INTRODUCTION
Mitochondria are not only one of the major ancient endomembrane systems in eukaryotic cells but they are also the major energy generators in nearly all eukaryotic cells (Coffman & Denegre, 2007; Friedman & Nunnari, 2014). Mitochondrial numbers vary depending on the cell type and can change with the energy needs of the cell. In a liver cell, mitochondria are present about 1,000 to 2,000 (Alberts et al., 2013). Neural and muscle cells may contain many thousands of mitochondria. Mitochondria are seen to form complex branched networks inside the cell with cytoskeleton (Egner et al., 2002; Friedman & Nunnari, 2014). The association with the cytoskeleton, such as microtubule and vimentin, regulates the distribution, function and shape of mitochondria (Hoitzing et al., 2015). Mitochondrial distribution is also correlated with the endoplasmic reticulum (Soltys & Gupta, 1992). It is reported that mitochondria are remarkably mobile organelles. They can move in association with the microtubules from one side to the other of the cells (Lawrence et al., 2016). In animal development, since distributions of mitochondria is closely related to cell differentiation, more mitochondria are transferred to the cells that use a lot of energy compared to other cells (Coffman & Denegre, 2007; Dumollard et al., 2007). Usually, a large number of mitochondria are transferred to the muscle and neural precursor cells.
Ascidian embryos have become an important model for embryological studies, offering a simple example for mechanisms of differential segregation of cytoplasmic components. They have an invariant cleavage pattern with no cell migration or cell death up to the gastrula stage, and the developmental fate of each blastomere are invariant among individual embryos (Nishida, 1987). It is a well-known example that the differential segregation of mitochondria into muscle lineage cells occurs during ascidian embryogenesis (Zalokar & Sardet, 1984; Fujiwara & Satoh, 1990; Nishida, 1990). However, it is still unknown what signaling and molecular events control the polarized distribution of mitochondria. We have been interested in how the asymmetric distribution of mitochondria is achieved during ascidian embryogenesis. In this study, we show three-types of monoclonal antibodies that recognize mitochondria-rich cytoplasm in the ascidian embryo. The staining patterns of these antibodies are very similar to each other. Distribution pattern of mitochondria revealed by these antibodies coincided with that of DiOC2 experiment. These antibodies will be useful immunological probes for studying the polarized distribution of mitochondria in the ascidian embryo.
MATERIALS AND METHODS
During the spawning season, Halocynthia roretzi adults were collected by fishermen in the vicinity of Gangneung-Wonju National University, Gangneung, South Korea. Naturally spawned eggs were inseminated with a dilute suspension of non-self sperm and the fertilized eggs were raised in filtered sea water containing 50 μg/mL streptomycin at 13℃. At this temperature, tadpole larvae hatched about 35 hr after fertilization.
Monoclonal antibodies were produced as described by Fujiwara and Satoh (1990) and Kim and Nishida (1998). Embryos just before hatching were collected and homogenized in an ice-cold physiological saline solution. The homogenate was centrifuged at about 10,000 ×g for 5 min at 4℃ to remove yolk granules. The supernatant was used as an immunogen. Female BALB/c mice were injected intraperitoneally with 0.5 mL supernatant. From the second immunization the mice were injected with the supernatant combined with Freund’s incomplete adjuvant. The immunization was repeated five times. Three days after the last boost, mouse spleen cells were fused with PAI myeloma cells using 50% polyethylene glycol 4,000 Mw in serum-free Dulbecco’s modified Eagle’s medium (DMEM). The fused cells were separated into 96 multiwells and were cultured in DMEM containing hypoxanthine, aminopterin and thymidine. Each clone was assayed by indirect immunofluorescence as described below.
Eggs and various stage embryos of Halocynthia were fixed for 10 minutes in 100% methanol at −20℃, and then in 100% ethanol for 10 min at −20℃. After dechorionation, the whole-mount specimens were washed with phosphate-buffered saline containing 0.05% Triton X-100 (PBSTr) and were stained with the hybridoma supernatant. Indirect immunofluorescence staining was carried out by standard methods using a TSA fluorescein system (PerkinElmer Life Sciences) according to the manufacturer’s protocol. The monoclonal antibodies, Mito-1(5G10, 101), Mito-2 (2G3, 32) and Mito-3 (3B8, 63), specifically recognizes mitochondria-rich cytoplasm in cells of Halocynthia embryos. 3, 3’-Diethyloxacarbocyanine lodide (DiOC2) is a membrane potential fluorescent probe for vital staining of mitochondria and its validity in ascidian embryos has been demonstrated (Zaloker & Sardet, 1984; Nishida, 1990). Dechorionated eggs and embryos were incubated in the filtered sea water that contained 0.5 μg/mL DiOC2 (Thermo Fisher Scientific) for 1 hr at 13℃. The specimens were rinsed several times and then transferred to new filtered sea water. Mitochondria were visualized by green emitted by the DiOC2 stain under a fluorescence microscope.
RESULTS AND DISCUSSION
To obtain specific immunological probes for studying mechanisms involved in the polarized distribution of mitochondria during ascidian embryogenesis, we have produced monoclonal antibodies against mitochondria of the embryos. We immunized mice with a homogenate of whole embryos, fused the mouse spleen cells to mouse myeloma cells to produce hybridoma cell lines. We screened individual hybridoma culture fluid samples by immunofluorescence staining. As described in Materials and Methods, more than 500 specimens were tested, from which we identified and cloned three hybridoma cell lines, 5G10 (Mito-1), 2G3 (Mito-2) and 3B8 (Mito-3), secreting antibodies that recognized mitochondria-rich cytoplasm in the eggs and embryos.
The Mito-1, Mito-2 and Mito-3 antibodies specifically recognized mitochondria-rich cytoplasm from eggs to tailbud embryos. The immunofluorescence results of these antiodies were very similar to each other (Fig. 1 and Fig. 2). At the early tailbud stage, each antibody stained cytoplasm like reticular structure in epidermis cells (Fig. 1B-1D). It seemed to be that the immunostaining signal was localized in the cytoplasm, except for nucleus. Similar staining pattern was observed in a vital staining of mitochondria with DiOC2, a fluorescent probe of mitochondria (Fig. 1A). It was reported that, in eukaryotic cells, mitochondria fuse to form elongated tubular networks around the nucleus (Egner et al., 2002; Friedman & Nunnari, 2014). They are diffusely extended through the cytoplasm. Mitochondrial networks are dynamic, continually breaking apart by fission and fusing again. The features of mitochondria networks in cells were similarly observed in our experiments. Antigens that are recognized by the Mito-1, Mito-2 and Mito-3 antibodies started to be detected from egg cytoplasm. The egg cytoplasm was also stained like networks with these antibodies (Fig. 2A). Mitochondria were dispersed over the cytoplasm, but they were localized more frequently in the cortical cytoplasm of eggs. A polarized distribution of mitochondria in the egg leads to the differential segregation of mitochondria between blastomeres. During cleavage stages, mitochondria are unevenly partitioned into blastomeres (Fig. 2B-2F). At the 8-cell stage, the Mito-1, Mito-2 and Mito-3 antibodies stained the posterior-vegetal B4.1 blastomeres, which mainly give rise to muscle and mesenchyme cells, more strongly than other blastomeres. The immunofluorescence of mitochondria was particularly strong in the posterior myoplasm-rich region of the B4.1 blastomeres (Fig. 2B white arrows). This distribution pattern corresponds to that reported in the previous studies (Zalokar & Sardet, 1984; Fujiwara & Satoh, 1990; Roegiers et al., 1999). These studies showed that the B4.1 blastomeres contain the largest amount of mitochondria among all the blastomeres at the 8-cell stage. Mitochondria are equally distributed in the 2-cell stage embryo of the ascidian Phallusia, but the majority of mitochondria become segregated to the B4.1 blastomeres. In human embryos, differences in the mitochondrial distribution between blastomeres are obvious during the 2-cell and the 8-cell stages (Van Blerkom, 2009). The asymmetric distribution can be traced back to polarized peri-nuclear aggregation at the one-cell stage. Similar asymmetry between blastomeres are also observed in mouse embryo (Acton et al., 2004). Thus, it is suggested that the asymmetric distribution of mitochondria initiated from a one-celled zygote is important for chordate development. It is likely that differential distribution of mitochondria is closely linked to cell fate choice.
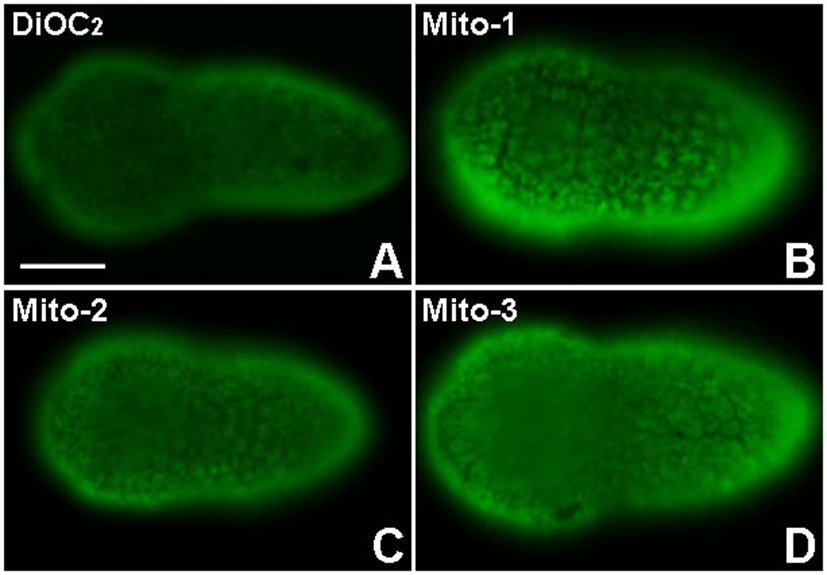
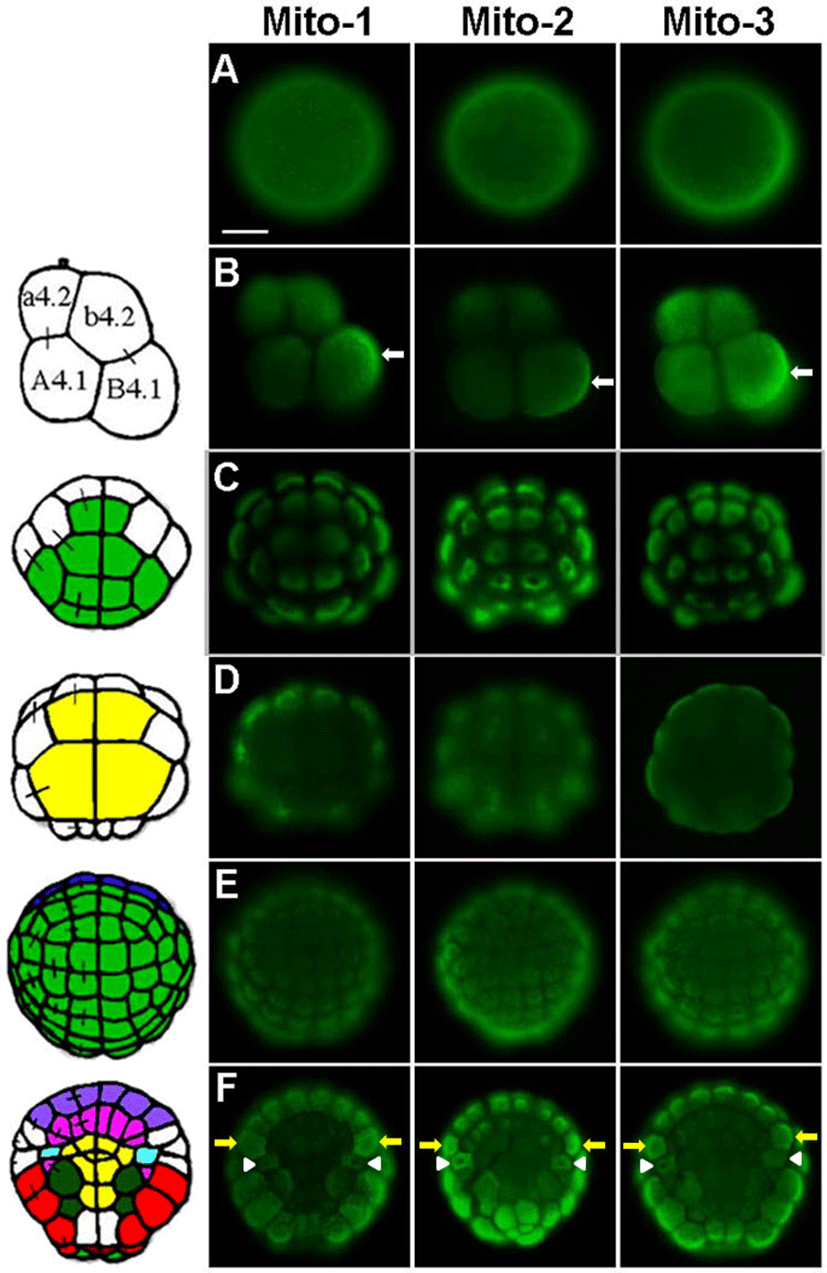
The Mito-1, Mito-2 and Mito-3 antibodies showed that mitochondria are evenly distributed in the animal hemisphere blastomeres, which mainly form epidermis cells, at the 32-cell and the 110-cell stages (Fig. 2C, 2E). Immunostaining signals were localized mainly in the central cytoplasm, except for nuclei. In contrast, mitochondria appeared to be partitioned preferentially into blastomeres of the marginal zone, such as muscle (red) and nerve cord (purple) lineage, in the vegetal hemisphere (Fig. 2D, 2F). It appeared that endoderm (yellow) precursor cells which located in the central zone have been received the smallest amount of mitochondria. Mesenchyme (dark green) and notochord (pink) lineage blastomeres, located between muscle/nerve cord and endoderm, had a relatively small amount of mitochondria. At the 110-cell stage, distribution pattern of mitochondria revealed by these antibodies coincided with that of DiOC2 experiment (Fig. 2F and Fig. 3). DiOC2 fluorescence was detected strongly in the marginal zone blastomeres of the vegetal hemisphere more than in the central zone blastomeres. In addition, it was likely that endoderm blastomeres have the smallest amount of mitochondria. Therefore, it is suggested that these antibodies specifically recognize mitochondria of ascidian embryos, and they will be useful probes for studying mechanisms involved in asymmetric distribution of mitochondria in ascidian embryonic development. DiOC2 is a vital staining dye of mitochondria, whereas immunostaining with antibody requires fixing the specimens. Living specimens are advantageous to investigate successive changes in mitochondria distribution. The same specimen should be observed for a long time to examine the exact distribution of mitochondria. Immunostaining is more suitable for this. Thus, these antibodies and DiOC2 are necessary to study the mechanisms involved in asymmetric distribution of mitochondria during ascidian embryogenesis.
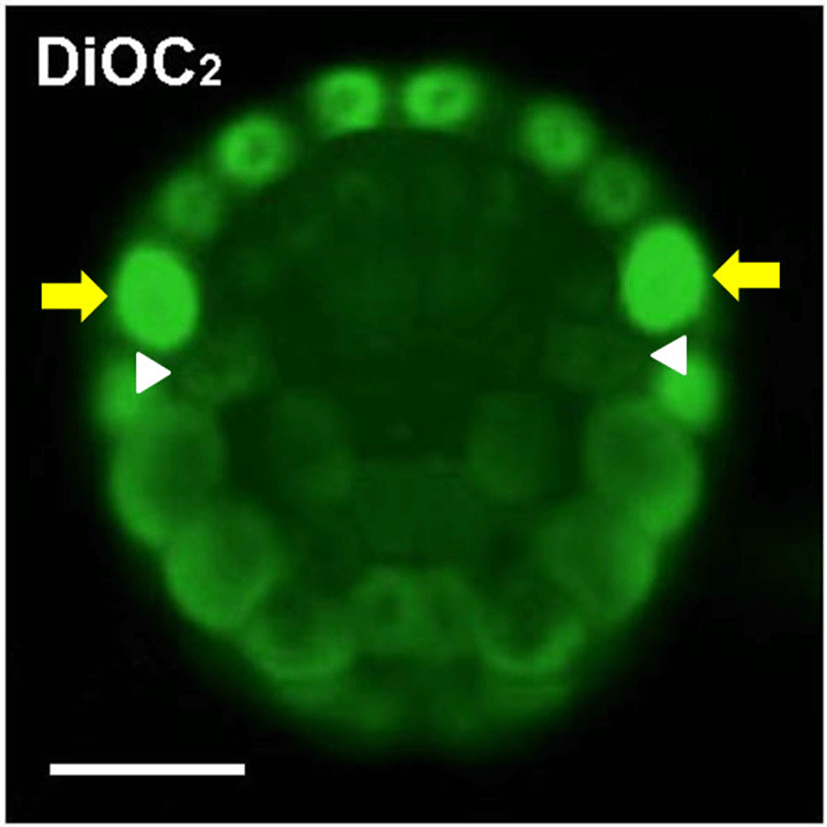
In Fig. 2F and Fig. 3, yellow arrows indicate neural/ secondary muscle-lineage blastomeres and white arrowheads represent trunk lateral cells (TLCs). It is also clear that these cells had a large number of mitochondria. The TLCs are precursors of body-wall muscle and blood cells in adult organisms (Hirano & Nishida, 1997). Considering that mitochondria are preferentially segregated into muscle and neural precursor cells during ascidian embryogenesis, these antibodies specifically recognize mitochondria. Such distribution pattern of mitochondria continued during gastrulation. At the early tailbud stage, muscle cells in tail possess a large amount of mitochondria (data not shown). At the 16-cell and the 32-cell stages, mitochondria which are segregated to one side of the vegetal hemisphere blastomeres transferred into one of the daughter cells (data not shown). In contrast, the polarized distribution of mitochondria did not observe in cytoplasm of the animal hemisphere blastomeres before cell division begins. Zalokar and Sardet (1984) have shown similar observations. However, the molecular events involved in the phenomenon are still unknown. It is probably important to isolate cDNA of the intracellular transport-related genes and to analyze roles of FGF signaling in the polarized distribution of mitochondria. FGF signaling promotes mesenchyme/notochord fates and suppresses muscle/nerve cord fate during ascidian embryogenesis (Kim et al., 2007). Thus, there is a possibility that FGF signaling involved in regulation of the differential segregation of mitochondria.