INTRODUCTION
The stone flounder, K. bicoloratus, is a coast-settled species that spawns during the winter season and is distributed along the coasts of Korea, China and Japan (Kim et al., 2005). In Korea, this species is mainly found in coastal waters in western Korea. It is an edible fish and a commercially important resource for fisheries. In particular, due to the recent sharp reduction in standing stock, this fish has been noted as a possible organism for commercial aquaculture. To maintain the reproduction and propagation of natural living resources, it is important to understand the reproductive biology associated with oocyte differentiation and yolk formation (vitellogenesis) during oogenesis. However, comprehensive ultrastructural studies of teleost fish oogenesis by observations from electron microscopy in Korea have been reported for a relatively small number of species, mainly those of economic importance, including Agrammus agrammus (Chung & Lee, 1985), Sebastes schlegeli (Chung & Chang, 1995), Hexagrammos otakii (Kang et al., 2004) and Boleophthalmus pectinirostris (Chung et al., 2009). To date, there have been several studies on reproduction in K. bicoloratus, including reproductive ecology (Jun 2003; Jun et al., 2006), egg and larval development (Kim, 1982; Kim & Han, 1989; Han & Kim, 1997a; Jun et al., 2002) and growth and maturity (Mori et al., 1986; Chen et al., 1992; Uehara & Shimizu, 1999), and egg and larval development (Minami, 1984; Jun et al., 1999a). In addition, there are studies on this species regarding several aspects of ecology, including development of larvae and juveniles (Han & Kim, 1997b), feeding (Choi, 2000), and on aquaculture, including the technical production of seedling development (Jun et al., 1999b, 2000, 2001), Even though the reproductive ecology of this species has been investigated, its mechanisms of reproduction remain to be fully elucidated. There is limited information available on the oocyte differentiation and the process of vitellogenesis during oogenesis of this species. Recently, it has been shown that the mechanism of vitellogenesis in some fishes occurs through the processes of endogenous autosynthesis and exogenous heterosynthesis (Chung & Chang, 1995). The formation, accumulation and secretions of several special endocrinological substances for gametogenesis show cyclic changes by the regulation of GTH from the pituitary gland according to the control of external or internal environmental factors (Ng et al., 1986). However, the mechanism of vitellogenesis during reproduction in this fish remains unclear. Therefore, it is necessary to study vitellogenesis and the functions of the cellular organelles in the oocytes during oogenesis in the winter-spawning fish. Therefore, the present study used cytological methods to examine oocyte differentiation and the processes of vitellogenesis during oogenesis in K. bicoloratus. The results provide information regarding the mechanism of vitellogenesis in the ovary by electron microscopic observations.
MATERIALS AND METHODS
The stone flounder, K. bicoloratus were collected monthly by the trawl net at the coastal waters between Gyeongryelbiyeoldo, Taean-Gun, Chungcheongnam-do, Korea from January to December 2008 (Fig. 1). After the live fishes were transported to the laboratory, their total lengths and standard length were measured using a ruler.
A total of 80 females were used for ultrastructural studies of germ cell differentiation and vitellogenesis in the oocytes during oogenesis by electron microscopy. With respect to the production of tissue specimens for transmission electron microscopy, excised samples of gonads were cut into small pieces and immediately fixed in 2.5% paraformaldehyde-glutaraldehyde in 0.1 M phosphate buffer (pH 7.4) for 2 h at 4℃. After prefixation, the specimens were washed several times in the buffer solution and then postfixed in 1% osmium tetroxide solution in 0.2 M phosphate buffer (pH 7.4) for 1 h at 4℃. The specimens were then dehydrated in increasing concentrations of ethanol, cleared in propylene oxide, and embedded in Epon-812. Ultrathin sections of Epon-embedded specimens were cut to a thickness of 80–100 nm with a LKB ultramicrotome. The sections were mounted on collodion-coated copper grids, double stained with uranyl acetate followed by lead citrate, and observed under a JEM 100 CX-2 (80 kv) electron microscope.
RESULTS
Based on the morphological and ultrastructural characteristics of oocytes by electron microscopic observations, the developmental phases of the oocytes during oogenesis can be classified into four phases: (1) the oogonial phase, (2) the previtellogenic phase, (3) the vitellogenic phase, and (4) the mature phase.
In addition, the ultrastructure of the granulose cells and thecal cells, which are supply of exogenous substances, that are attached to the oocytes associated with vitellogenesis were investigated. Ultrastructural characteristics of oocyte development, granulosa cells and thecal cells in each stage during oogenesis are as follows:
The oogonial phase: During this phase, the oogonia occurred on the germinal epithelium of the ovarian lobules. An oogonium has a nucleolus in the nucleus, and several mitochondria and vacuoles appeared in the cytoplasm (Fig. 2A).
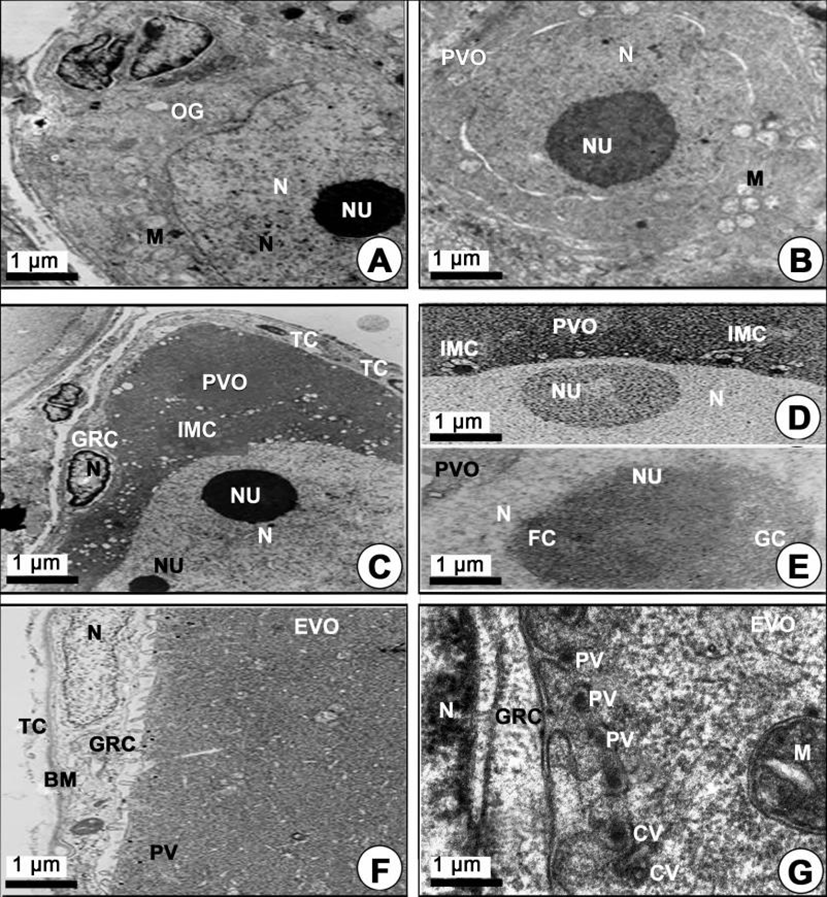
The previtellogenic phase: The oogonial phase developed into the previtellogenic phase by the first prophase of meiosis. The oocytes in the previtellogenic phase can be divided into two forms of oocytes according to the characteristics of oocyte development: 1) the chromatin nucleolus oocyte, and 2) the perinucleolar oocyte.
-
The chromatin nucleolus oocytes (20–100 µm in diameter) contained a large chromatin nucleolus in the nucleus, and a few mitochondria or several intermitochondrial cements appeared around the perinuclear region in the cytoplasm (Fig. 2B). However, at this time the granulosa cells and thecal cell layers on the vitelline envelope of this oocyte were still not observed.
-
The perinucleolar oocytes (approximately 140–170 µm in diameter) contained several perinucleoli along the nuclear envelope, and a few mitochondria and several intermitochondrial cements appeared around the perinuclear region in the cytoplasm. Notably, the granulosa cells were prominently observed on the vitelline envelope of the perinucleolar oocytes (Fig. 2C, 2D). At this phase, the morphological characteristics of nucleoli appeared round or oval in shape. In particular, a nucleolus, which is composed of granular and fibrillar components, appeared in the nucleus (Fig. 2E).
The vitellogenic phase: Development of previtellogenic phase proceeds into the vitellogenic phase. The vitellogenic phase can be divided into two vitellogenic phases: the early and late vitellogenic phases. At the same time, two forms of oocytes occurred: 1) the yolk vesicle oocyte in the early vitellogenic phase, and 2) the yolked oocyte in the late vitellogenic phase.
-
Yolk vesicle oocytes (110–310 µm in diameter): During the early vitellogenic phase, the perinuclear oocytes grew to yolk vesicle oocytes. At this time, granulosa cells with slender nuclei, the basement membrane (BM) and the thecal cells appeared clearly. Notably, the protoplasmic processes began to form on the vitelline envelope. Proteinaceous substances appeared around the cytoplasm of the granulosa cell, and a number of microvilli became present on the vitelline envelope of the early vitellogenic oocyte (Fig. 2F). Then, proteinaceous substances in the pinocytotic vesicles and coated vesicles appeared by pinocytosis in the cytoplasm of the yolk vesicle oocyte near the granulosa cell (Fig. 2F, 2G). In particular, the microvilli of the zona pellucida protruded into the cytoplasmic space, and their morphology was irregular, i.e., from straight to curved, etc. Around the border of the zona pellucida, PVs and coated vesicles appeared (Fig. 2G). In the yolk vesicle oocyte, the occurrence of the Golgi complex appeared in the cytoplasm. The Golgi complex was mainly located adjacent to the yolk vesicle. Especially the Golgi vacuoles and Golgi vesicles appeared near the yolk vesicle so that these seemed to be related to the formation of the yolk vesicle. The Golgi complex, with the Golgi vacuole and the Golgi vesicle, appeared near the yolk vesicles (Fig. 3A). In addition, a few yolk vesicles formed by the Golgi complex in the cytoplasm around the zona pellucida. The yolk vesicles were observed near the Golgi complex in the cytoplasm of in the yolk vesicle oocyte containing the yolk vesicles (approximately 1.1–2.5 µm in diameter). These yolk vesicles were enclosed by the limiting membrane. The inner side of these vesicles is filled with many glycogen particles that show low electron density. As the early vitellogenic oocytes develop, the number and size of the yolk vesicles in the cytoplasm increased. Thereafter, glycogen particles, which were carbohydrate-type yolk precursors, accumulated in the yolk vesicle (Fig. 3B). As development of the yolk vesicle oocytes proceeded and when the oocytes grew to 180–210 µm in diameter, the granulosa cells and thecal cells surrounding the yolk vesicle vitelline envelope of the oocytes became distinct by the BM. An elongated nucleus in the granulosa cell, including a granule and several mitochondria in the cytoplasm, were observed. At this phase, a large blood cell (erythrocyte) appeared in the inner thecal cell (ITC; Fig. 3C). As development of early the vitellogenic oocytes proceeded, a large quantity of carbohydrate-type yolk precursors (YP) converted into lipid droplets near several mitochondria (Fig. 3D).
-
Yolked oocytes (400–500 µm in diameter): During the late vitellogenic phase, the yolk vesicle oocytes grew to become the yolked oocytes. In the yolked oocyte, the vitelline envelopes of the oocytes formed a thicker zona pellucida. At this time, proteinaceous substances in the granulose cell passed into the ooplasm through the micropores of the zona pellucida of the yolked oocyte (Fig. 3E). At this time modification of several mitochondria was observed in the cytoplasm of the oocytes. As a consequence of the modification of the mitochondrial cristae, several multivesicular bodies (MVB) formed. At this time, the sizes of MVB, which were round or oval in shape, were approximately 1.5–3.0 µm in diameter, and each of them has a limiting membrane (Fig. 3F, 4A). Several modified mitochondria combined to form the MVB near the rough endoplasmic reticulum (Fig. 4A). Two morphologically different bodies, which were formed by modified mitochondria, appeared in the yolked oocyte during vitellogenesis: 1) the multivesicular body, and 2) the yolk precursors. The MVB appeared near the primary yolk globules (Fig. 4B). In the yolked oocytes, a capillary vessel in the cell of the ITC layer of the oocytes developed to make a red blood cell (RBC) appear. In the cytoplasm of the oocyte, MVB again combined to form the yolk precursor (Fig. 4C). Because the zona pellucida developed rapidly, the thick zona pellucida can be divided into two parts: 1) the outer and 2) the inner zona pellucida. In the cytoplasm of the late vitellogenic oocyte (LVO), these primary yolk globules mixed with each other to become secondary yolk globules under the inner zona pellucida. The well-developed outer and ITC layers, BM, and a granulosa cell layer appeared on the outer zona pellucida (Fig. 4D).
-
Mature phase: As development of the yolked oocyte proceeded, the oocytes containing secondary yolk globules developed into mature oocytes (containing mature yolk globules) during the maturation phase. In mature oocytes or ripe ova (600–700 µm in diameter), growth of the zona pellucida in oocytes led to a thick zona pellucida composed of 6-layer structures between the outer layer and the inner layer of the zona pellucida. However, the several micropores (pits) on the outer zona pellucida were still found. In the cytoplasm of oocytes, lamellar structures near the primary yolk granules (PYG) appeared to be involved in the formation of the tertiary yolk globules. At this phase, the tertiary yolk globules were observed in the cytoplasm of the oocytes (Fig. 4E). Proteinaceous granular and lipid granular substances were observed in the granulosa cell on the thick zona pellucida. The thecal cell layers (outer and ITCs) on the BM continued to develop. When oocytes reached over 600–700 µm in diameter, it was observed that the accumulation of the yolk substances was completed. The homogeneous membrane of the outer layer of the zona pellucida was closed, and the granulosa cells and thecal cell layers separated from the zona pellucida of mature oocytes (ripe ova) (Fig. 4F). At this time, the fully mature yolk globule of mature oocyte was composed of three components: (1) the main body, (2) the superficial layer, and (3) the limiting membrane. The main body had a high electron density. A small yolk globule had one main body, while a large yolk globule had two or more main bodies showing irregular forms. The main body showed a crystalline form (Fig. 5A). After fully ripe oocytes were completed, highly electron dense protein substance droplets appeared in the cytoplasm of the inner and outer thecal cell layers. The protein droplets may be composed of protein-lysis enzyme that is present prior to the ovulation of mature or ripe ova (Fig. 5B).
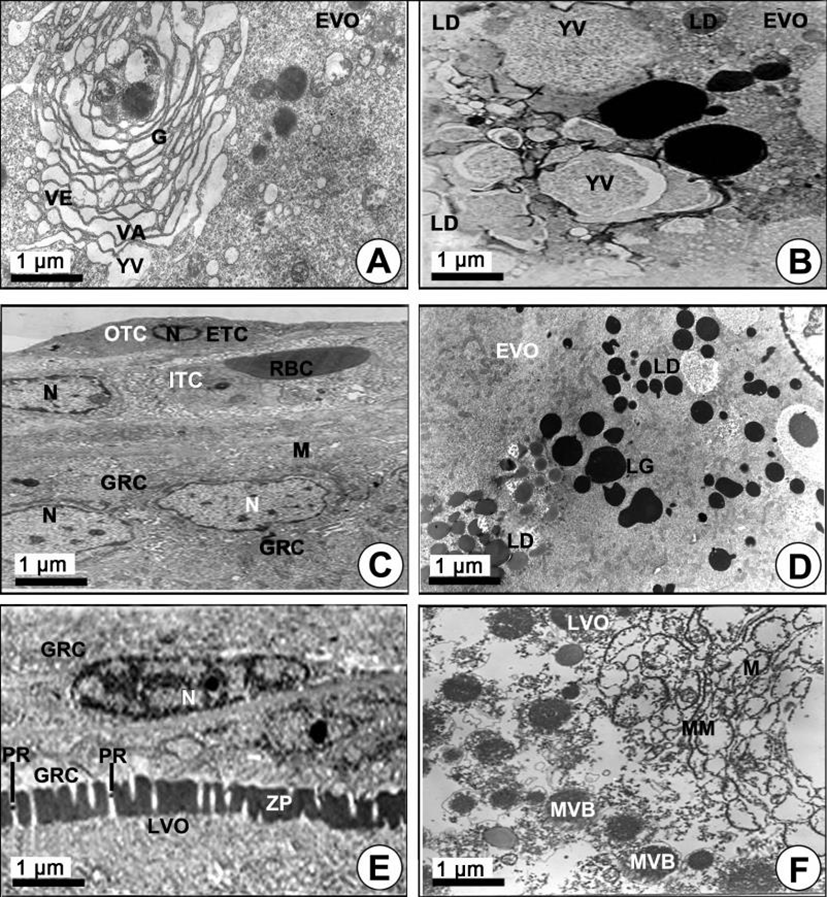
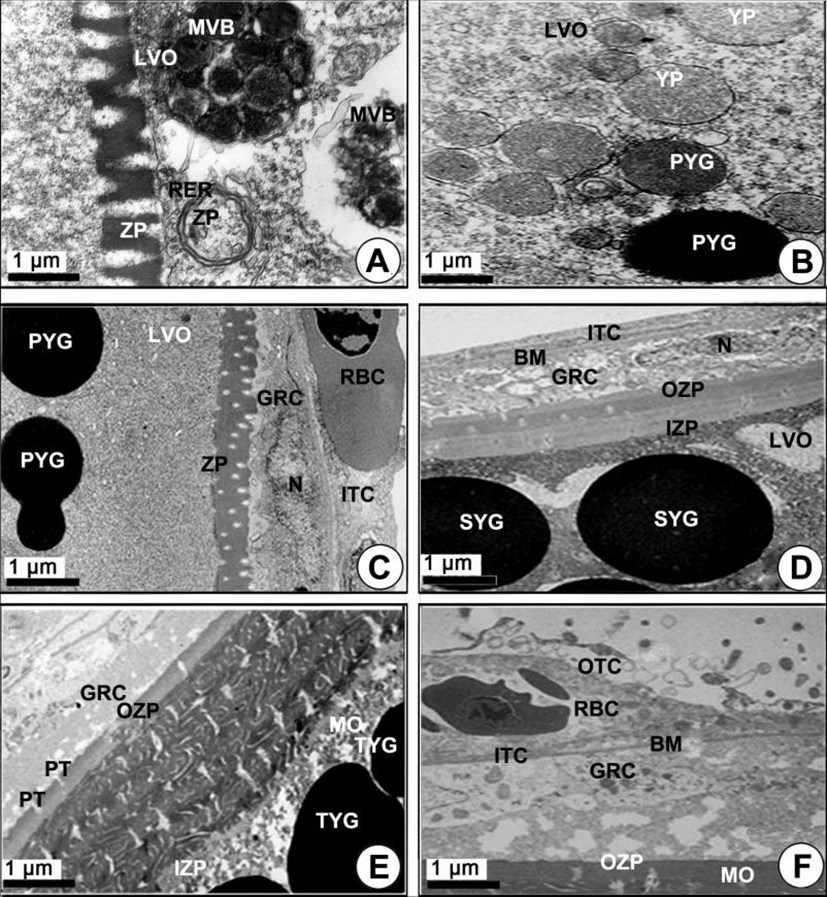
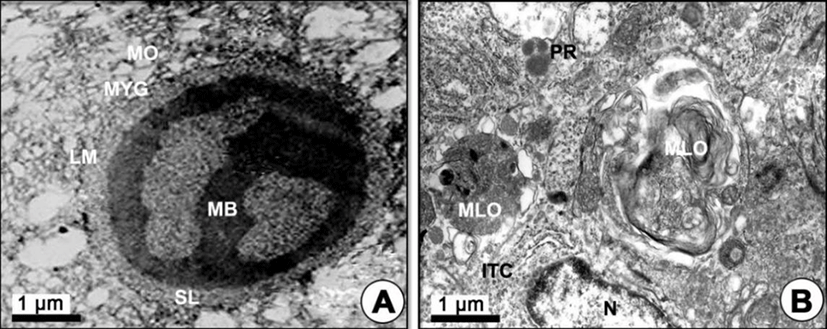
DISCUSSION
In general, the organization of the teleost ovary is variable, and the ovaries can be classified into two basic conditions by the anatomical deposition of the germinal tissue: 1) the gymnovarian condition and 2) the crystovarian condition (Jun, 2003; Kang et al., 2004; Chung et al., 2009). The structures of K. bicoloratus and B. pectinirostris belong to the crystovarian condition as seen in H. otakii (Kang et al., 2004). The ovary of this species is separated into right and left ovaries, and the separated ovaries are asymmetric in shape. The internal structure of the ovary of this species is composed of several ovarian lobules. A nucleolus was composed of two components VIZ: 1) loosely packed fine granules and 2) a fibrous component. Miller (1962, 1966) described that these two parts contain RNA and protein. The synthesis and accumulation of RNA took place in the loose and granular part. Therefore, it is assumed that metabolism might be active at this time.
In the previtellogenic oocyte of K. bicoloratus, intermitochondrial cements appeared in the cytoplasms of the oocytes having a large chromatin-nucleolus in the nucleus around the nuclear envelope. Billard (1984) reported that mitochondrial groupings are associated with intermitochondrial cements. Therefore, after the appearance of intermitochondrial cements, the number of mitochondria in the early developmental stage of oocytes gradually increased. Thus, the intermitochondrial cements are associated with mitochondrial groupings and the multiplication of the number of mitochondria (Chung et al., 2009). In this study, several large vacuoles appeared near the Golgi complex in the cytoplasm of the previtellogenic oocyte. We believe that large vacuoles were formed by the Golgi vacuoles of the Golgi complex. Previous studies have examined the formation of the yolk vesicle in Sebastes schlegeli; (Chung & Chang, 1995) and H. otakii; (Kang et al., 2004). In the present study, several yolk vesicles appeared near large vacuoles or vesicles in the cytoplasm of the early vitellogenic oocyte of K. bicoloratus.
As shown in Fig. 3A, large vacuoles, which were formed by the Golgi complex, gave rise to yolk vesicles. Thus, it is assumed that the Golgi complex plays an important role in the formation of yolk vesicles containing carbohydrate yolks in the early vitellogenic oocyte of K. bicoloratus. In this study, several large vacuoles appeared near the Golgi complex in the cytoplasm of the previtellogenic oocyte. We believe that large vacuoles were formed by the Golgi vacuoles of the Golgi complex. Previous studies have examined the formation of the yolk vesicle in Sebastes schlegeli; (Chung & Chang, 1995), H. otakii; (Kang et al., 2004) and K. bicoloratus (Jun, 2003). In the present study, several yolk vesicles appeared near large vacuoles or vesicles in the cytoplasm of the early vitellogenic oocyte.
Thus, vitellogenesis of K. bicoloratus occurred via endogenous autosynthesis and exogenous heterosynthesis. The process of autosynthesis, involved the combined activity of the Golgi complex, mitochondria and the MVB formed by modified mitochondria. The process of heterosynthesis involved pinocytotic incorporation of extraovarian precursors (such as vitellogenin in the liver) into the zona pellucida (by way of follicle cell layers) of early vitellogenic oocytes.
It is assumed that the Golgi complex plays an important role leading to the formation of yolk vesicles containing carbohydrate yolks in the early vitellogenic oocytes of B. pectinirostris (Chung et al., 2009) and K. bicoloratus (Jun, 2003).
From observations in the present study, it is assumed that the well-developed Golgi complex and the mitochondria in the cytoplasm of the early vitellogenic oocyte are involved in the formation of lipid droplets in the cytoplasm, and they are also involved in the occurrence of the initial zona pellucida on the vitelline envelope through the process of autosynthetic vitellogenesis. At this time, a number of PVs appeared in the cytoplasm near the basal region of the zona pellucida. Then, the PVs were filled with exogenous extraovarian substances, such as vitellogenin substances formed in the liver, from the granulosa cells through heterosynthetic vitellogenesis.
In the present study, two morphological different bodies, which formed by the modified mitochondria, notably appeared in the LVO. One was the MVB, which were intermediate products of the first process, and the other was the yolk precursors, which are the intermediate products of the other process (Gupta & Yamamoto, 1971). The MVB were transformed into the primary yolk globules, while the YP were associated with exogenous primary and secondary PVs near the zona pellucida. After the PVs were taken into yolk precursors, the YP were transformed into the primary yolk globules as seen in zebrafish (Yamamoto & Oota, 1967). In the K. bicoloratus oocyte, the mitochondria were modified at first into the MVB or YP of yolk globules, and then these two bodies became the primary yolk globules and finally formed mature yolk globules via the secondary and tertiary yolk globules. Thus, it is supposed that MVB and YP have a common origin from mitochondria and a common fate of being transformed into the primary yolk globules (Gupta & Yamamoto, 1971).
Regarding the pits (or micropores) of B. pectinirostris, Chung et al. (2009) reported that a number of projections of the follicle cell stretched into the pits of the zona radiata of the yolked oocyte during vitellogenesis, while the pits of the zona radiata of mature oocyte (or ripe ova) were closed in the mature stage. In this study, several well-developed microvilli of the zona pellucida projected into the granulosa cells during vitellogenesis. Although the zona pellucida was very thick, the microvilli of the zona pellucida of mature oocytes disappeared in the maturation phase. It is assumed that various nutrients cannot move into the zona pellucida of mature oocyte because the pits of the zona pellucida were closed. Therefore, our results showed similar phenomena to those of Chung et al., 2009.