INTRODUCTION
The epididymis is a part of the male excurrent ductal system and the place in which spermatozoa produced from the testis become mature and acquire fertilizing capacity. The epididymis has a ductal structure and is consisted of a lay of epithelial cells surrounded by smooth muscle layers (Robaire & Hermo, 1988). The epididymal epithelium possesses several cell types, including principal, narrow, basal, halo, and apical cell (Robaire & Hermo, 1988). Each cell type in the epididymal epithelium has different morphologies and functions (Robaire et al., 2006). For example, principal cell, the main epithelial cell type, is characterized by columnar shape, basally localized nucleus, and well-developed secretory and endocytotic vesicles (Robaire et al., 2006). On the other hand, basal cell is localized on the basement membrane of the duct and has extended shape (Robaire et al., 2006). The cytoplasm of basal cell contains typical secretory granules and some endocytotic vesicles (Robaire et al., 2006). Based on these histological features and physiological examinations, it is now well known that the epididymis plays in several important roles for male reproduction, such as transport, maturation, and storage of spermatozoa (Robaire et al., 2000, 2006).
The epididymis is divided into four different regions, including initial segment, caput epididymis, corpus epididymis, and caudal epididymis, based on morphological and functional characteristics (Cosentino & Cockett, 1986; Robaire et al., 2006). The concentration of sodium within the epididymal lumen is gradually decreased from the initial segment to the caudal epididymis, while potassium concentration becomes s increased along the epididymis (Robaire et al., 2006). Luminal concentration of chloride is greatly reduced at the caput epididymis but no clear change at the corpus and caudal epididymis (Robaire et al., 2006). Such changes of luminal composition along the epididymis suggest that unique microenvironment within each compartment of the epididymis is closely related with proper sperm maturation (Robaire et al., 2006). Segmental specification of the epididymis is also found in different distribution of epithelial cell types. For instance, the proposition of the principal cell along the epididymis varies from 65% to 80% of the total cell population of the epididymal epithelium (Trasler et al., 1988). Such compositional difference of cell types among the epididymal regions is considered to in part contribute the creation of segmental-specific microenvironment (Trasler et al., 1988).
The formation and maintenance of distinct microenvironments within different epididymal segments are the result of a complex coordination among cells. A way to accomplish such accordance between neighboring cells is a direct cell-cell communication via gap junction. The gap junction is a specialized intercellular channel which connects the cytoplasm of adjacent cells and allows direct movement of small molecules, ions, and various intracellular signal particles (Goodengough et al., 1996). The gap junction is made of connexin (Cx) molecule, and at least, twenty Cx isoforms have been identified in human and rodents (Willecke et al., 2002). The presence and expression of tissue-specific Cx isoforms have been determined by several researches (Goodengough et al., 1996; Willecke et al., 2002). The differential expression of Cx isoforms in the epididymis during postnatal period has been demonstrated by our previous and other researches (Dufresne et al., 2003; Han & Lee, 2013; Lee 2013; Pointis et al., 2005). Moreover, our recent findings have demonstrated that expression of Cx isoforms in the initial segment and corpus epididymis is differentially regulated by estradiol benzoate, an agonist of estradiol, or flutamide, a synthetic antiandrogen, which is administrated at the early neonatal and/or weaning age (Lee, 2014; Lee & Lee, 2015). However, the effect of estradiol benzoate or flutamide on the expression of Cx isoforms in other epididymal regions has not been determined.
It is well accepted that functions of the epididymis are generally regulated by androgen and in part estrogen (Joseph et al., 2011; Robaire & Hamzeh, 2011). Thus, in the current examination, expressional changes of Cx isoforms in the caudal epididymis of adult rat treated with estradiol benzoate or flutamide at 7 days of postnatal age was investigated by quantitative real-time PCR analysis.
MATERIALS AND METHODS
Male Spragure Dawley rats at 7 days of postnatal age were obtained from pregnant female rats (n=5), which were purchased from Samtako (OSan, Korea) and were individually caged upon the arrival and randomly assigned into 5 different experimental groups, control, low-dose estradiol benzoate (EB) treated (EB-L), high-dose EB treated (EB-H), lowdose flutamide treated (Flu-L), and high-dose flutamide treated (Flu-H) group. Animals were allowed to freely access to food and drinking water for entire experimental period. The present study was carried out in accordance with the guide for the care and use of laboratory animals of National Research Council in S. Korea.
The EB and flutamide were purchased from Tokyo Chemical Industry Co. (Tokyo, Japan). The powder of EB or flutamide was dissolved in 100% EtOH with stirring at room temperature to make the stock solution, and a series of dilution of the stock solution in peanut oil were carried out for preparation of working solution. When male pups at 7 days of age were acquired, the weight of each animal was measured, and a proper amount of working solution of EB or flutamide was subcutaneously injected to make a final concentration of 0.015 mg/kg body weight (BW) for EB-L, 1.5 mg/kg BW for EB-H, 500 mg/kg BW for Flu-L, or 50 mg/Kg BW for Flu-H. Animals in control group were treated with peanut oil instead of EB or flutamide. After the treatment, experimental animals were placed back to their mother for nursery.
Each experimental group was consisted of 6 animals and thus 30 male rats in total were used for the present study. When the animal was reached at 4 months of age, the euthanasia was carried out in CO2 chamber. The male reproductive tract was extracted and placed in cold PBS. Under the dissecting microscope, the epididymis was separated from the rest of male reproductive tract and further divided into different epididymal segments. The caudal epididymis was rinsed in fresh cold PBS and quickly frozen in liquid nitrogen. The tissue was stored in –80°C until used for total RNA extraction.
To isolate total RNA from the caudal epididymis, the tissue was first homogenized in total RNA extraction solution (iNtRON Biotech, Sungnam, Korea). Isopropanol was included into the homogenized solution to precipitate total RNA, and a pellet of total RNA was collected by an ultracentrifugation at 4°C for 30 min. The pellet was rinsed in 70% EtOH once, air-dried, and dissolved in DEPC-treated RNasefree dH2O. Total amount and purity and quality of total RNA extracted were determined by NanoDrop Lite spectrophotometer (Thermo Scientific, Wilmington, DE, USA) and agarose gel electrophoresis, respectively. Total RNAs were stored in –80°C until used as templates for cDNA synthesis.
Construction of cDNA was carried out with 1 mg of total RNA and oligo-dT primer in ImProm-IITM reverse transcription system (Promega, Madision, WI, USA). Then, reverse transcription reactions were performed in a sequence of 25°C for 5 min, 42°C for 90 min, and 70°C for 15 min. For each experimental group, cDNAs were independently generated from total RNAs isolated from four animals. The cDNAs were directly used for quantitative real-time PCR. Table 1 provides information of oligonucleotide primers utilized for real-time PCR analysis.
A reaction cocktail for real-time PCR was prepared with 1 mL of cDNA, 10 pmol of primer set, 10 mL of PCR master mixture (Finnzymes, Espoo, Finland), and dH2O to make a final volume of 20 mL. A pre-denaturation of the reaction mixture was carried out at 95°C for 30 sec, followed by 35 to 40 cycles of denaturation at 95°C for 30 sec, annealing at Tm for 30 sec, and extension at 72°C for 30 sec. At the end of each PCR, an additional extension step at 72°C for 10 min was included. Cyclophilin A (Ppia), the housekeeping gene, was amplified as a PCR internal control. The size of PCR products were evaluated with fractionation in 1.2% agarose gel electrophoresis.
At least three repeats of PCR per cDNA were performed to obtain a mean and a standard error of an experimental group. Data are presented in the relative expressional ratio between Ppia and target Cx isoform. The statistical significance of the difference in mean values among experimental groups of EB or flutamide treatment was assessed by oneway ANOVA, followed by Duncan’s test. The existence of statistical significance between control and experimental group was considered if P value was < 0.05.
RESULTS
Neither EB nor flutamide treatment at 7 days of age didn’t result in significant change of Cx26 expression in the caudal epididymis of adult rat (Fig. 1A). However, the treatment with a low-dose EB caused a significant decrease of Cx30.3 expression, while there was no statistical expressional change of Cx30.3 gene by a high-dose EB treatment (Fig. 1B). The treatment of a high-dose flutamide at 7 days of age led to a significant increase of Cx30.3 expression, even though no significant change of Cx30.3 expression was detected by a low-dose flutamide (Fig. 1B).
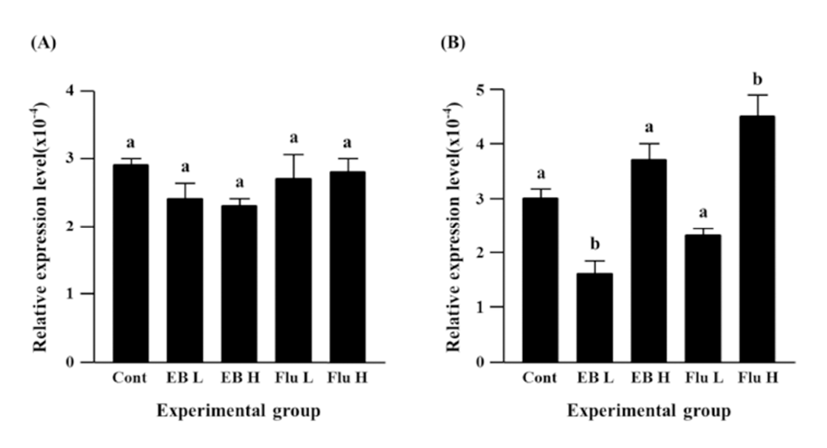
Expression of Cx31 in the caudal epididymis of adult rat was not changed by a low-dose EB treatment at 7 days of age, but a tremendous increase of Cx31 expression was found in the high-dose EB treated group (Fig. 2A). Treatments of both doses of flutamide resulted in significant increases of Cx31 expression, and an increase of Cx31 expression by a high-dose flutamide treatment was significantly higher than that by a low-dose flutamide treatment (Fig. 2A).
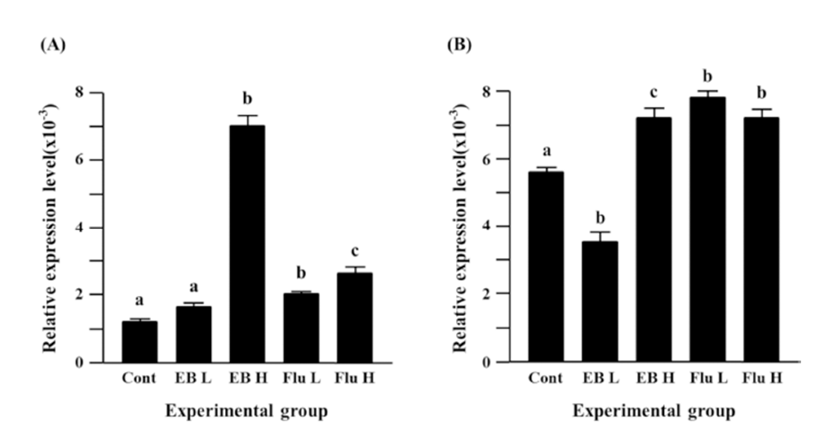
The treatment of a low-dose EB caused a significant decrease of Cx31.1 expression in the adult caudal epididymis, but a significant expressional increase of Cx31.1 gene was observed with the treatment of a high-dose EB (Fig. 2B). Treatments of both flutamide doses resulted in significant increases of Cx31.1 expression, even though there was no difference on the degree of increment of Cx31.1 expression (Fig. 2B).
Fig. 3A shows the expressional change of Cx32 gene in the adult caudal epididymis exposed to EB or flutamide at 7 days of postnatal age. Both EB and flutamide treatments resulted in significant increases of Cx32 gene expression, compared with that of control (Fig. 3A). Treatments with different doses of EB or flutamide didn’t cause significant expressional changes of Cx32 gene (Fig. 3A).
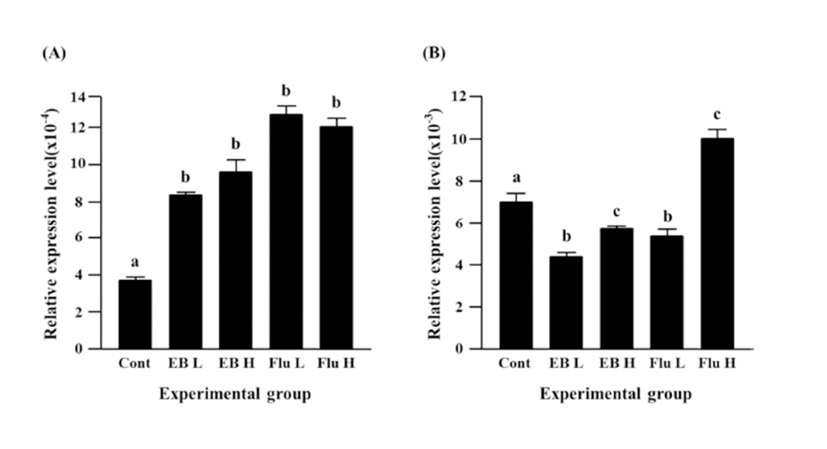
Expression of Cx37 was significantly decreased by EB treatment (Fig. 3B). Reduction of Cx37 gene expression was more significant with a low-dose EB treatment than that with a high-dose EB treatment (Fig. 3B). The treatment of a low-dose flutamide also induced a significant decrease of Cx37 gene expression, but expression of Cx37 gene was significantly increased by a high-dose flutamide (Fig. 3B).
The treatment of a low-dose EB didn’t influence on expression of Cx40 in the caudal epididymis, while a significant increase of Cx40 transcript level was detected with the treatment of a high-dose EB (Fig. 4A). Neither a low-dose nor a high-dose flutamide affected expression of Cx40 gene (Fig. 4A).
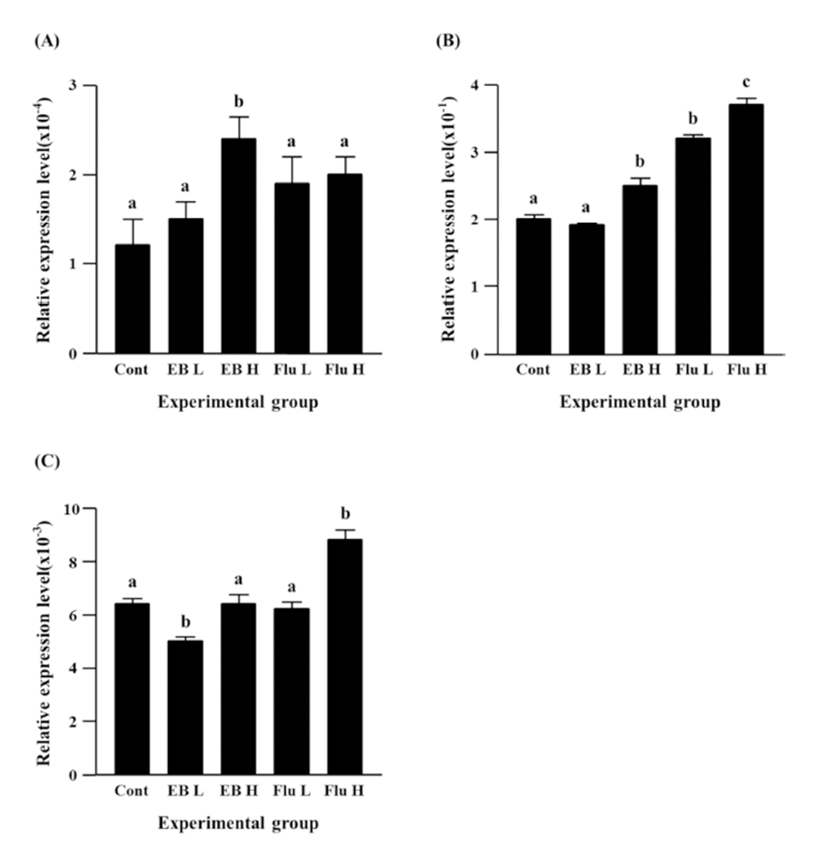
Expression of Cx43 gene was not changed by a lowdose EB treatment, but a significant increase of Cx43 expression was observed with the treatment of a high-dose EB (Fig. 4B). Both treatments of flutamide at different doses resulted in significant increases of Cx43 expression, even though administration of a high-dose flutamide led to a significant increase of Cx43 expression than that of a low-dose flutamide (Fig. 4B).
There was no change of Cx45 gene expression by a high-dose EB treatment, even though a low-dose EB resulted in a significant decrease of Cx45 expression (Fig. 4C). No significant change of Cx45 expression was also detected in a low-dose flutamide-treated group (Fig. 4C). The treatment of a high-dose flutamide caused a significant increase of Cx45 expression in the adult caudal epididymis (Fig. 4C).
DISCUSSION
Disruption of normal gene expression in the adult epididymis is frequently found with exposure to exogenous compounds during the early postnatal period. Our previous researches have demonstrated aberrant expression of Cx isoforms in the initial segment and corpus epididymis of adult rat treated with EB or flutamide at 7 days of postnatal age. Data from the present study also clearly shows that exposure to steroidal and/or antisteroidal material at the early postnatal development age influences on the expression of some Cx isoforms in the caudal epididymis of adult rat.
The exogenous treatment of EB or flutamide in neonatal rat at 7 days of postnatal age results in different expressional patterns of Cx isoforms within the corpus and caudal epididymis. For example, in the corpus epididymis, gene expression of Cx26 was significantly decreased by a highdose (1.5 mg/kg BW) EB and a low-dose (500 mg/kg BW) and a high-dose (50 mg/kg BW) flutamide treatment (Lee, 2015), while there was no significant change on Cx26 in the caudal epididymis. These findings suggest that expression of Cx26 gene could be regulated by estrogenic and/or androgenic materials in segment-specific manner within the epididymis. Indeed, expression of forkhead box A2 (Faxa2), which is one of androgen receptor coregulatory and involves in suppression of activation of lipocalin 5 by androgen, is higher in the corpus and caudal epididymis than the caput epididymis (Yu et al., 2006). Moreover, differential expression of various miRNAs among the epididymal segments has been extensively examined by other researches (Chu et al., 2015; Nixon et al., 2015). Taken together, different expressional patterns of Cx26 gene at different epididymal regions by treatment of same exogenous materials would be reasonable to be considered. Moreover, it is speculated that expression of Cx26 in the corpus epididymis would not be under regulation of estrogenic and/or androgenic action. Detailed examination on expressional regulation of Cx26 gene in the epididymis is clearly suggested for future researches.
It is noteworthy that expression of Cx30.3, Cx31.1, Cx37, and Cx45 genes by the treatment of a low-dose (0.015 mg/kg BW) EB at 7 days of postnatal age is significantly decreased in the caudal epididymis, but either no change or significantly increased in the corpus epididymis of adult rat (Lee, 2015). In addition, expression of Cx32 gene is induced by the same treatment in both caudal and corpus epididymis (Lee, 2015). Moreover, the treatment of a low-dose EB at 7 days of postnatal age doesn’t influence on expression of Cx26, Cx31, Cx40, and Cx43 gene in bother caudal and corpus epididymis (Lee, 2015). Such ununiformed outcomes on expressional patterns of Cx isoforms obtained from the corpus and caudal epididymis by the treatment of a low-dose EB could not be simply explainable at this point. Differential amounts of androgen and estrogen receptors along the epididymal duct are detected in the rodent (Zhou et al., 2002). Thus, it is speculated that the existence of androgen receptor and/or estrogen receptor at different amount within two epididymal regions could contribute to differential expression of Cx isoforms in these epididymal segments. Also, differential expression of specific transcription factors and/or coregulators between the corpus and caudal epididymis (Chu et al., 2015; Nixon et al., 2015) could lead into segmental-specific expression of Cx isoforms in the epididymis.
Comparison of data extracted from the corpus and caudal epididymis by a high-dose of EB and a low-dose and a high-dose of flutamide provides quite interesting findings. In the most of cases, these treatments at 7 days of postnatal age result in significant decreases of gene expression of Cx isoforms in the adult corpus epididymis (Lee, 2015), but the opposite results are acquired with the adult caudal epididymis treated with same materials. The exceptions are Cx26, Cx30.3, and Cx45 in a high-dose EB treatment, Cx26, Cx30.3, Cx40, and Cx45 in a low-dose flutamide treatment, and Cx26, Cx37, Cx40, and Cx43 (Lee, 2015). Lydka et al. (2011) have demonstrated that the administration of flutamide in prenatal boar results in morphological and functional abnormalities in the epididymis of adult boar. Especially, this research shows expressional change of Cx43 in the caudal epididymis, but not in the corpus epididymis (Lydka et al., 2011). As seen in our previous (Lee, 2013 & 2015) and present researches, Cx43 is the most predominant form of Cx isoforms in the epididymis. Thus, it is important to notice the opposite effects of high-dose EB and low-dose flutamide treatments on expression of Cx43 in the corpus and caudal epididymis. At this point, it is not possible to provide a clear description to explain such segmental differential expression of Cx43 gene at the epididymis. The existence of different concentration of androgen and/or estrogen receptor along the epididymal duct could influence on differential expression of Cx43 gene among different epididymal segments. Detailed molecular biological examination should be conducted to resolve a complex of transcriptional regulation of Cx isoforms in the epididymis.
In conclusion, the present study, in addition with previous findings in the corpus epididymis (Lee, 2015), provides an information which exposure to exogenous estrogenic and/ or antiandrogenic compound during the early postnatal period would deliver aberrant expression of Cx isoforms in the corpus and caudal epididymis. Therefore, it is supposed that such abnormal expression of Cx isoforms induced by endocrine-disrupting compounds during the neonatal development could eventually lead into functional defect of the epididymis.