INTRODUCTION
Artificial sexual maturation of eel (Anguilla japonica) relies on rearing in seawater and injecting salmon pituitary extract (SPE). Salinity of the seawater and components of the SPE are known to influence hormonal activities of the eel pituitary, the regulatory center for reproduction, consequently resulting in the development of gonad (Kagawa et al., 1998). However, the exact mechanisms by which these external stimuli exert their effects remain unclear. These external stimuli could act either directly on the pituitary or indirectly through the hypothalamus with the help of hormonal substances or neurotransmitters.
Direct action of salinity change and SPE treatment on the pituitary can be proved by using the primary culture of pituitary cells. In vitro approaches have been successfully used to study gonadotropic hormone (GTH) expression in eel pituitary cells in response to various hormones and factors (Montero et al., 1996; Aroua et al., 2007; Pasquier et al., 2018; Yoon et al., 2023). Furthermore, cell culture technique provides a controlled in vitro environment, eliminating the confounding effects of various environmental factors and individual differences often encountered in vivo experiments (Ager-Wick et al., 2018).
Among the genes expressed in the pituitary, several genes have been implicated in sexual maturation and/or osmoregulation. GTH genes including luteinizing hormone (LHβ) and follicle stimulating hormone (FSHβ) are definite key genes for reproduction. Progesterone receptor-like (pgrl) plays a crucial role in oocyte maturation (Thomas et al., 2002), reflecting the broader influence of progesterone signaling on various female reproductive processes like follicle growth, ovulation, implantation and pregnancy maintenance (Chen et al., 2010). Prolactin (PRL) has been known as a “freshwater adapting hormone” in teleost (Breves et al., 2011), exhibiting diverse activities encompassing hydromineral balance, reproduction, growth and development, etc (Bole-Feysot et al., 1998). Dopamine and its receptors are important regulators of renal natriuresis, which is critical for the adaptation of many animals to changing environmental salinity (Su et al., 2016). Additionally, they exert direct inhibitory control on gonadotropes, counteracting the stimulatory effect of gonadotropin-releasing hormone (GnRH) on gonadotropin release (Dufour et al., 2005). Neuropeptide B/W receptor (NKBWR) is a receptor for Neuropeptide B (NPB) and Neuropeptide W (NPW). Studies in mammals have revealed that NPB/NPW plays a role in regulating various physiological processes, including food intake, energy homeostasis and stress (Mondal et al., 2003; Samson et al., 2004; Levine et al., 2005; Date et al., 2010). Relaxin family peptides and their receptors (rxfp1, rxfp2b, rxfp3-2a, and rxfp3-2b) exhibit diverse functions in mammals, including promoting growth, reproduction, and regulating renal and cardiovascular systems (Conrad & Novak, 2004; Sherwood, 2004).
In this study, we investigated the expression of LHβ, FSHβ, pgrl, PRL, dopamine receptor D4 (drd4), Neuropeptide B/W receptor 2 (NPBWR2) and relaxin family peptide receptor 3-2b (rxfp3-2b) genes to prove direct action of salinity change and SPE treatment on the pituitary using the primary culture of eel pituitary cells.
MATERIALS AND METHODS
Wild female eels (11 fish) were captured with a net in the vicinity of Lake Sapgyo and Lake Asan, Korea and transported to a fish rearing facility in Sunmoon university. Fish were treated with oxytetracycline (DaOne, Seoul, Korea) to prevent any possible bacterial disease transmission and anesthetized using 2-phenoxyethanol (0.5 mL/L freshwater) for subsequent measurements. Body length, body height, body circumference and body weight were recorded for each eel (Table 1). Blood was collected in a 3 mL heparinized syringe and centrifuged (2,000×g, 4°C, 5 min), and the plasma fraction was separated and stored −80°C until analysis. The eels were then dissected to obtain their livers, gonads and pituitaries. Liver weight was used to calculate the hepatosomatic index (HSI), while gonad weight was used for the gonadosomatic index (GSI). Finally, the pituitaries were carefully removed and stored in Hank’s Balanced Salt Solution (HBSS; Gibco, Grand Island, NY, USA) for use in cell culture experiments (Fig. 1). All fish maintenance and experimental procedures complied with the guidelines approved by the Animal Care and Use Committee of Sunmoon university and were conducted in accordance with established ethical principles for the care and utilization of laboratory animals (SM-2022-09-01).
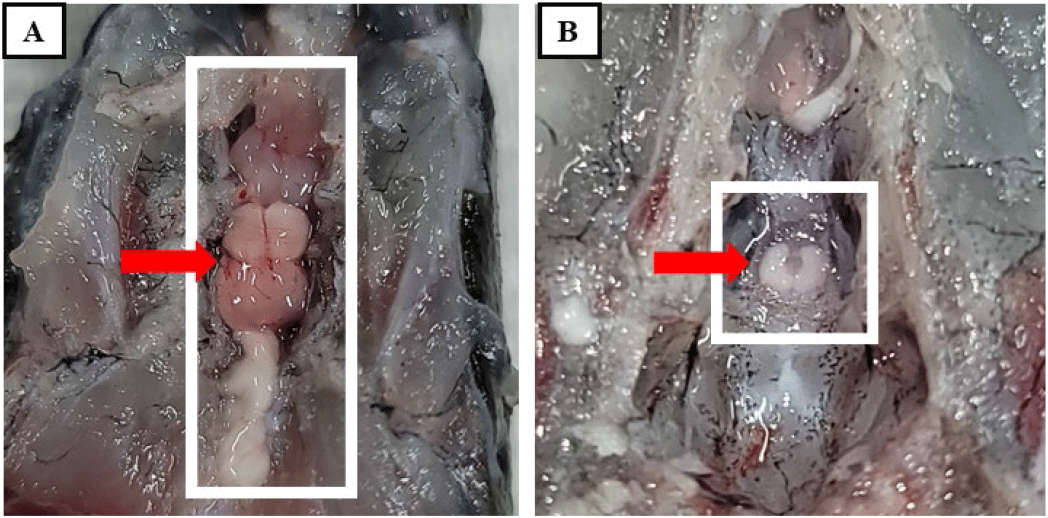
The primary culture of pituitary cell was performed with reference to Ager-Wick et al. (2018) and Mun et al. (2022). Briefly, the pituitary was removed from eels, washed with HBSS (Gibco) and then minced into small pieces using a razor blade. The chopped pituitaries were treated with 500 μL of trypsin- ethylenediaminetetraacetic acid (EDTA) solution (Gibco) and incubated at 27°C for 1 h. After incubation, trypsin-EDTA treatment was terminated by adding 50 μL of fetal bovine serum (FBS; Gibco). The cell suspension was then centrifuged (200×g, 4°C, 10 min) to separate the cell pellet from the supernatant, which was discarded. A culture medium containing 89% Leibovitz-15 medium (L-15; Gibco), 10% FBS, and 1% Penicillin-streptomycin (Pen-Strep, Gibco) was prepared, and the pituitary cells were suspended. After thorough pipetting, the number of cells and viability were determined using a hemocytometer and trypan blue, respectively. Poly-L-lysine solution (Sigma-Aldrich, St. Louis, MO, USA ) 200 μL was dispensed into each well of a 24-well plate and evenly coated on the bottom. The coating was performed at room temperature for approximately 2 h. The wells were then washed with sterile distilled water and dried in an incubator at 27°C for about 2 h. Cell suspension (1 mL) in culture medium was then dispensed into each coated well. To monitor cell attachment and stabilization, the cultures were incubated at 27°C for 4 days and observed under an inverted microscope (CKX53, Olympus, Japan) on days 1, 2, and 4. Subsequently, the cultures were divided into four experimental groups: control culture (control), SPE-treated culture (SPE), NaCl-treated culture (NaCl) and NaCl+SPE treated culture (NaCl+SPE). The culture medium for the NaCl groups (NaCl and NaCl+SPE) were treated with NaCl to reach a final concentration of 20 mM. To prepare the SPE solution for cell culture, 20 mg of SPE was suspended in 2 mL of distilled water. The mixture was then thoroughly homogenized. Following homogenization, the suspension was centrifuged (3,000×g, 4°C, 15 min) to separate the supernatant. Only the resulting supernatant, containing the dissolved SPE, was collected for further use. This supernatant was then mixed with the culture medium for SPE and NaCl+SPE, respectively. The culture medium was replaced with the corresponding treatment for each group and incubated for an additional 6 h at 27°C. Finally, the pituitary cells were collected for total RNA extraction.
Plasma osmolality of eels used to obtain the pituitary was measured using a Vapor Pressure Osmometer (Wescor 5600, Logan, UT, USA). In addition, to ensure keeping different osmolality of the culture medium between treatments during cell culture, the osmolality of the 4-experimental culture media (control, SPE, NaCl, and NaCl+SPE) was also measured at the end of experiment.
Total RNA was isolated from the pituitary cells treated with the different experimental media using Trizol® reagent (Ambion, Austin, TX, USA) according to the manufacturer’s instructions. The quantity of the extracted RNA was then determined using a Nanodrop 2000 spectrophotometer (Thermo Scientific, Massachusetts, MA, USA). Subsequently, complementary DNA (cDNA) was synthesized from the purified RNA using TOPscript™RT DryMIX (Enzynomics, Daejeon, Korea). Expression levels of target genes were assessed using quantitative real-time PCR (qRT-PCR) performed on a CFX96 Touch™ Real-Time PCR Detection System (Bio-Rad, Hercules, CA, USA) with Topreal™ qPCR 2X PreMIX SYBR Green (Enzynomics, Daejeon, Korea). For each sample, a 20 μL reaction mixture was prepared to contain 10 μL SYBR Green (Enzynomics), 5 μL cDNA (1:50 dilution) and 3 μL nuclease free water with the appropriate primers. The target genes analyzed were LHβ, FSHβ, pgrl, PRL, drd4, NPBWR2, and rxfp3-2b with primer sequences detailed in Table 2. The qRT-PCR reaction program consisted of an initial denaturation step at 95°C for 10 min, followed by 40 cycles of denaturation at 95°C for 15 sec, annealing at 60°C for 30 sec, and extension at 72°C for 30 sec. Transcript levels of target genes were normalized to β-actin expression levels.
RESULTS
The plates were incubated at 27°C for 4 days to allow cell attachment and stabilization. After 24 h, cells were observed to be evenly attached. By 48 h, the cells appeared larger and exhibited a slightly more spread-out morphology. Finally, at 96 h (day 4), cells displayed the largest size and were most extensively attached to the plate, indicating successful stabilization (Fig. 2). Subsequently, the culture medium was replaced in each well-plate to establish the treatment groups. The control group was replaced with a normal culture medium, while the NaCl group with a culture medium containing 20 mM NaCl, the SPE group with a normal culture medium containing SPE and the NaCl+SPE group with a culture medium containing 20 mM NaCl and SPE, respectively. The cells were then further incubated at 27°C for 6 h. Microscopic examination revealed that cells in all four experimental groups displayed a similar spread-out morphology, size, and quantity (Fig. 3). The plasma osmolality of the experimental eels was 305±7.8 mOsm/kg. Osmolality of the culture medium at the end of experiment is control 348±8.1 mOsm/kg, NaCl 384±9.7 mOsm/kg, SPE 308±7.1 mOsm/kg and NaCl+SPE 354±7.6 mOsm/kg.
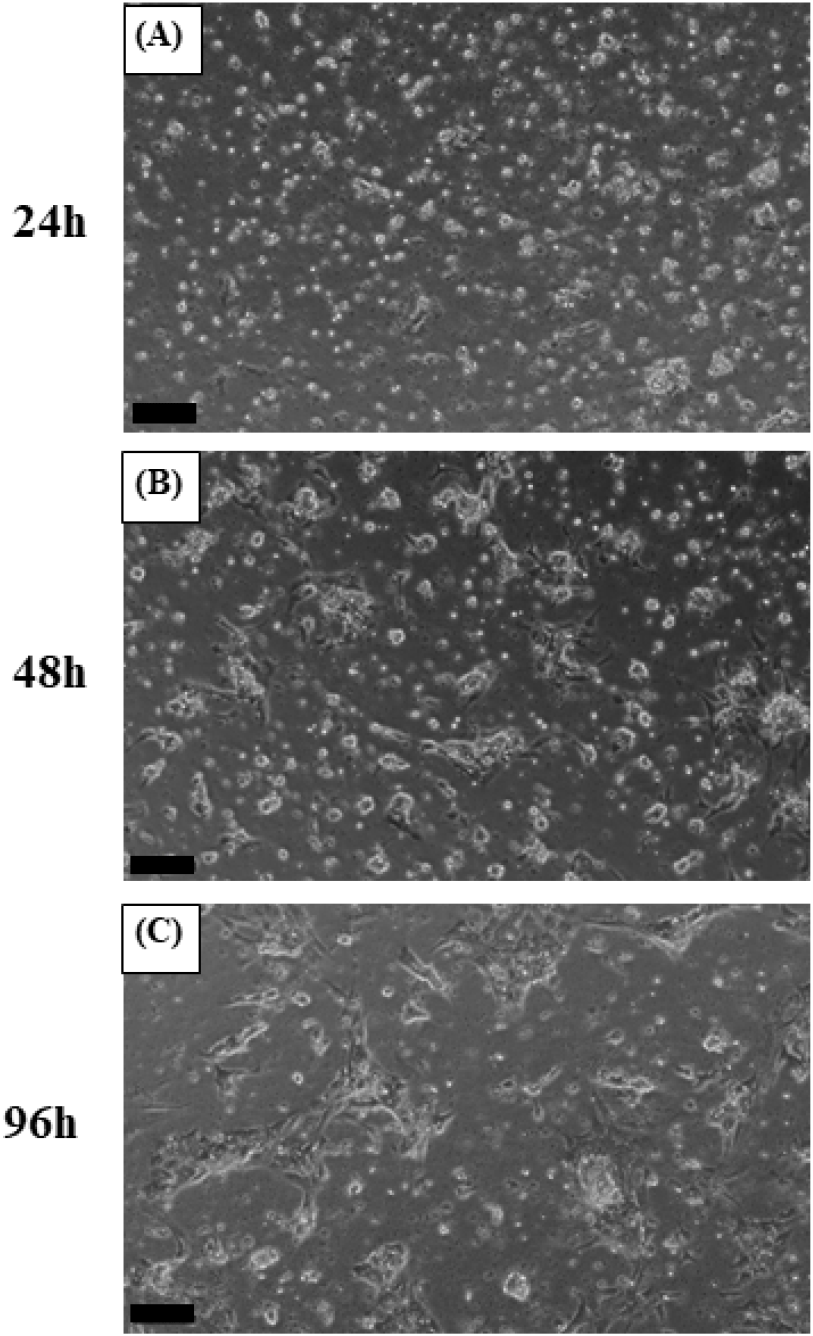
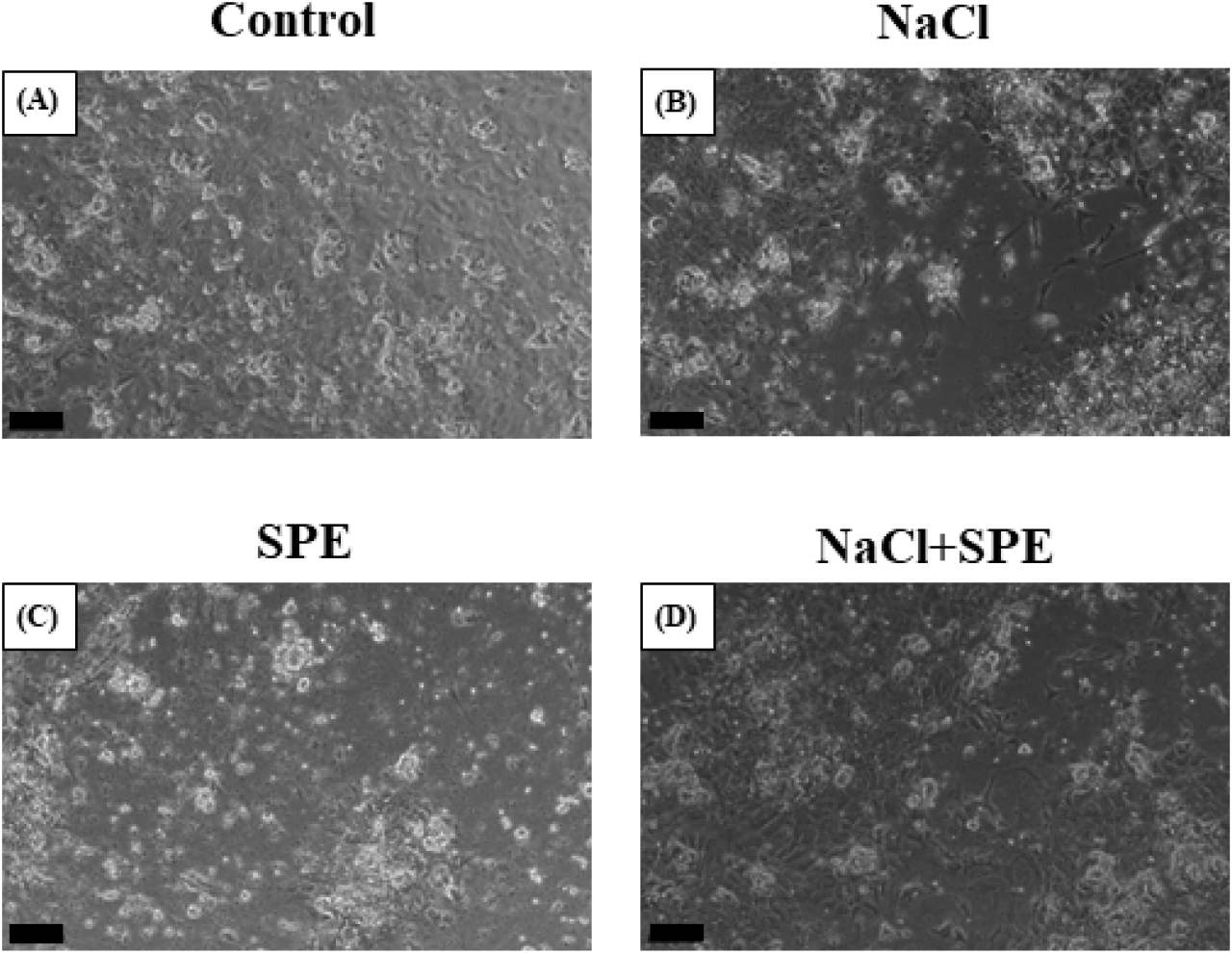
Expression of reproduction and/or salinity-related genes in the pituitary treated with 4 different culture conditions (control, NaCl, SPE, and NaCl+SPE) were examined. There was no significant difference in the expression level of LHβ between control and NaCl groups, but significantly higher in the SPE, and NaCl+SPE groups (p<0.05, Fig. 4A). Expression of FSHβ gene, however, did not significantly differ between treatments although the level in the NaCl+SPE group was higher (Fig. 4B). Pgr1 gene expression was significantly suppressed in all treatments compared to control group (p<0.05, Fig. 4C). Expression level of prolactin gene, a representative salinity-related gene, was significantly lower in the NaCl group compared to the control and SPE groups (p<0.05, Fig. 5A). We further investigated the expression of several reproduction and salinity related genes (drd4, NPBWR2, and rxfp3-2b) (Fig. 5B, C, and D). Interestingly, the control group displayed significantly higher expression levels of drd4 and NPBWR2 compared to the other groups. Expression of rxfp3-2b gene was also significantly higher in the control group compared to the SPE group, but not to the NaCl and NaCl+SPE groups.

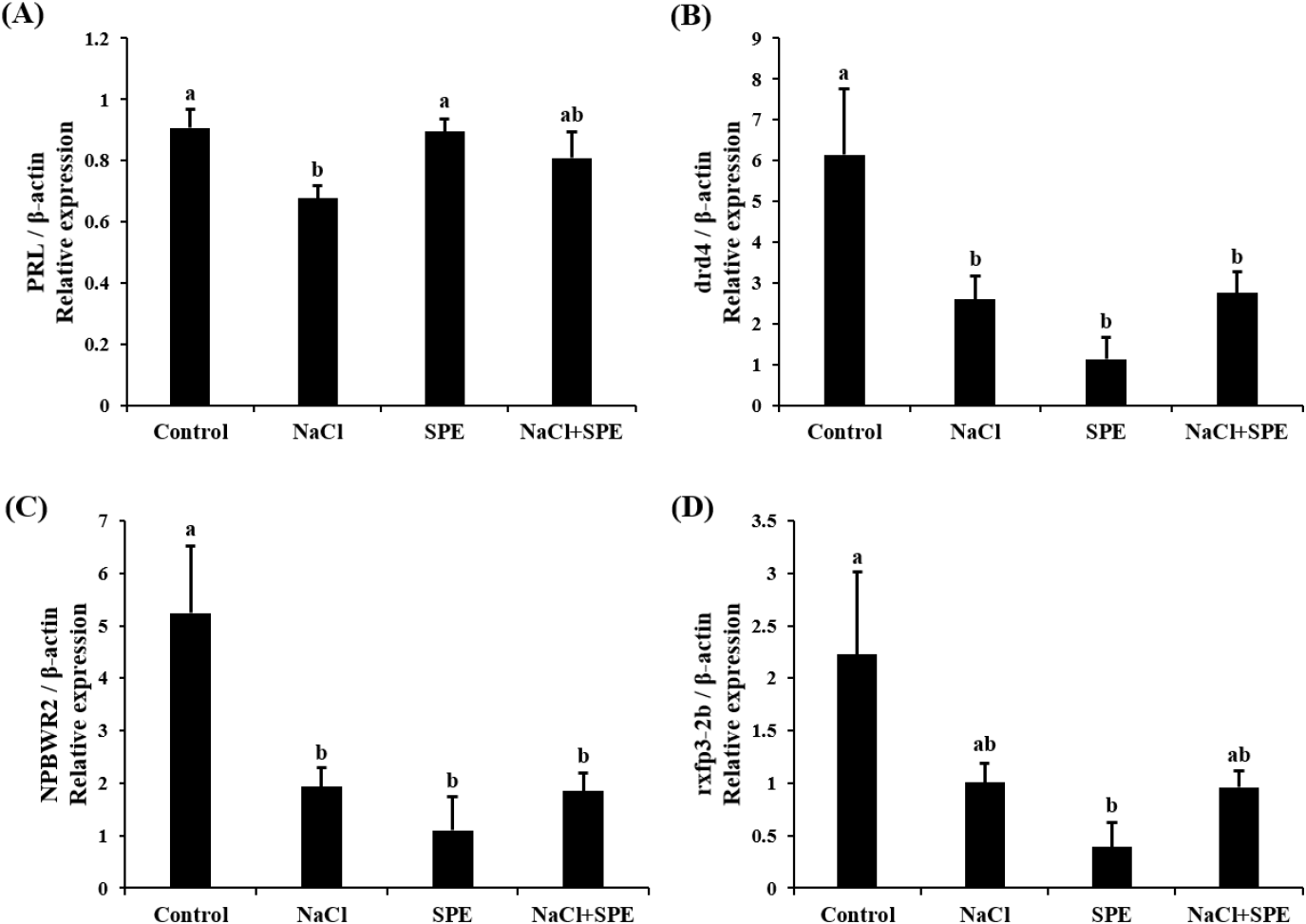
DISCUSSION
In nature, eels are catadromous, migrating from freshwater to seawater for reproduction. Interestingly, seawater rearing seems also necessary in artificially triggering sexual maturity. A study by Kagawa et al. (1998) showed that eels reared in seawater for longer periods required less SPE injections to reach maturity, highlighting seawater’s role in enhancing SPE effectiveness. Furthermore, research suggests seawater can trigger early development of egg yolk precursors (vitellogenesis) (Kagawa et al., 1998). Additionally, Sudo et al. (2011) found significantly higher levels of sex steroids in migrating eels compared to freshwater eels, suggesting hormonal changes triggered by seawater exposure.
NaCl treatment to the primary culture of eel pituitary cell altered osmolality of culture medium and increased or decreased the levels of reproduction and/or salinity-related genes in this study, suggesting the direct action of salinity changes on the pituitary cells. Fish exposed to higher salinity showed increased plasma osmolality even after acclimatization to the salinity (Kammerer et al., 2010), indicating the pituitary in the fish could experience higher osmolality and respond to it. Several studies have investigated the effects of osmolality on gene expression in pituitary cells. For example, Fuentes et al. (2010) examined the influence of varying NaCl concentrations in the culture medium on PRL and growth hormone (GH) gene expression in sea bream (Sparus auratus) pituitary cells. A study of pituitary cell cultures treated with NaCl showed that PRL release from the pituitary of silver sea bream (Sparus sarba) was stimulated by decreased extracellular ion content and osmolality (Kwong et al., 2009).
Freshwater teleost fish typically maintain a blood osmolality range of 250–350 mOsm/kg, while marine teleost fish have a higher range of 350–500 mOsm/kg (Kim et al., 2009). Kim et al. (2008) reported an average plasma osmolality of 311.3±1.5 mOsm/L in the eels (approximately 200 g) acclimated to seawater for 10 days after freshwater rearing. Similarly, Seo et al. (2009) found that eels (approximately 200 g) raised in different salinities for 7 days exhibited the lowest plasma osmolality in freshwater. The plasma osmolality was significantly highest in the seawater group (281–315 mOsm/kg). These studies support that the culture medium used in this study falls within the physiological osmolality range of eels.
The present study proved that the direct action of salinity change on the pituitary. However, salinity change alone seems not strong enough to induce sexual development. Our investigation for GTH gene expression revealed that LHβ mRNA levels were significantly higher in the SPE and NaCl+SPE groups (containing SPE) compared to the control and NaCl groups (without SPE). Increased osmolality by adding NaCl did not strongly affect even with SPE in the case of FSHβ mRNA expression. These results are similar to those of the Japanese eel artificial sexual maturation in vivo experiment and suggest that SPE directly affects pituitary cells, particularly by upregulating LHβ expression (Saito et al., 2003).
Progesterone play crucial roles in development and reproduction in vertebrates, including teleost. Pgrl is known for its role in oocyte maturation (Thomas et al., 2002). However, emerging research suggests its presence and potential function in the fish pituitary gland. Studies have documented pgrl expression in zebrafish pituitary (Hanna & Zhu, 2009) and specifically in LH cells of female Nile tilapia (Hollander-Cohen et al., 2021). Intriguingly, this in vitro study observed changes in pgrl expression, suggesting a potential role for pgrl in sexual maturation and reproduction in eel pituitary cells.
Examining prolactin expression, a key indicator of osmolality changes, revealed the lowest levels in the NaCl group. These findings align with the established role of PRL as a salinity-responsive hormone in teleost, where expression typically decreases with increasing salinity (Breves et al., 2011; Mohammed-Geba et al., 2017). In this study, the culture medium osmolality of the NaCl-treated group was significantly increased compared to the control group, and the expression of PRL in pituitary cells was significantly decreased. Our findings provide further evidence that PRL expression is directly influenced by changes in osmolality within the culture medium. PRL has been implicated in fish reproductive development and attainment of sexual maturity. For example, PRL levels increase alongside gonadotrophins during spawning in the catfish (Clarius batrachus) (Singh & Singh, 1981). Similarly, treatment with estradiol-17β in seabream increased PRL mRNA depending on maturity (Cavaco et al., 2003). These findings suggest a potential role of decreased PRL in eel sexual maturation. However, further investigation is needed to elucidate the precise relationship between PRL and GTH, particularly within the context of artificial sexual maturation in eels, where salinity changes and hormonal manipulations work in concert.
Dopamine is a well-established inhibitor of GnRH and LH action across various vertebrates, including mammals (Thiéry, 1995), amphibians (Sotowska-Brochocka et al., 1994), birds (Contijoch et al., 1992), and teleost (Kah et al., 1987; Vidal et al., 2004; Fontaine et al., 2015). Our study found a decrease in dopamine receptor drd4 expression across all NaCl, SPE, and NaCl+SPE groups compared to the control. This observation aligns with the established principle that dopamine receptor downregulation leads to increased GnRH and GTH expression. Kottmann et al. (2020) proposed a complex role for dopamine in eel sexual maturation, suggesting that continuous SPE injection might override the initial inhibitory dopamine response, ultimately leading to sexual development.
NKBWR is a receptor for NPB and NPW, known to regulate food intake, energy homeostasis and stress in mammals (Mondal et al., 2003; Samson et al., 2004; Levine et al., 2005; Date et al., 2010). In chickens, NKBWR2 is highly expressed in the pituitary gland, and NPW can influence the secretion of GH and PRL depending on NKBWR2 levels (Bu et al., 2016). Similarly, NPB has been shown to regulate the secretion of progesterone and estradiol in pigs (Yang et al., 2018). Our in vitro study using NaCl and SPE treatments revealed changes in NKBWR2 expression, suggesting its potential involvement in both osmoregulation and reproductive processes in the eel. This finding warrants further investigation to elucidate the precise role of NKBWR2 in these physiological systems.
Relaxin 3, relaxin family peptide, has shown promise in osmoregulation. Studies in rats demonstrated that intraventricular Relaxin 3 injection stimulated water drinking and LH secretion, suggesting a potential link between nutritional status, reproduction, and osmotic balance (McGowan et al., 2008; Otsubo et al., 2010). In fish, the relaxin family (rxfp1, rxfp2b, rxfp3-2a, and rxfp3-2b) appears to be involved in osmoregulation. Three-spined stickleback (Gasterosteus aculeatus) studies revealed differential regulation of relaxin family peptide receptor by salinity signals, suggesting their role in this process (Kusakabe et al., 2014). However, research on the relaxin family in eels has yielded mixed results. While Hu et al. (2011) identified the relaxin family in the eel brain, expression levels did not significantly change with seawater acclimation. This discrepancy may be a difference between in vivo and in vitro. In this study, employing in vitro eel pituitary cell culture, we observed a decrease in rxfp3-2b expression upon treatment with NaCl and SPE. These findings suggest a potential role for rxfp3-2b in osmoregulation or reproduction-related processes in eel pituitary cells.
Altogether, in this study, we have experimentally proved that the direct action of salinity change and SPE on the pituitary of eels. Results from this study also suggest that salinity change is necessary but work together with SPE to induce reproductive process, and that LHβ, pgr1, PRL, drd4, NPBWR2, and rxfp3-2b genes are obviously associated with reproduction and salinity changes in eels. Further studies are required to understand the possible concurrent operation of osmoregulatory and sexual maturation pathways in this species.