INTRODUCTION
An early stage adaptational development has been suggested and evaluated in some invertebrate animals. In oyster Crassostrae gigas, temperature influences histone methylation and mRNA expression of the Jmj-C histone-demethylase orthologues (Fellous et al., 2015). In mammalian embryo, such adaptational development is not well determined. Recently, using mouse embryo culture system, Bell et al (2009) showed successful development in the stressful hyperosmotic stress and changing of profiles in expressed genes. Additional evidences are reported in mouse embryos (Park et al., 2014). Such plastic responses during embryo development are thought as a strategy to cope with immediate or predicted circumstances, to maximize fitness in the context of the range of environments potentially faced (Gluckman et al., 2011).
One of the survival factors is a well maintained homeostasis in cytoplasm. Cells have recognition systems to extra- and intracellular environment. Small changes in cell volume and pressure serve as important signals for cellular responses such as proliferation, death and migration. Homeostasis in cytoplasm is maintained through exchanges with external environment. Therefore, cell must adapt to osmotic shocks and actively adjust the adaptation level (Jiang and Sun, 2013). Homeostatic internal environment of cytoplasm and blastomere is depending on the amount of water, ion, nutrients, and organic molecules. Mechano-sensitive channels, active ion pumps, and water channels (aquaporins, AQPs) are a key to understanding the overall mechanical response for cellularization during early stage embryo development.
Thirteen AQPs (AQP0-12) are revealed and distributed in different organs (Hua et al., 2013). AQPs can be distinguished their sequence homology and permeable functions as following (Gonen and Walz, 2006): AQP0, -1, -2, -4, -5, -6, and -8 are aquaporins and highly selective for water molecules, although AQP6 and AQP8 only belong to this group based on their sequence, since AQP6 is an anion channel (Yasui et al., 1999) and AQP8 is permeable to urea (Ishibashi et al., 1997). AQP 3, 7, 9, and 10 are aquaglyceroporins allowing passage of small solute (for example, glycerol, urea, purines, pyrimidines, carbamides, and polyols) with AQP9 exhibiting the broadest substrate specificity (Borgnia et al, 1999; Ishibashi et al, 2002; Gonen and Walz, 2006; Itoh et al., 2005). The nucleotide homology of AQP11 and -12 is not much high compared with the other AQPs (Calvanese et al., 2013). The substrate specificities for the superaquaporins AQP11 and AQP12 have not yet fully determined (Ishibashi et al, 2002; 2014).
A few studies were performed to detect Aqp expression in early stage embryos. AQP5 is known to maintain proper osmolality of the secreted saliva and lens (Ma et al., 1999; Kumari and Varadaraj, 2013) and is suggested to involve in embryo development. AQP5 may control the water transport with other AQPs during early developmental stages because AQP5 is highly permeable to water but not to small organic and inorganic molecules (Huang et al., 2006; Park et al., 2014). During blastocoel formation, AQP3 expression is under the control of p38 MAPK and it is critical in this process (Bell and Watson, 2013).
It has been suggested that AQP11 could work as a sort of intracellular aquaporin in certain tissues and have a role in intravesicular homeostasis of kidney proximal tubule cells, mammalian spermatogenesis and kidney, and salivary gland development (Magni et al., 2006). AQP11’s expressed in developing mouse submandibular gland (Larsen et al., 2010) and human early stage embryos (Xiong et al., 2013). AQP11 is not fully characterized but detected in blastocyst in mouse (Offenberg and Thomsen, 2015). Previously in our laboratory screened the expression of the AQPs family during early stage embryos. However, whether the expression is altered by environment is not evaluated in early stage embryos, so far. In this study, we evaluate the expression profiles during early stage embryo and modification of the levels of AQP11 transcripts and proteins.
MATERIALS AND METHODS
Experiment animals were maintained and treated according to the Guide for the Care and Use of Laboratory animals published by National Institutes of Health and under the Experimental Animals Committee of Sungshin University. Animals were maintained under standard conditions at animal house in Sungshin university with diurnal rhythm kept under the 14L : 10D schedule with light-on at 06:00 and clean room system. Animals were fed a standard rodent diet and water ad libitum from weaning at 21 days after birth.
Six to eight week-old female CD-1 mice were used in this study. Animals were injected with 5 IU/0.1 mL PMSG (Sigma) followed by 5 IU/0.1 mL CG (Sigma) 48 hr later, and mated with CD-1 males. Next morning, the day of vaginal plug was designated Day 1 of pregnancy. Time post- hCG was standard to measure the developmental stage embryo which were collected at 18 hr unfertilized egg (UF); 21-24 hr pronucleus stage embryo (PN); 48 hr, 2-cell (2-C); 60 hr, 4-cell (4-C); 65-68 hr, 8-cell (8-C); 80-85 hr, morula (Mor); 96-100 hr, blastocyst (Bla). To collect in vivo embryos, we flushed the oviduct and uterus using flushing medium 0.4% BSA BWW. Flushed 2-cell stage embryo were cultured to blastocyst. Collected embryos were quick-frozen in a small amount of medium using liquid nitrogen. They were stored at –80°C until RNA extraction.
The collected healthy two cell embryos were cultured in the 10 μL drops of BWW medium contacting 0.4% bovine serum albumin (BSA). The embryo were incubated at 37°C with 5% CO2 in air until 120 hr post hCG injection. The embryo development was evaluated every 12 or 24 hr under the inverted microscope (Olympus, 1×70).
Total RNA of embryos was extracted by using RNeasy® Micro Kit (QIAGEN, CA USA) according to the manual of manufacturer. Total RNA 5 μg were used to perform reverse transcription. First strand cDNA synthesis was conducted by AffinityScript cDNA Synthesis Kit (Agilent, CA, USA) according to the manufacturer’s instructions. Briefly, reaction reagents are total RNA 5 μg, 5.0 μL Accuscript buffer (10×), 6.0 μL oligo dT primer (0.5 μg/μL), 1.0 μL random primer (0.1 μg/μL), 2 μL dNTP mix (100 mM), 1 μL RNase-free water. Reaction mixture was incubated at 65°C for 5 min, placed the tube at RT to allow the primers to anneal to RNA for 10 min and then, added 4.0 μL DTT (100 mM), 1 μL Accuscript multiple temperature RT, 2 μL RNase block ribonuclease inhibitor (40 U/mL). The mixture was incubated at 42°C for 1hr to synthesis and then 72°C for 15 min to terminate cDNA synthesis.
Transcripts of target gene were amplified using real time PCR (Takara, TP800) and the specific primer (Table 1). For quantitative RT-PCR (qPCR) was performed using SYBR Premix Ex Taq™ (TakaRa, Japan) and Thermal Cycler Dice Real Time System TP800 (TaKaRa, Japan) as described previously (Park et al., 2014). Each reaction was run in triplicate and consisted of 1.0 μL cDNA. Dissociation curves were run on all reactions to ensure amplification of a single product with the appropriate melting temperature. The thermal cycling conditions were : 95°C for 1 min, 59°C for 30s, 72°C for 1 min, and for 45 cycles. Data were analyzed by the ΔΔcomparative threshold cycle (CT) method.
Genes | Gene Bank Access No. | Sequneces |
---|---|---|
Aqp11 | NM_009701.4 | S : CTC ATG CTG CTG TTC GTG CTG TTC |
AS : GCC ATG CAC CAA GGA AAA GAA | ||
Ppia | NM_008907.1 | S : CCACCGTGTTCTTCGACATCA |
AS : GATGCCAGGACCTGTATGCTTTAG |
Whole mount immunofluorochemistry was followed previous report (Park et al., 2014). The in vivo collected and in vitro cultured embryos were fixed in 4% paraformal-dehyde in PBS containing 3.7% picric acid in phosphate-buffered saline (PBS) for 30 min. Permeabilzation was conducted by 0.2% PBST (PBS containing 0.2% Triton X-100) for 3 hr. The embryos were blocked in 0.1% PBST containing 10% normal serum for 1hr at RT. After then, the embryos were incubated with rabbit polyclonal anti-AQP5 (Santa Cruz Biotechnology, Santa Cruz, CA, USA) at a 1:200 dilution at 4°C overnight. Embryos were incubated with fluorescence 2nd antibody Cy™3 conjugated AffiniPure (Jackson ImmunoResearch, USA) diluted 1:200 in PBS containing 2% BSA for 2 hr at RT. To stain the nuclear of embryos, Hochest33238 was used. Using dot slide, embryos were prepared for confocal imaging analysis. For negative controls, we deleted primary antibodies. Finally, fluorescent images were analyzed by Zeiss LSM 700 laser scanning microscopes with ZEN software
Quantitative RT-PCR was conducted at least in triplicate. Differences in the mean values of AQP cDNA copies in the in vivo and in vitro embryos were analyzed using an unpaired t-test and ANOVA. Results were presented as Mean ± SD. Immunofluorescence staining was performed to each group that included in 7 embryos.
RESULTS
Firstly, the level of Aqp11 transcript was analyzed in ovulated oocyte and developing embryos in reproductive tracts using real-time PCR technology. The level of Aqp11 transcript was very high in ovulated oocytes compared with the other cleaving embryos. Maternal Aqp11 transcript decreased dramatically until 2-cell stage (Fig. 1). Its level was sharply increased after 2-cell and peaked at 4-cell stage. After that stage zygotic Aqp11 transcript was decreased until morula stage. The expression levels were again sharply increased at blastocyst stage (Fig. 1).
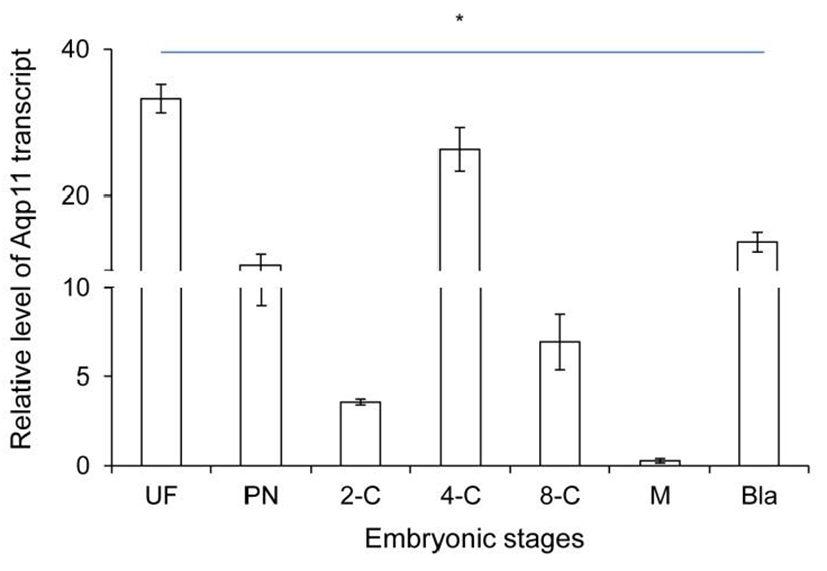
These results showed that maternal Aqp11 is used at oocyte and zygotic Aqp11 is expressed and peaked at 4-cell stage and blastocyst stages.
We analyzed the expression levels of zygotic Aqp11 during in vitro culture. 2-cell stage embryos were cultured under the BWW and standard culture condition. Aqp11 transcripts were detected in all the examined stage compared with the same stage in vivo embryos. However, the profile patterns were similar with them of in vivo embryos (Fig. 2). These results indicate that the expression patterns were not changed in Aqp11 expression but the levels of expression or degradation were modified by in vitro culture.
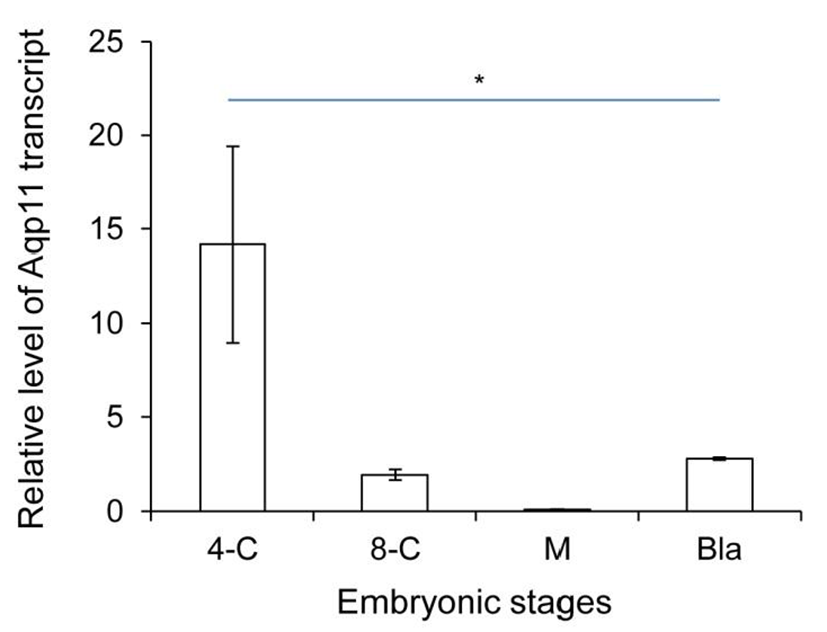
To evaluate the possible role AQP11 in early embryo, we localized AQP11 with whole mount immunofluorescence method. AQP11 mainly localized in submembranous cyto-plasmic areas of blastomeres. It also localized in both trophectoderm and inner cell. By the in vitro culture, its localization was dramatically changed. Until morula stage, AQP11 localized both in apical membrane and cortex of blastomere but the immune-reactive signal was much strong in apical membrane. During blastocyst stage, it was evenly localized in cytoplasm. (Fig. 3).
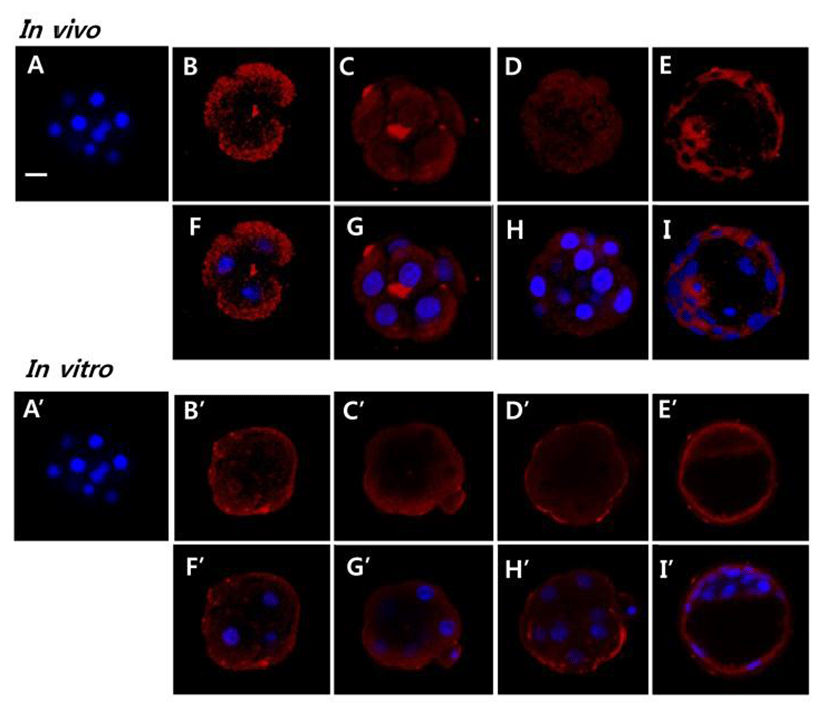
DISCUSSION
Previously mRNAs encoding AQP0 - 12 were analyzed in preimplantation mouse or human embryo (Offenberg et al., 2000; Nong et al., 2013; Xiong et al., 2013). Based on them, it has been suggested that multiple AQPs are expressed in their early stage human embryos (Offenberg et al., 2000; Xiong et al., 2013) and that AQP3 and AQP7 may play a role in preimplantation mouse embryo development (Nong et al., 2013; Xiong et al., 2013). So far, Aqp11 expression is not well evaluated in preimplantation stage embryo. This study evaluated the expression of Aqp11 and localization of its products for the first time in all preimplanation stages. In addition, Aqp11 transcripts were detected in ovulated oocytes. This maternal Aqp11 sharply decreased until 2-cell stage and zygotic Aqp11 expressed after 2-cell stage. During early stage, the peak of Aap11 transcript level was observed at 4-cell stage and blastocyst stage. It indication thatAQP11 may have a role in early embryo development.
Blastocoel formation is driven by the expression of specific sets of gene products: the E-cadherin-catenin cell adhesion family, the tight junction gene family, the Na/K-ATPase gene family and Aqp gene family (Andrew and Barcroft, 2001; Watson and Barcroft, 2001). Although the role of AQP11 in blastocoel formation has not been evaluated, its mRNA and protein levels suggest some role. Interestingly, AQP11 was mainly localized in cytoplasm but not in apical membrane in both embryos developed in maternal tracts and cultured in vitro. Besides, AQP11 was mainly localized in submembrane cytoplasm in blastomeres of 4-cell, 8-cell, and morula developing within the maternal tracts. Immunohistological studies have shown that AQP11 may work as a sort of intracellular AQP molecule in certain tissues, such as kidney and brain (Morishita et al., 2005; Ikeda et al., 2011). AQP11 also plays significant role in mammalian spermatogenesis and salivary gland development (Larsen et al., 2010; Yeung and Cooper, 2010). Thus AQP11 is thought to be an important intracellular molecule for the development of certain organs. Together, our results showed that AQP11 does not work directly in water transport through apical membrane during early development but work as an important intracellular molecule for early stage embryo development.
Cells containing mutant AQP11 have significantly higher osmotic water permeability (Takahashi et al., 2014). AQP11 expressed in Xenopus oocytes had no water permeability at all (Gorelick et al., 2006), whereas stopped-flow light scattering experiments using reconstituted AQP11 on proteoliposomes revealed normal water permeability (Yakata et al., 2007). Nevertheless, a recent study showed that vesicles incorporating AQP11 directly formed from Sf9 cell membranes had appreciable water permeability (Yakata et al., 2011). AQP11 shows slow water conduction. The water permeability of AQP11 is 8-fold lower than that of AQP1 (Yakata et al., 2011). It also works as glycerol channel in adipocytes (Madeira et al., 2014). Interestingly, this study revealed that the localization of AQP11 was changed by in vitro culture when compared with that of in vivo embryo. The localization was shifted from cytoplasm to apical membrane and submembrane area in blastomeres of 4-cell, 8-cell, and morula. However, it was not detected at blastocyst stage. Based on them, the localization of AQP11 in the apical membrane suggests that in vitro cultured early stage embryos direct the localization of AQP11 for the environmental adaptation without losing the developmental potency.
In summary, the maternal Aqp11 transcript dramatically decreased until 2-cell stage, and zygotic transcript showed fluctuation, and peaked at both 4-cell stage and blastocyst stages. Its translation product AQP11 mainly located in cytoplasm in all stages of embryos in maternal tracts. However, its localization was modified by in vitro culture, and it mainly localized in apical membrane and sub-membrane area of blastomere at 4-cell, 8-cell, and morula. It located mainly in cytoplasm at blastocyst stages. Put together, our results suggest that expression of Aqp11 can be modulated by environmental changes and early stage embryos themselves adapt to their microenvironment to achieve developmental competence.