (Price et al., 2008). Another study provided compelling evidence for the involvement of an oxytocin pathway in nesfatin-1’s inhibitory effect on food intake. An oxytocin receptor antagonist injected into the hindbrain at the level of the 4v blocked the food intake reducing effect of nesfatin-1 injected into the PVN (Maejima et al., 2009). Likewise, an oxytocin antagonist injected icv blocks the food intake suppressing effects of icv nesfatin-1 and α-MSH (Yosten & Samson, 2010). On the other hand, these pathway of nesfatin-1’s anorexigenic action is independently with central leptin signaling as suggested by the finding that nesfatin-1’s central food intake reducing effect is retained in leptin receptor deficient rats (Oh-I et al., 2006).
Some studies demonstrated that central nesfatin-1 may play a role in the response to stress, such as abdominal surgery, inflammatory stimulus, intraperitoneal injection of lipopolysaccharide and eating disorder (Bonnet et al., 2009; Dayas et al., 1999; Goebel et al., 2009b; Merali et al., 2008; Okere et al., 2010; Stengel et al., 2010b; Stengel et al., 2011; Xu et al., 2010). These findings are based on the result of that icv nesfatin-1 exerts a CRF receptor-dependent effect in food intake and the central distribution in forebrain, hindbrain and spinal autonomic nuclei, along with the expression of nesfatin-1 in CRF neuron (Foo et al., 2008; Stengel et al., 2009a). In addition, the brain activation by stress is associated with the co-localization of nesfatin-1 immunoreactive neurons and brain transmitters, such as a neurotensin, urocortin and serotonin (Stengel et al., 2009a).
Recent two studies reported that effects of nesfatin-1 as an anti-inflammatory and anti-apoptotic peptide in subarachnoid hemorrhage (SAH)-induced rats (O¨zsavci et al., 2011; Tang et al., 2012). These findings suggest that nesfatin-1 exerts neuroprotection in SAH-induced injury in rats by inhibiting neutrophil infiltration and nuclear factor kappa-B-dependent inflammatory responses. Furthermore, nesfatin-1 may lessen caspase-3-mediated neuronal cell apoptosis after traumatic brain injury (O¨zsavci et al., 2011; Tang et al., 2012).
Peripheral Expression of Nesfatin-1
Large amounts of the nesfatin-1 expression were detected in the digestive organs as well as the hypothalamus and brainstem. In particular, nesfatin-1 is markedly expressed in the pancreas, stomach and duodenum of rodent and canine (Gonkowski et al., 2012; Iwasaki et al., 2012; Mohan & Unniappan, 2012; Osaki et al., 2012; Stengel et al., 2009c; Stengel & Taché, 2012; Zhang et al., 2010).
In the pancreas, nesfatin-1 is located in rat islet betacells (Gonzalez et al., 2009) and secreted by high glucose stimulation in vitro (Foo et al., 2010; Gonzalez et al., 2011a). A certain study has reported that intravenous administration of nesfatin-1 has anti-hyperglycemic effects in hyperglycemic db/db mice (Su et al., 2010). However, this reduction in blood glucose levels was not observed in non-hyperglycemic animals and appears to be insulindependent (Gonzalez et al., 2012a; Su et al., 2010). Furthermore, nesfatin-1 enhances glucose-stimulated insulin secretion (GSIS) in mouse pancreatic beta-cells and rat pancreatic islets (Gonzalez et al., 2011b; Gonzalez et al., 2012a; Nakata et al., 2011). This effect is known to be mediated by an increase in intracellular calcium through L-type calcium channels (Nakata et al., 2011). Both calcium free saline and the L-type calcium channel blocker, nitrendipine, inhibit nesfatin-1 potentiation of insulin secretion in the presence of glucose. The mechanism by which nesfatin-1 enhances GSIS is unclear, however, it may be independent of the signaling pathways of glucagon-like peptide-1 (GLP-1), GIP and pituitary adenylate cyclase-activating polypeptide (PACAP) (Nakata et al., 2011). In accordance with the glucose-dependent insulinotropic effects of nesfatin-1 in vitro, an oral glucose tolerance test in ad libitum fed rats infused with nesfatin-1 produced significant increases in circulating insulin levels (Gonzalez et al., 2011a; Gonzalez et al., 2012a). Most recently, a certain paper reported that the icv nesfatin-1 results in increased insulin signaling through Akt kinase (Akt)/AMP kinase (AMPK)/target of rapamycin complex (TORC) 2 pathway, suggesting a potential mechanism for increased insulin sensitivity (Yang et al., 2012). These reports provide the evidence that the presence of nesfatin-1 within the pancreatic islets may be associated with glucose homeostasis.
In addition, nesfatin-1 immunosignals are mainly localized in mucosal endocrine X/A-like cells of the stomach, within a distinct sub-population of vesicles different from those containing the orexigenic hormone ghrelin, suggesting differential regulation and release of ghrelin and nesfatin-1 (Li et al., 2012; Stengel et al., 2009c; Stengel & Taché, 2010). The fasting for 24 h decreases NUCB2 mRNA expression in gastric mucosa and significantly reduces nesfatin-1 plasma revels in rats (Stengel et al., 2009a). Furthermore, nesfatin-1, which is released from the synaptic endings of the vagus nerve, has an impact on secretory and motor activity of the gastrointestinal tract and regulates the course of digestive functions (Goebel et al., 2009a; Stengel et al., 2009b; Xia et al., 2012). Interestingly, a recent paper has been demonstrated that pretreatment with capsaicin (to block autonomic C fibers) abolished the food intake reduction caused by peripheral nesfatin-1 injection. These findings indicate a putative role of vagal afferents in peripheral nesfatin-1 signaling to the brain centers (Shimizu et al., 2009c).
To date, a few studies have focused on nesfatin-1 in the human digestive system. Circulating levels of nesfatin-1 were significantly reduced in fasted type-2-diabetic patients compared to healthy subjects and type-1-diabetic patients. In addition, intravenous infusion of glucose significantly elevated basal nesfatin-1 levels in healthy adults (Li et al., 2012). However, circulating nesfatin-1 levels were not altered under an oral glucose tolerance test (Tsuchiya et al., 2010). Interestingly, circulating nesfatin-1 levels were significantly lower in patients with restricting-type anorexia nervosa (Ogiso et al., 2011). These results demonstrate that nesfatin-1 expression in the digestive organs is regulated by nutritional status, suggesting a potential role of peripheral nesfatin-1 in energy metabolism.
Nesfatin-1 was detected in the adipose tissues of humans and rodents, showing that it was expressed more in the subcutaneous than in the visceral adipocytes (Ramanjaneya et al., 2010). In experiments with fat cell lines, nesfatin-1 expression increased exponentially during the process in which the fat progenitor cells differentiate into the mature fat cells (Ramanjaneya et al., 2010). The fact that high nesfatin-1 concentrations are found in the blood of people with high body mass index (BMI) suggests that nesfatin-1 secreted from fat may regulate food intake independently, rather than relying on leptin (Aydin et al., 2009; Li et al., 2010; Ogiso et al., 2011; Ramanjaneya et al., 2010; Shimizu et al., 2009b; Yamawaki et al., 2012). Most recent two reports showed that NUCB2 enhances the suppression of adipose differentiation as a positive modulator of epidermal growth factor (EGF)-dependent signals (Tagaya et al., 2012a; Tagaya et al., 2012b).
A few studies have demonstrated that nesfatin-1 protein are expressed in the heart, as a cardiac peptide (Angelone et al., 2012; Mimee et al., 2012; Yosten & Samson, 2009). Nesfatin-1 directly affects myocardial performance, possibly involving particulate guanylate cyclase-linked natriuretic peptide receptor A (pGC-linked NPR-A), pGC/the protein kinase G (PKG), and extracellular signalregulated kinases1/2 (ERK1/2) pathway (Mimee et al., 2012). In particular, nesfatin-1 protects the heart against ischemia/reperfusion (I/R) injury and this protection involves multiple prosurvival kinases such as PKC, ERK1/2, signal transducer and activator of transcription 3, and mitochondrial KATP channels (Mimee et al., 2012). These results pave the way to include nesfatin-1 in the neuroendocrine modulators of the cardiac function, also encouraging the clarification of its clinical potential in the presence of nutrition-dependent physio-pathologic cardiovascular diseases.
Expression of Nesfatin-1 in Reproductive System
To date, a few studies have focused on nesfatin-1 in the mouse reproductive system. It is not clear yet whether nesfatin-1 is expressed in the mouse reproductive system and if the binding site of nesfatin-1 protein exists in the reproductive organs. Several studies demonstrated so far that the nesfatin-1 expression and its binding sites are found in the male and female reproductive organs.
Several recent studies showed the expression of nesfatin-1 in the male reproductive system of human and rodent (García-Galiano et al., 2010; García-Galiano et al., 2012; Kim et al., 2011a). In the testis, nesfatin-1 was localized in the interstitial cells, including the Leydig cells between the seminiferous tubules (Fig. 1A). Leydig cells produce and secrete testosterone, androstenedione, and dehydroepiandrosterone (DHEA) by cholesterol desmolase by which cholesterol changes to pregnenolone after stimulation of LH secreted from the pituitary (Akingbemi et al., 2004). LH receptors on Leydig cell membrane play a critical role in the steroidogenesis and the number of them is increased by prolactin resulting increasing LH-mediated response (Dufau, 1988). Leydig cells are also known to produce oxytocin and vitamin C (Kukucka et al., 1992), suggesting that they may play as local regulators to control the function of Leydig cells. The fact that Leydig cells express nesfatin-1 protein proposes that nesfatin-1 also has an important role in testicular function as one of local regulators similar to other proteins present in the testis. On the other hand, nesfatin-1 binding sites were displayed at boundary cells in the tunica albuginea of the testis (Fig. 1B). These results support that paracrine signaling by nesfatin-1 between the Leydig cells and the boundary cells of tunica albuginea may regulate the steroidogenesis and spermatogenesis. In addition, we demonstrated that large amounts of nesfatin-1 expression were detected in the mouse epididymis as well as the testis (Kim et al., 2011a). Immunohistochemistry results displayed that nesfatin-1 was expressed at the columnar epithelium (Fig. 2A). Nesfatin-1 binding sites were also localized at the columnar epithelium (Fig. 2B). The fact that columnar epithelial cells in the epididymis express both nesfatin-1 protein and its binding sites proposes that nesfatin-1 may play a role as a local regulator by autocrine signaling to regulate the sperm maturation and motility. Furthermore, nesfatin-1 expression levels of the testis and epididymis were increased by the gonadotropin injection (Kim et al., 2011a). This result demonstrates that nesfatin-1 produced in the male reproductive system is regulated by gonadotropin secreted by the pituitary like other proteins produced in the testis under gonadotropin control.
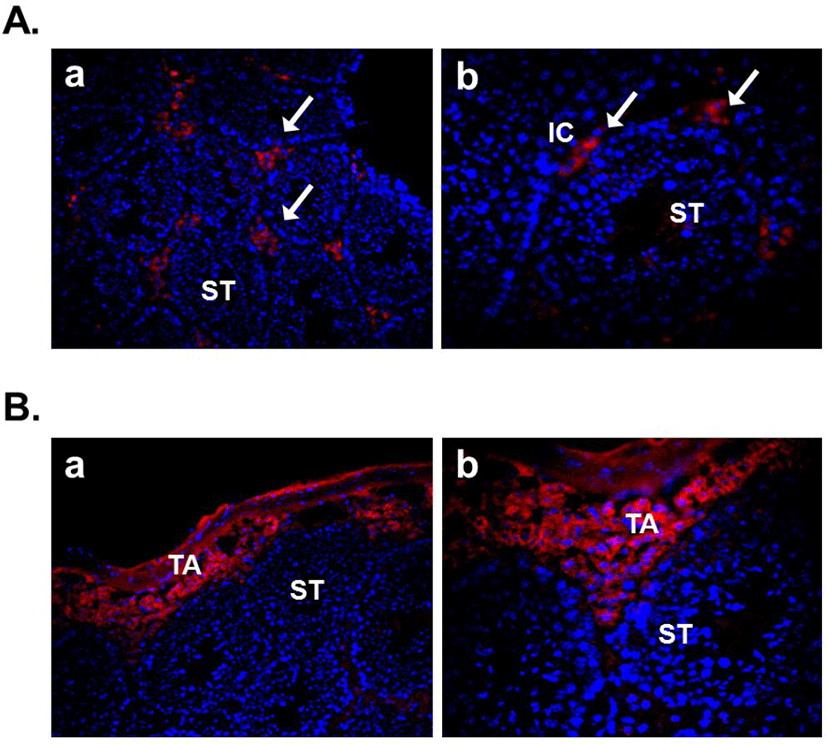
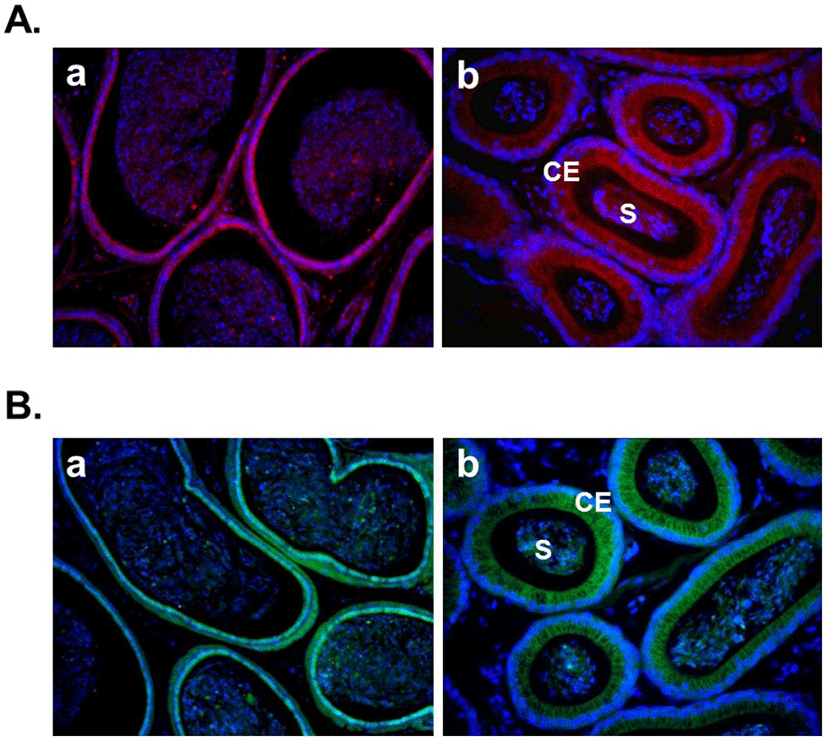
In this year, Gonzalez et al. (2012b) demonstrated the expression of nesfatin-1 protein in the fish ovary and hypothalamus, suggesting the regulatory effects of nesfatin-1 on the hypothalamo-pituitary-ovarian (HPO) axis of fish. In the ovary of goldfish and zebrafish, nesfatin-1-like immunoreactivity was detected in the follicle cells encircling the oocytes. In addition, a single intraperitoneal injection of synthetic goldfish nesfatin-1 resulted in an acute decrease in the expression of hypothalamic GnRH and serum LH levels (Gonzalez et al., 2012b). Furthermore, our previous papers have shown that nesfatin-1 and its binding sites are expressed in the female mouse reproductive organs (Kim et al., 2010; Kim et al., 2011b). In the ovary, nesfatin-1 was expressed in the theca cells, interstitial cells, and some of oocytes. Interestingly, the granulosa and cumulus cells around the oocytes were stained with nesfatin-1, but not the corpus luteum (Fig. 3A). On the other hand, nesfatin-1 binding sites were detected in the theca cells and interstitial cells around follicles (Fig. 3B). These results suggest that nesfatin-1 may play a role as a local regulator via autocrine and paracrine signaling to regulate the steroid-ogenesis and energy homeostasis in the ovary. In addition, nesfatin-1 was localized on the epithelial cells of endometrium and uterine glands in the uterus (Fig. 4A). Nesfatin-1 binding sites were displayed in the epithelial cells of uterine glands and neutrophils in the endometrium (Fig. 4B). Neutrophils are known to play an important role in the collapse and recovery of the endometrium (Kaitu'u-Lino et al., 2007). During estrous cycle, neutrophils move from blood into endometrium and the number of them are dramatically increased in the estrous phase (Sonoda et al., 1998; Steffl et al., 2010). Based on these reports, the finding that nesfatin-1 binding sites were localized on the neutrophils suggests that uterine function may be regulated by nesfatin-1 to attract neutrophils from blood into the endometrium and activate them. In addition, we previously demonstrated that nesfatin-1/NUCB2 expression in the uterus is regulated by estrogen, but not gonadotropin. Its expression levels were significantly increased in the ovariectomized mice treated with 17β-estradiol, whereas the mice treated with PMSG showed no difference compared to non-treated mice (Kim et al., 2011b). These results suggest that nesfatin-1/NUCB2 expression in the uterus may be regulated by estrogen secreted from the ovary, but not gonadotropin released by the pituitary gland.
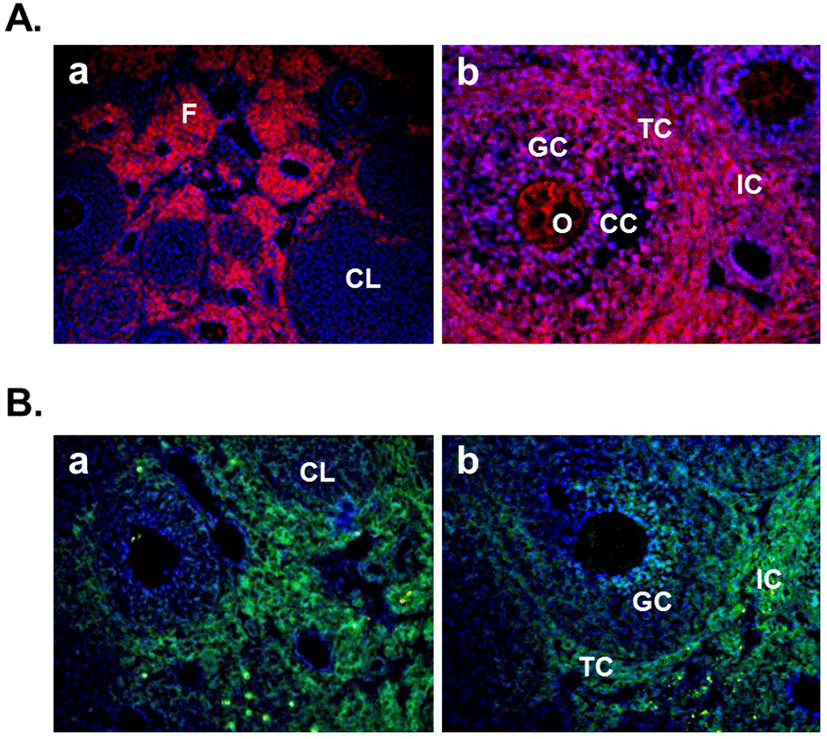
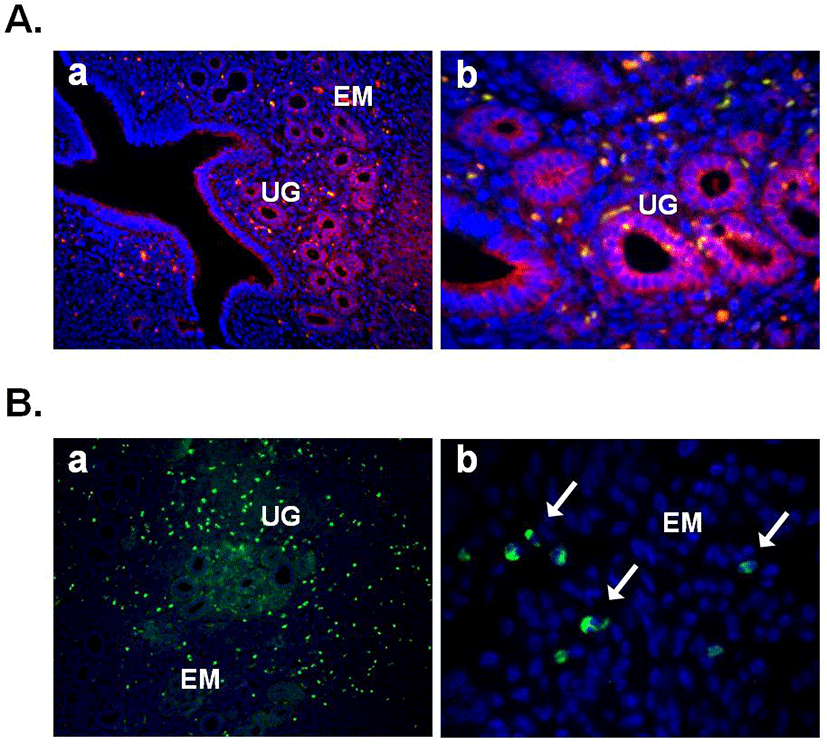
CONCLUSION
Nesfatin-1 protein produced by neurons in the hypothalamus is known to be secreted into cerebrospinal fluid, be controlling appetite and energy metabolism (Oh-I et al., 2006). Recently, it has been reported that nesfatin-1 is expressed in digestive organs such as the stomach and pancreas (Stengel et al., 2009c) and in adipose tissues (Ramanjaneya et al., 2010). To date, little is known about the expression of nesfatin-1 in reproductive system. However, several recent studies demonstrated that nesfatin-1 and its binding sites expressed in the reproductive organs, including testis, epididymis, ovary and uterus. These results suggest that nesfatin-1 as a new potent regulator in the male and female reproductive organs may regulate the steroidogenesis and gonadal function. Further study is needed in order to elucidate the exact function of nesfatin-1 in the reproductive system.