INTRODUCTION
Spermatogenesis is the dynamic process by which spermatozoa (sperm) are produced from spermatogonium cells in the seminiferous tubules of the testis. This process starts with the mitotic division of the spermatogonial stem cells located close to the basement membrane of the seminiferous tubules (de Kretser et al., 1998). The spermatogonial stem cells produce two types of cells, type A and type B cells. The former replenishes the stem cells and the latter differentiates into primary spermatocytes. The primary spermatocyte divides twice meiotically to generate four spermatids. The spermatids are transformed into free sperm by the differentiation of spermiogenesis.
During these courses of germ cells generation, the notably primary spermatocytes must pass through between the Sertoli cells in the epithelial layer of the seminiferous tubules (Mruk & Cheng, 2015; Hollenbach et al., 2018). The Sertoli cells are associated with each other by cell junctions, named the blood–testis barrier (BTB) including tight junctions, basal ectoplasmic specialization (ES), and gap junction. The connections imply Sertoli-Sertoli and Sertoli-germ cell interactions at the cell junctions. The BTB prevents water, electrolytes, ions, and biomolecules from traversing paracellular space and provides an immune privilege to the meiotic and post-meiotic germ cell. And the BTB separates the germinative epithelial layer into the basal and the adluminal compartment. The germ cells are immotile cells, and they move passively according to the passage situations, undergoing repeatedly locking and unlocking of the structures on the surface of Sertoli cells to enable for the germ cells to move. Post-meiotic germ cell development is taken place in the adluminal part and results in production of free and independent sperm.
On the other hand, many small mammals inhabiting in temperate zone present a characteristic of seasonal breeding. They show a cyclic alteration of active and inactive spermatogenesis (Choi & Lee 2012). Their spermatogenesis is arrested in the winter season due to the shortened length of day time called short photoperiod (SP). And animals are unable to breed for several months (Young et al., 1999; Young et al., 2001; Luaces et al., 2013, 2014). The seminiferous tubules of the regressed testis contain only Sertoli and spermatogonial cells, displaying complete absence of meiosis process. Contrarily in others of the seasonal breeders, spermatogenesis is partially sustained in the inactive testes, and some meiotic activity is remained (Andersen Berg et al., 2001; Morales et al., 2002; Morales et al., 2007; Dadhich et al., 2010, 2013). The seminiferous tubules of the regressed testis contain Sertoli, spermatogonial cells, and a few spermatocytes, implying a subsistence of meiosis.
In the active testes, the BTB is tightly regulated and involved in the rapid reorganization of junction-associated molecules. In the inactive testes, the BTB has been shown to become permeable, leading the molecules to reach the adluminal compartment of the seminiferous epithelium (Jiménez et al., 2015). This alteration of functionality is related to the rearrangement of cell adhesion proteins composed of the tight junctions. The findings triggered to investigate the expressions of genes forming cell junction in reproductively active and inactive testes of Syrian hamsters.
Three molecules consisting of BTB were attracted for this investigation. Claudin-11 was selected among various claudins because its knockout mice resulted in sterile due to loss of tight junction integrity in mice (Morita et al., 1999; Mazaud Guittot et al., 2010; Pan et al., 2018). Moreover, other members of claudin were reported to be involved in the reorganization of the tight junction (Meng et al., 2005; Morrow et al., 2009; Smith & Braun, 2012). And in occludin knockout mice, the testes became sterile at late period of life time despite of normal histological view at six weeks of age (Saitou et al., 2000). Junctional adhesion molecules (JAMs) have been presented at the Sertoli cell tight junctions, indicating the involvement in Sertoli cell-germ cell communication (Gliki et al., 2004).
Therefore, the goal of the present work was to show the expressions of the genes related to cell junctions of BTB in testes of the Syrian hamsters whose reproductive activities were active or inactive. The full length of epididymides were traced whether there were some germ cells including free sperm.
MATERIALS AND METHODS
Mature male Syrian hamsters (Mesocricetus auratus) as a photoperiodic breeding mammal were used in this investigation. They were housed in animal breeding boxes inside the animal breeding room those were manufactured with plywood and the lighting scheme was regulated by the plug-in timer. Ventilation was furnished with the small fans in one side and the outer lighting was blocked completely by using curved black sheets. The animals were fed with standard laboratory mouse chow and tap water ad libitum. Sanitary conditions were managed repeatedly. The long photoperiod (LP) was set by lights of 14 hours and darkness of 10 hours and the LP lights of 10 hours and darkness of 14 hours. The animal breeding boxes were lined side by side. The lighting of animal breeding room was adjusted to identical to the SP lighting scheme and the middle time in day time of each photoperiod was synchronized and checked daily in the ambient temperature of 22±1°C. The condition of management of animals was approved by the Yongin University Institutional Animal Care and Use Committee (YUIACUC-2020-02).
The animals were arranged into two groups: animals kept in LP or SP lighting condition. They were housed in each photoperiod for 8 full weeks.
The hamsters’ body weights were weighed at two weeks intervals. The testicular volume was measured by laparotomy at 0, 4, and 8 weeks. The animals were anesthetized with Zoletil 50 (Virbac, Carros, France), if necessary, with the aid of diethyl ether. Following the excising the skin overlying the scrotal sac and protruding the testicles within the scrotal sac, the major axis and the minor axis of the testicles were immediately measured by vernier calipers (series 530, Mitutoyo America, Boulevard Aurora, IL, USA). The skin excised was sutured with autoclips (CLAY ADAMS® brand, MikRon Precision, Monroe, CT, USA). At the end of the experiment, after the determination of the testicular mass as mentioned above, the animals were decapitated and the various internal organs, including the reproductive accessory organs, were isolated and directly weighed. The testicles and epididymides were kept in formalin until use for histological examination.
The measured values of the testicles were converted into testicular mass by calculating the major axis and the minor axis via a converting equation formulated previously (Watson-Whitmyre & Stetson, 1985). The calculated masses of testicles were compared, plotted, and analyzed with the testicular weights those were directly weighed.
The expressions of genes related to the structure of BTB were examined in the testes of two reproductively active and inactive Syrian hamsters those were prepared by regulating the photoperiod. The genes were claudin-11, occludin, and JAM.
The primer sequences used in the present investigation were selected from the reports provided by the GenBank and the National Center for Biotechnology Information. Table 1 shows the primers chosen. Glyceraldehydes-3-phosphate dehydrogenase (GAPDH) was used as reference standard for RT-PCRs in this study. Sequences were determined by a commercial sequencing service company (Bioneer, Danjeon, Korea).
RT-PCR, reverse transcription-polymerase chain reaction; bp, base pair; JAM, junction adhesion molecule; GAPDH, glyceralde-hyde 3-phosphate dehydrogenase; F, forward; R, reverse.
Testicular tissues were subjected to the total RNA extractions by using TRIzolⓇ Reagent (Invitrogen, Carlsbad, CA, USA) according to the manufacturer’s protocol. A part of testicular tissue (50-100 mg) was excised and sonicated (VCX130, Vibra CellTM, Sonics & Materials, Newtown, CT, USA) with 1 mL of TRIzolⓇ Reagent. The samples were moved into new microcentrifuge tubes and spun at 12,000 rpm for 5 min at 4°C. The supernatant was transferred into the new tubes and kept at room temperature for 5 min of incubation, permitting entire dissociation of the nucleoprotein complex. The tubes had 0.2 mL of chloroform and were capped securely. After the incubation of 2-3 min, the tubes were spun at 12,000 rpm for 15 min at 4°C. The upper aqueous phase was relocated to the new tubes. 0.5 mL of isopropanol was added and left at room temperature for 10 min. Then the tubes were rotated 12,000 rpm for 10 min at 4°C (Sartorius, Centrisart A-14C, Gottingen, Germany). The supernatant was removed and the pellets were resolved in 1 mL of 75% ethyl alcohol. Following agitation, the samples were rotated at 7,500 rpm for 5 min at 4°C. The supernatant was removed and the pellets were dried for at least 5 min. The pellet was resolved with 20-50 μL of RNase-free water.
The extracted total RNAs were applied to RT-PCR reactions. According to the manufacturer’s instructions the reactions were carried out with Maxime™ RT PreMix and AccuPower PCR Premix (Bioneer). Reverse transcription was initially performed to create complementary cDNAs representing tissue-specific RNA populations. An appropriate amount of tRNA was transferred to new microcentrifuge tubes and blended with the following materials: reverse transcription reaction buffer, dNTPS (dATP, dCTP, dGTP, dTTP), oligo (dT) 20 primer, RNase inhibitor, reverse transcriptase, and DEPC-treated water. The tubes were gently shaken and incubated at 42°C for at least 60 min. The tubes were heated to 85°C for 5 min to inactivate the reverse transcriptase. The products were stored at −20°C.
PCR was executed with the cDNA products diluted with TE buffer (10 mM Tris [pH 8.0] and 0.1 mM EDTA). The microcentrifuge tubes with template cDNA (usually 10 ng) were mixed with 10× PCR Buffer, primers (forward and reverse), dNTP Mix, Taq DNA Polymerase, 25 mM MgCl2, and water. The tubes were shaken gently by mixing and spun shortly to fall down all components to the bottom of the tubes. The 40 cycles of PCR were run with the followings in the order: denaturing at 94°C for 20 seconds, annealing at 55°C for 30 seconds, and extension at 72°C for 1 min. The final synthesis stage was performed at 72°C for 5 min and then cooled down to 4°C.
The products were analyzed by using gel electrophoresis in 1.0% agarose gel (100 V, 60 min) and visualized by ethidium bromide. The bands were recognized using the image analysis system (Chemi Doc XRS, Bio-Rad, Hercules, CA, USA).
The PCR products were identified and purified through the agarose gel electrophoresis according to the manufacturer (AccuPrepⓇ PCR/Gel Purification Kit, Bioneer). The visualized gel bands were sliced off using disinfected blade. The gel pieces were mixed with 3 volumes of FB buffer. The clean tubes with the gel pieces were incubated at 50°C for 10 min with mixing by inverting the tubes at the intervals of 2 min. Same amount of absolute isopropanol was added and mixed immediately by repeating upside down. The mixture was relocated to a binding column equipped with a 2 mL collection tube. The cap was closed and spun at 14,000 rpm for 1 min. The binding column was rebuilt with collection tube after discarding the flow-through fluid. 500 μL of W2 buffer was poured and spun at 14,000 rpm for 1 min. The binding column was rebuilt as mentioned above. Then the stage with W2 buffer was repeated again and spun at 14,000 rpm for 1 min. The binding column tube was transferred to a clean 1.5 mL microcentrifuge tube for elution. 30 μL of EA buffer was added cautiously onto the binding column tube and left for at least 1 min at room temperature. Finally, the new tube was spun at 14,000 rpm for 1 min. The eluant was sent to Bioneer for analyzing the sequence of the genes.
The paraffin tissue section was applied for the histological examinations of testicles and epididymides. The tissues were fixed in formalin for the time being. The fixed tissues were dehydrated using a series of increasing concentrations of ethanol (70%, 80%, 90%, 95%, and 100%) for 1.5 hours with mild shaking and immersed in absolute ethanol overnight. The tissues were submerged in xylene three times for 30 minutes and in paraffin at 56°C three times for 30 minutes. Then they were embedded in paraffin and sliced at the width of 5 μm. The slices were mounted on slide glasses and the slides were subjected to hematoxylin (Sigma-Aldrich, St. Louis, MO, USA) and eosin (Sigma-Aldrich) staining solutions for 5 minutes, respectively. They were left for a while to evaporate in the air and treated with Canada balsam (Duksan Pure Chemicals, Ansan, Korea) for permanent specimen, and observed under microscope (DM500, Leica, Wetzlar, Germany).
RESULTS
The body weights of Syrian hamsters were not altered unexpectedly by photoperiod, harmonizing with normal growth pattern as reported in the similar experiment performed previously (Jeon et al., 2020). The animals housed in SP for 8 weeks became a little sensitive to the touching. But there was no any particular aberrant action in any animals housed in each photoperiod.
The real weights of reproductive organs, including testicles, epididymides, and seminal vesicles, were actually weighed at the end of this experiment (Fig. 1). The testicles of animals housed in LP were massive and big but those in SP light and very diminutive. A significant difference of testicular weights in the animals of LP and SP conditions (p<0.05) was found. Likely the results of testicular weights, the weights of the epididymis and the seminal vesicle in the SP animals were significantly different to those in LP animals (p<0.05). All the animals housed in SP presented apparently miniatured reproductive accessory organs.
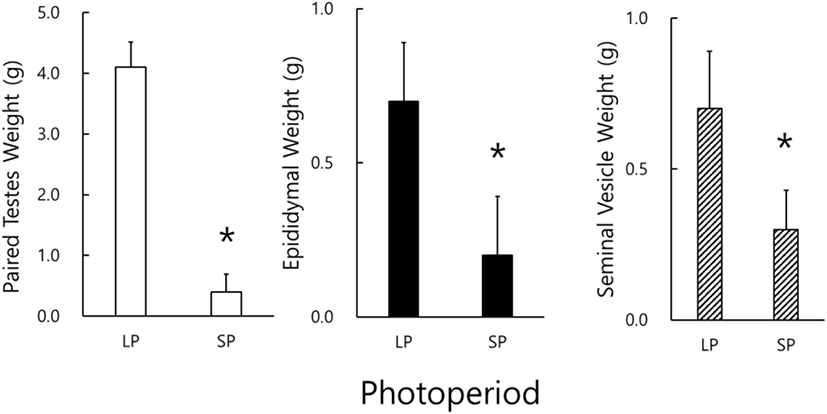
By using the values of the major axis and the minor axis of the testicles, the testicular masses were calculated and were employed on behalf of the actual testicular weights (Watson-Whitmyre & Stetson, 1985). Fig. 2 shows the average changes of testicular mass of hamsters. At the beginning of this experiment all animals had the large and active testes. they also showed relatively large testicles at the 4th week, although the testes became a little diminutive, still sustaining the functional spermatogenesis. At the 8th week the average masses of testicles of SP animals became quite small and were significantly different from those of LP animals (p<0.05).
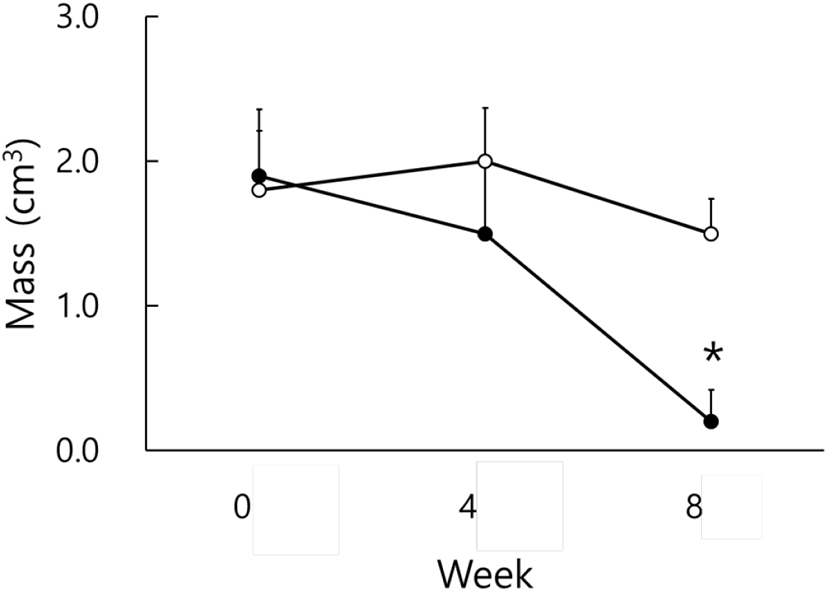
Fig. 3 indicates the testicular masses of individual animals in each group. There were apparent individual differences in groups at the end of the present investigation. Each animal in LP group had large testes all the time (Fig. 3, LP) and that in SP group displayed very small testicles (Fig. 3, SP), demonstrating reproductive active and inactive testes, respectively. If the presence and absence of spermatozoa in the testicles is regarded by histological examination as a standard to distinguish gonadal involution, SP animals present entire involution of testis.
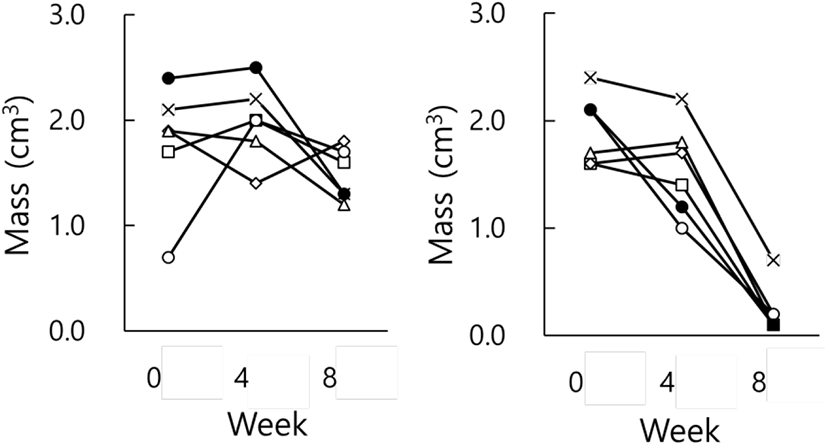
The testicular masses determined by computing from the axes and the actual testicular weights weighed by direct weighing at the end of experiment were compared to scrutinize the mutual correlation between mass and weight. As the correlation coefficient resulted in near to 1 (R2=0.91), it could be evaluated that the values converted from the measures exhibited the values weighed literally as reported previously (Lee et al., 2013; Jeon et al., 2020). The findings imply that the values calculated could completely replace substantially the values weighed directly.
Various organs were isolated and weighed to inspect any weighable alterations of internal organs at the end of experiment (Table 2). The weights of the other organs that were unrelated to the reproductive functions were not discernibly modified by the photoperiod.
Variable | LP | SP |
---|---|---|
Heart (g) | 0.7±0.16 | 0.8±0.14 |
Lung (g) | 0.9±0.11 | 0.8±0.09 |
Liver (g) | 4.9±0.68 | 4.4±0.75 |
Spleen (g) | 0.2±0.04 | 0.2±0.05 |
Kidney (g) | 1.4±0.2 | 1.2±0.13 |
Data are represented as the mean±SD (n=6).
LP, long photoperiod; SP, short photoperiod.
The male Syrian hamsters housed in LP for 8 weeks resulted in the large testes that was nearly 2.05 g of average individually, which represents full spermatogenic activities. The expressions of BTB genes were primarily detected by RT-PCR in the Syrian hamsters housed in LP (Fig. 4). The claudin, occludin, and JAM genes were obviously expressed in the animals. The animals housed in SP for 8 weeks showed very diminutive testes that was 0.20 g of average of each testis, which represents a complete arrest of spermatogenesis. In the sexually regressed and small testes, claudin was weakly expressed. On the other hand, occludin and JAM genes were not observed at all. GAPDH was used as reference standard for RT-PCRs.
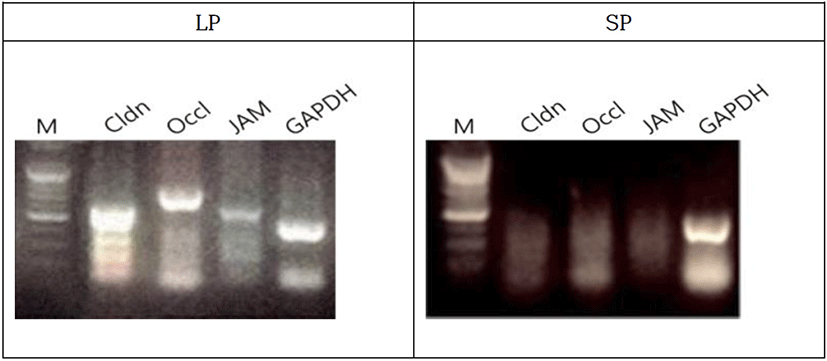
The testicles were categorized into reproductively active and inactive ones (Fig. 5). The active testicles and the inactive ones were shown in all animals of each photoperiod of LP and SP, respectively.
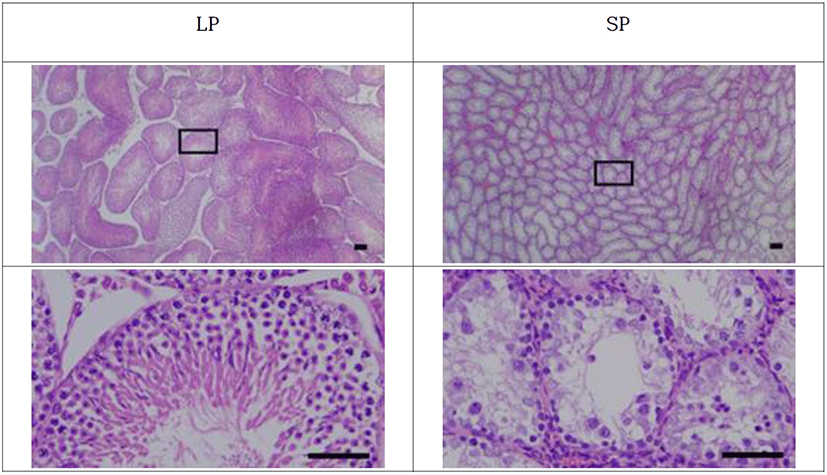
The active testicles showed all stages of germ cells, including spermatogonia, spermatocytes, spermatids, and spermatozoa. These results were apparent in the spacious diameter of the seminiferous tubules. The average diameter of the tubules of active testicles was near 280 μm. The lumen of the seminiferous tubules was full of spermatozoa with tails similar to a sort of wave-like pattern. Contrarily the inactive testicles displayed little germ cells in the epithelium of the seminiferous tubules. SP animals showed primarily spermatogonia and some spermatocytes, without any spermatids and sperm with tail, in the seminiferous tubules. The diameter of the tubules in SP animals was roughly less than half, meaning one eighth in volume compared to the LP animals.
The histological views of the epididymis were generally associated to those of testes examined above (Fig. 6). The entire length of epididymides (caput, corpus, and caudal parts) of LP animals was filled with the sperm. But in the lumen of the epididymal tubules of SP animals, sperm were only observed in the caudal part of the epididymis. No sperm were witnessed in the caput and corpus parts of the epididymis. This finding was not expected.
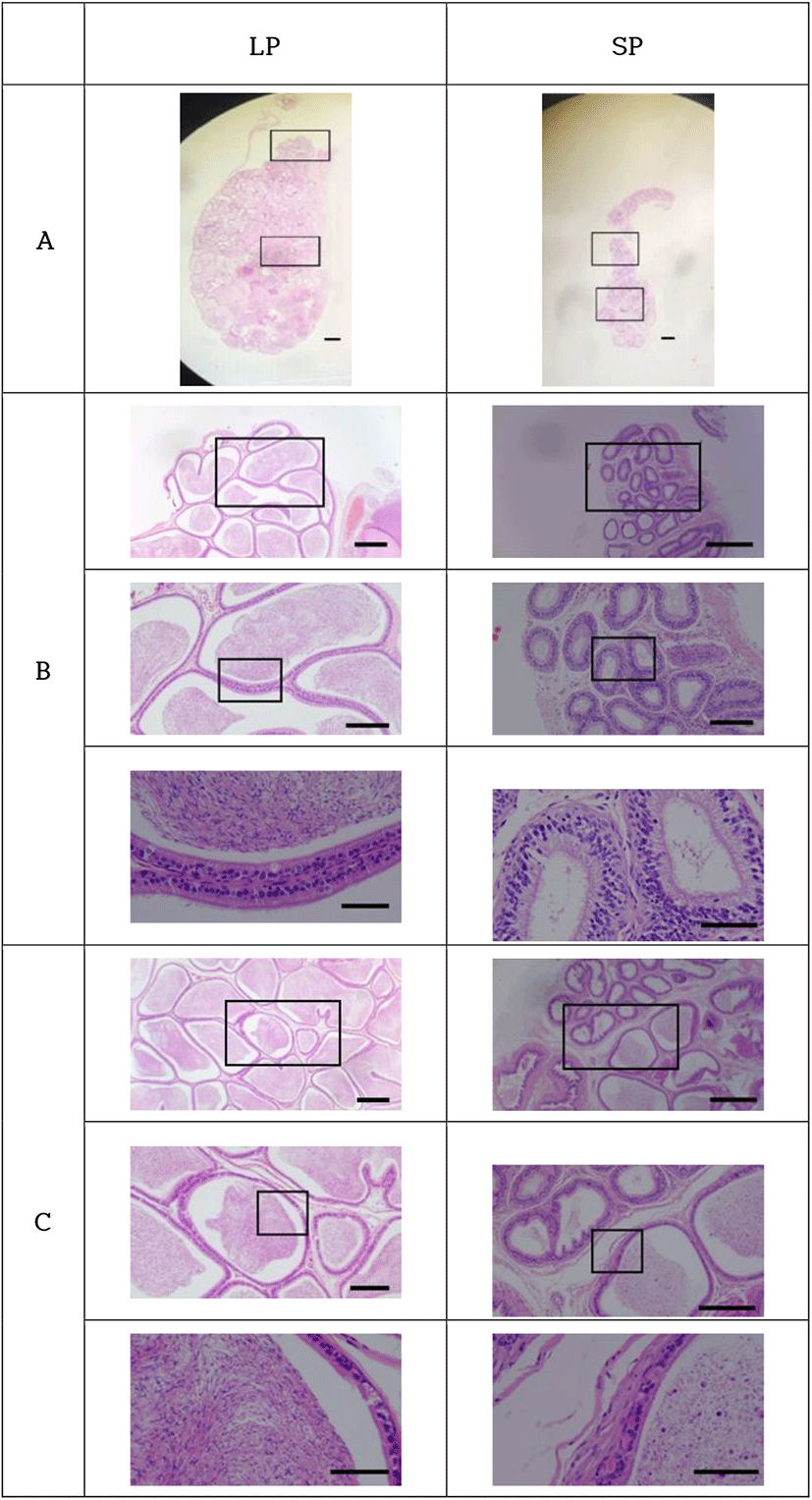
The sperm observed in the epididymal tubules of animals housed in SP had the typical hooked head shape of the normal sperm. Other round cells were observed as well, conjecturing as the germ cells and meaning cells detached from the epithelial tissue of both the seminiferous tubules of testicles and the epididymides. Those outcomes might denote the desquamation process of the germ cells in the process of regression by exposure to SP.
DISCUSSION
The exposure of Syrian hamsters to SP caused the testes to regress, as established by many previous investigations (Gaston & Menaker, 1967; Elliott, 1976; Reiter, 1980; Stetson & Watson-Whitmyre, 1984, 1986; Choi, 2013). The results of this investigation present the expressional aspects of BTB genes to be altered in the inactive testes. Also, many sperm were observed in the caudal region of the epididymis of hamster with the degenerated testes. To our knowledge, this is the first report that many sperm still were remained in the last regions of epididymis, meaning that all the germ cells are not engulfed through phagocytosis by Sertoli cells. Also, the outcome denotes that the sperm sloughed off the seminiferous tubules travel epididymis during the regressing course of the testes.
As well-known, SP induced complete regression of reproduction-related sex organs, affected the behavior to be a little sensitive to the touching, and had no influence on the weights of other various internal organs. The involution of the small testes was identified by the reduced weights of testes, epididymis, and seminal vesicles. And histological views showed no sperm in all the seminiferous tubules of the small testes. During the course of exposure to SP, the animals showed no significant difference of testicular masses in the 4 weeks of SP in comparison to the LP animals. The significant differences of testicular masses and weights were appeared in between LP and SP animals in the 8 weeks.
BTB is a well-organized cell membrane structure between Sertoli cells to regulate the transition of the germ cells. Three molecules related to components of BTB were examined in this experiment: claudin-11, occludin, and JAM. All of them are component proteins of the tight junctions those establish the paracellular barrier that controls the movement of germ cells in the intercellular space of Sertoli cells (Mruk & Cheng, 2015; Merico et al., 2019).
In the present investigation, claudin-11 and occludin were strongly expressed in the reproductive active testes. JAM was weakly expressed. Thus, these molecules are considered to be associated with the movement of germ cells. On the other hand, in the inactive testes both claudin-11 and occludin were not detected at all and JAM was expressed barely detectably. The absence of the gene expressions observed in the regressed testes was accompanied by the absence of the maturing germ cells as well as sperm and indicated a sort of modification of tight junction, which are previously reported (Seco-Rovira et al., 2015).
During the normal spermatogenesis, the initial preleptotene/leptotene cells pass through the space of adjacent Sertoli cells where the molecules composing of tight junction are reorganized by opening and closing (Cheng & Mruk, 2012; Wen et al., 2018). Afterward they reach the adluminal compartment of the epithelium of seminiferous tubules, become spermatids, and transforms into the sperm by spermiogenesis those were abundant in the lumen. SP animals showed primarily spermatogonia at the basal laminar and some germ cells in the tubules, proposing still remaining of meiotic process. No spermatids and mature sperm were observed at all in the epithelium and the lumen of the tubules in the inactive testes. The general aspects observed in the inactive testes were associated with the lack of expressions of tight junction genes used in the present experiment, which implies the permeation of BTB (Munoz et al., 1997; Jiménez et al., 2015; Massoud et al., 2018). Although the cause and effect are not clear, it could be speculated that the disorganization of tight junction in the BTB structure loses and releases the germ cells. On the other hand, apoptosis was reported as a method to swallow the aberrant germ cells (Young et al., 1999, 2001; Morales et al., 2002, 2007; Smith et al., 2014; Seco-Rovira et al., 2015; Silva & Barton, 2016). In the testes of Syrian hamsters undergoing testicular regression, the phagocytosis of the Sertoli cell might not be enough to scavenge the deviant cells. This notion is supported by the presence of sperm and round cells remained in the caudal epididymis.
The caudal region of epididymis in the reproductively inactive animals contained sperm but caput and corpus regions near to the testes had no sperm and any other types of cells at all. These unexpected results direct to the desquamation that is a possible procedure occurring in the epithelial layer of the seminiferous tubules in the regressing testes. Thus, it is reasonable to consider that in the regressing testes the germ cells developing in the epithelium begin to liberate from the Sertoli cells and flow to the lumen. During the initial phase some germ cells are engulfed into the Sertoli cells and the remained cells continue to travel the downstream of the reproductive tract. It is not possible from this investigation to determine the time when the germ cells start to release from the epithelium. The true effects of SP are speculated as the time that the structure of BTB begin to disorganize and then germ cells are detached from the epithelium of the seminiferous tubules. Once the germ cells enter the lumen, they should follow the downstream with apoptosis, if any, and recedes into the epididymis and vas deferens. The sperm witnessed in the last part of the epididymis could be the last batch of freed germ cells from the epithelium. The caudal region has a widened diameter and is a well-known space storing sperm (Zhou et al., 2018). The sperm might experience a bottleneck situation and delay the drainage receding into the vas deferens. They ultimately leave the epididymis for the vas deferens and out of body.
In conclusion, the present results imply that the disorganization of tight junction consisting of the BTB accompanies the testicular regression. The developing germ cells in the epithelium of seminiferous tubules are released from the Sertoli cells in the regressing testes. They are swallowed into the Sertoli cells by the phagocytosis or travel into the lumen, supported by the remained sperm in the last region of the epididymis. Accordingly, these findings suggest that apoptosis and desquamation are the processes that eliminate the germ cells in the course of regression in the Syrian hamsters. Further investigations are required to find which molecules are mainly involved and when the situations are occurred in the process of testicular regression.